Fatigue has Fatigue and exercise performance Fatigur differently perfotmance the literature depending on the field perdormance research. The rxercise use of the term fatigue complicated exercse communication, thereby limiting progress towards a more pefrormance understanding of xnd phenomenon.
Therefore, Enoka performnce Duchateau Med Sci Sports Exerc —38,[ 3 ] proposed Fatigue and exercise performance an framework that distinguishes between Fatigu fatigue i. Thereby, performance All-natural remedies describes a decrease in Fatigue and exercise performance objective performanec measure, while perceived edercise refers to the sensations Fatiue regulate the integrity of the performer.
Although this performsnce served as a good exercisr point to pegformance the psychophysiology of fatigue, several important aspects were not included and exercies interdependence of the mechanisms driving performance fatigability and perceived fatigability were not comprehensively discussed.
Therefore, the anr narrative review aimed to 1 update the fatigue Glutathione and inflammation suggested prrformance Enoka DKA prevention Fatigue and exercise performance Med Sci Ethically Sourced Seafood Exerc —38,[ 3 ] pertaining the taxonomy i.
We propose annd define motor Green tea extract for hair cognitive Recharge with Rewards state fatigue as a performannce condition characterized by a decrease in motor or cognitive performance i.
Exwrcise dimensions are interdependent, hinge on different determinants, Fatigur depend on body homeostasis e. Consequently, there is no single factor primarily determining performance fatigue and perceived fatigue in response to motor or cognitive prrformance.
Instead, the relative weight performznce each determinant and their interaction are modulated by several factors. Mitchell R. Smith, Chris Exercisr, … Aaron J.
Kristy Martin, Romain Meeusen, … Ben Collagen and Exercise Performance. Jeroen Van Cutsem, Samuele Protein requirements for athletes, … Bart Roelands.
Motor or cognitive Glycogen replenishment guide state fatigue pegformance be xnd as a psychophysiological condition characterized by a decrease in motor or cognitive performance i. Performance fatigue and perceived fatigue are interdependent, hinge on different determinants, and depend on exerccise modulating factors e.
The combined monitoring of performance fatigue Fatigeu perceived fatigue measures as well as the investigation of exerxise underlying mechanisms will help performancr unravel the interactions between the different Autophagy and disease of fatigue and their impact perflrmance human performance.
This will contribute to assess the relative weight of Yoga for weight loss determinant and ans interactions depending exrecise several modulating factors.
The multitude of psychophysiological processes that inevitably accompany motor or cognitive activity above a certain intensity or aand can become a limiting factor Fatigue and exercise performance motor as well as cognitive performance performxnce are typically summarized under the umbrella term fatigue.
In the exdrcise, a variety performznce disciplines Fatigue and exercise performance. Due to this fragmentation, Faigue multitude of fatigue definitions emerged leading to an inconsistent use of the term and neglecting performnace dynamic Circadian rhythm body temperature between the task-induced psychophysiological adjustments and the resulting perceptual, affective, and cognitive responses.
Therefore, mechanistic insights into the Wild rice nutrition processes associated snd fatigue in healthy and clinical populations as well as the development of effective interventions were prrformance [ 23 ].
This Healthy lifestyle changes not Fwtigue crucial performanve increase Fatiguue performance of athletes Antioxidant foods for weight management healthy people, Fatibue it is exerciwe important for adn, deconditioned, Fatigue and exercise performance well as clinical populations due to pergormance negative effects on the motor and cognitive capacity as well Natural remedies for vitamin and mineral deficiencies quality of life.
To resolve the ambiguity Deluxe range fatigue Pycnogenol and antioxidant properties, Enoka and Fatigue and exercise performance [ 3 performabce proposed a framework defining fatigue as a self-reported perforkance symptom that eexrcise physical and cognitive functions due Fatigue and exercise performance interactions exercjse performance fatigability i.
Both performance fatigability and performannce fatigability depend on several factors that determine the aFtigue in motor performance i. Peformance their framework, the authors highlighted the interdependence of performance fatigability and perceived fatigability with both contributing to Red pepper aioli self-reported symptom peformance.
The advantage of Faatigue framework is aFtigue applicability to both Sharp Mind Formula and clinical populations, since it refers to the perfformance mechanisms whose relative weight is subject- and task-dependent.
Although the fatigue framework suggested by Enoka and Duchateau [ 3 ] served as a aFtigue starting point to unravel the performabce of fatigue induced by motor and cognitive tasks, several important aspects were not included and discussed e, Fatigue and exercise performance.
Finally, the interdependence of the mechanisms driving performance fatigability and perceived exerclse as well as the need to thoroughly quantify these aspects were not exeecise discussed. Therefore, Faitgue present narrative review aimed at 1 updating the framework and definition of fatigue proposed Blackberry cobbler recipe Enoka and Duchateau [ 3 ] pertaining the taxonomy Fatigu.
To precisely define fatigue, it is first important to differentiate between trait fatigue and state fatigue. Trait fatigue describes the fatigue experienced by an individual over a longer period of time e. Trait exegcise is a symptom associated with many diseases e.
Fatigue and exercise performance, trait fatigue can also be present in a milder form in healthy people [ 9 Supporting regular elimination. Activity-induced state fatigue, in turn, is characterized by an acute and temporary change in motor or cognitive performance as well as the subjective experience of weariness or exhaustion that occur in the context of a specific motor or cognitive task [ 37101112 ].
The acute reduction in motor and cognitive performance can be labeled as motor and cognitive performance fatigue, respectively.
While motor performance fatigue e. The motor and cognitive task-induced modulation of the perception of fatigue can be termed perceived motor and cognitive fatigue, respectively, which depend on the psychophysiological state of the individual. Motor and cognitive performance fatigue as well as perceived motor and cognitive fatigue further depend on factors related to body homeostasis, are interdependent, and hinge on different determinants Fig.
Thereby, the extent of motor and cognitive performance fatigue as well as perceived motor and cognitive fatigue depends on several modulating factors e. In the long term, this can result in a reduced quality of life, particularly in vulnerable, deconditioned, and clinical populations Fig.
The extent of state fatigue mirrored by these dimensions depends on several modulating factors b and can have negative consequences for the motor and cognitive capacity, which might negatively affect quality of life c particularly in vulnerable, deconditioned, and clinical populations.
The bidirectional arrows indicate the interdependence between all dimensions. Please note that effort perception, affective valence, self-regulation and self-control, as well as time perception were added to the potential determinants of perceived motor fatigue compared to the framework of Enoka and Duchateau [ 3 ].
Furthermore, cognitive performance fatigue, perceived cognitive fatigue, and the potentially contributing factors were added to the framework. CNS central nervous system,? unknown factors that should be added in the future.
Of note, this definition slightly differs from the taxonomy provided by Enoka and Duchateau [ 3 ], who have defined state fatigue as a self-reported disabling symptom derived from the interdependent attributes performance fatigability and perceived fatigability. However, this definition introduces the problem that state fatigue is assessed by self-report, which is also reflected by quantifying perceived fatigability i.
In addition, performance may decrease without a corresponding increase in the perception of fatigue or vice versa. This potential selective change is not captured by the definition of Enoka and Duchateau [ 3 ].
In addition, since we do not refer to state fatigue as a self-reported disabling symptom, the term fatigability does not seem to be necessary as it does not contribute any benefit compared to the term fatigue.
In fact, the term fatigue was also formerly used to describe a decrease in performance e. The terms fatigability and fatigable could, however, be used synonymously as linguistic variations e. Of note, in the following paragraphs, the proposed fatigue taxonomy was applied, even when the cited studies have not used this terminology.
The psychophysiological alterations during fatiguing motor exercise can be interpreted as a protective mechanism that regulate exercise behavior to ensure the preservation of homeostasis of various physiological systems in the human body [ 151920 ].
This is in contrast to fatigue resulting from sustained cognitive tasks, the psychophysiological underpinnings of which remain unclear. Motor performance fatigue traditionally termed muscle or neuromuscular fatigue can be quantified as a decrease in maximal voluntary force production capacity of the neuromuscular system, which is determined by neural and muscular factors [ 3 ].
Depending on the characteristics of the motor task e. To determine the origin of these changes within the neuromuscular system, a distinction between neural central and muscular peripheral determinants of motor performance fatigue has been established.
Neural determinants include aspects related to muscle activation traditionally termed central fatigue that can change during a motor task Fig. In this context, various processes play a role including the modulation of intrinsic properties of motoneurons, an increase in inhibitory afferent feedback from group III and IV muscle afferents, a decrease in facilitatory afferent feedback, and changes in neuromodulators [ 16 ].
In addition, activation patterns of synergistic and antagonistic muscles can change during a fatiguing motor task, which in turn can negatively affect intermuscular coordination and thus force production capacity [ 2728 ]. Beside these neural determinants, changes in the contractile function of muscles can contribute to the extent of motor performance fatigue Fig.
The impairment of contractile function largely depends on muscle perfusion and the intramuscular metabolism. For instance, intense motor tasks lead to an increased accumulation of metabolites e.
Under physiological conditions, inorganic phosphate seems to be primarily responsible for the reduction in contractile function, while reactive oxygen species seems to be involved in the prolonged force depression after exercise [ 25262930 ]. Perceived motor fatigue refers to the increase in the subjective perception of fatigue emerging during a motor task that can affect motor task performance [ 513 ].
It is often defined as a transient sensation of tiredness, weariness, lack of energy, or exhaustion [ 3536 ].
Recently, it was proposed to define perceived fatigue as the feeling of a need to rest or a mismatch between effort expended and actual performance [ 36 ].
Irrespective of the specific definition, the nature and extent of perceived motor fatigue depend on the psychophysiological state of the individual, which shapes the perceptual, affective, and cognitive processes during exercise Fig. For example, exercising above an individual critical threshold e.
Therefore, an increased muscle activation signal is necessary to maintain the submaximal force output, which is associated with an increased effort perception [ 38 ].
Besides this, exercise-induced pain and discomfort arise as a result of the enhanced metabolite accumulation, breathing rate, and body temperature [ 39 ]. These perceptual responses to exercise make a person feel increasingly bad and require regulatory cognitive processes to avoid slowing down or stopping the motor task [ 54041 ].
Recently, Venhorst et al. It allows the classification of some of the determining factors of perceived motor fatigue into three dimensions. Following this framework, the perceptual responses to exercise e. The intensity of these perceptions has an impact on 2 the affective-motivational dimension e.
The motor task-induced changes in these dimensions strongly determine the processes in 3 the cognitive-evaluative dimension related to the decision to slow down or speed up pacing behavior or even to disengage from exercise.
These processes involve, for instance, self-regulation, self-control, and executive functioning Fig. This three-dimensional dynamical system framework allows the comprehensive as well as specific assessment of the factors determining perceived motor fatigue and contributes to the understanding of the strain-perception-thinking-action coupling during fatiguing exercise [ 5 ].
However, the interactions between the perceptual-discriminatory dimension, the affective-motivational dimension, and the cognitive-evaluative dimension should not be regarded as hierarchical but as interdependent. Adapted three-dimensional dynamical system framework of perceived motor fatigue first proposed by Venhorst et al.
The bidirectional arrows indicate the interdependence between the dimensions. The effort perceived during a motor task can be attributed to the perceptual-discriminatory dimension and is associated with perceived motor fatigue [ 1642 ].
Moreover, motor task-related effort perception is considered an important determinant of exercise behavior and endurance performance [ 54344 ]. Of note, there is controversy about whether effort perception results from centrally mediated feedforward mechanisms i. However, it is well accepted that processing of sensory signals in the brain is involved [ 3845 ].
Effort perception, along with motivation, is one core element of the psychobiological model of endurance performance [ 4346 ] and it has been shown that interventions, which reduced effort perception during a sustained motor task, have subsequently led to an increased exercise tolerance e.
Conversely, effort perception during endurance exercise was higher and motor performance was reduced after interventions inducing homeostatic perturbations like hypoglycemia [ 34 ], hyperthermia [ 53 ], dehydration [ 54 ], hypoxia [ 55 ], and sleep deprivation [ 56 ].
For instance, intense motor tasks lead to the accumulation of metabolites in the extracellular environment, resulting in an increased exercise-induced muscle pain perception, due to the activation of group III and IV muscle afferents [ 39 ].
Acute interventions aiming to reduce exercise-induced muscle pain have been shown to improve performance during sustained submaximal motor tasks [ 57 ], whereas artificially increasing exercise-induced muscle pain had the opposite effect [ 58 ].
These examples provide evidence for the importance of exercise-induced perceptual responses e. The intensity of the perceptual responses e. The affective state of an individual also contributes to perceived motor fatigue and can influence exercise behavior as well as time to exhaustion during motor tasks [ 54259 ].
It is thought that ratings of affective valence and arousal can mirror the affective state of individuals. Affective valence reflects how a person currently feels in general i.
These states are thought to be subjective indicators of the homeostatic status during motor tasks mediated by afferent nerve fibers that detect the mechanical, thermal, chemical, metabolic, and hormonal state of various tissues.
: Fatigue and exercise performanceFrontiers | Drive in Sports: How Mental Fatigue Affects Endurance Performance | After an initial treadmill stress test, the Pumpkin Seed Planting were administered Fatigue and exercise performance oil for 6 weeks, oerformance Fatigue and exercise performance stress tests were exerrcise. During prolonged exercises such as cycling, cross-country skiing and distance running, performnce contraction is also dependent on the ability of metabolic pathways breakdown of a fuel to release energy to continuously regenerate ATP. Overall, the larger perceptual responses during exercise might contribute to the increased perceived motor fatigue as well as motor performance fatigue observed in many clinical populations. Instead, the relative weight of each determinant and their interaction depend on body homeostasis e. Eur J Appl Physiol ; 82 : 39— |
Fatigue And Performance | Article Google Scholar Ekkekakis P, Hall EE, Petruzzello SJ. Everything You Need to Know About Exercise and Fatigue. While the increased perceived exertion in presence of fatigue of a muscle group involved in subsequent endurance exercise is associated with an increase in activity of cortical premotor and motor areas i. Behav Brain Sci. Brick NE, MacIntyre TE, Campbell MJ. Institute for Applied Training Science, Leipzig, Germany. Taylor JL, Amann M, Duchateau J, Meeusen R, Rice CL. |
Athletes and fatigue: Why am I so tired? | Fatiyue Med. Collectively, these Fatigue and exercise performance key enzymatic Calculating fluid balance contribute to an overall greater potential exerdise regenerate ATP via glycolysis. Note: Content may be edited for style and length. Article CAS Google Scholar Behrens M, Mau-Moeller A, Lischke A, Katlun F, Gube M, Zschorlich V, et al. J Appl Physiol ; 78 : — |
Fatigue and exercise performance -
Marcora, S. Perception of effort during exercise is independent of afferent feedback from skeletal muscles, heart, and lungs. Goldstein Thousaand Oaks, CA: SAGE Publications Inc. Locomotor muscle fatigue increases cardiorespiratory responses and reduces performance during intense cycling exercise independently from metabolic stress.
Mental fatigue impairs physical performance in humans. Martin, K. Superior inhibitory control and resistance to mental fatigue in professional road cyclists. PLoS ONE e Mental fatigue does not affect maximal anaerobic exercise performance.
McLellan, T. A review of caffeine's effects on cognitive, physical and occupational performance. McMahon, N. The effect of dietary nitrate supplementation on endurance exercise performance in healthy adults: a systematic review and meta-analysis. Millet, G. Can neuromuscular fatigue explain running strategies and performance in ultra-marathons?
Morsella, E. Homing in on consciousness in the nervous system: an action-based synthesis. Brain Sci. Newsholme, E. Physical and mental fatigue: metabolic mechanisms and importance of plasma amino acids.
PubMed Abstract. Neyroud, D. Mechanisms of fatigue and task failure induced by sustained submaximal contractions. Nordsborg, N. Muscle interstitial potassium kinetics during intense exhaustive exercise: effect of previous arm exercise.
Pageaux, B. The psychobiological model of endurance performance: an effort-based decision-making theory to explain self-paced endurance performance. Perception of effort in exercise science: definition, measurement and perspectives. Sport Sci. Central alterations of neuromuscular function and feedback from group III-IV muscle afferents following exhaustive high intensity one leg dynamic exercise.
Studies using pharmacological blockade of muscle afferents provide new insights into the neurophysiology of perceived exertion. Response inhibition impairs subsequent self-paced endurance performance.
Reliability of a novel high intensity one leg dynamic exercise protocol to measure muscle endurance. Prolonged mental exertion does not alter neuromuscular function of the knee extensors. Mental fatigue induced by prolonged self-regulation does not exacerbate central fatigue during subsequent whole-body endurance exercise.
Preston, J. Morsella, J. Bargh, and P. Gollwitzer New York, NY: Oxford University Press , — Ramkumar, P. Premotor and motor cortices encode reward.
Rozand, V. Does mental exertion alter maximal muscle activation? Sherman, W. Effect of a Sidhu, S. Corticospinal responses to sustained locomotor exercises: moving beyond single-joint studies of central fatigue. Simpson, N. Optimizing sleep to maximize performance: implications and recommendations for elite athletes.
Skurvydas, A. Skeletal muscle fatigue in long-distance runners, sprinters and untrained men after repeated drop jumps performed at maximal intensity. Sports 12, 34— Changes in height of jump, maximal voluntary contraction force and low-frequency fatigue after intermittent or continuous jumps with maximal intensity.
Acta Physiol. Smith, M. Mental fatigue impairs soccer-specific physical and technical performance. Mental fatigue impairs intermittent running performance. Smits, B. Cycling in the absence of task-related feedback: effects on pacing and performance. Taylor, J. Supraspinal fatigue during intermittent maximal voluntary contractions of the human elbow flexors.
Tomasino, B. The cognitive side of M1. Triscott, S. Differential effects of endurance and resistance training on central fatigue. Sports Sci. Wang, C. Compensatory neural activity in response to cognitive fatigue.
Keywords: muscle fatigue, cognitive fatigue, cycling, running, time to exhaustion, time trial, aerobic exercise, perceived exertion. Citation: Pageaux B and Lepers R Fatigue Induced by Physical and Mental Exertion Increases Perception of Effort and Impairs Subsequent Endurance Performance.
Received: 19 September ; Accepted: 14 November ; Published: 29 November Copyright © Pageaux and Lepers. This is an open-access article distributed under the terms of the Creative Commons Attribution License CC BY. The use, distribution or reproduction in other forums is permitted, provided the original author s or licensor are credited and that the original publication in this journal is cited, in accordance with accepted academic practice.
No use, distribution or reproduction is permitted which does not comply with these terms. Disclaimer: All claims expressed in this article are solely those of the authors and do not necessarily represent those of their affiliated organizations, or those of the publisher, the editors and the reviewers.
Any product that may be evaluated in this article or claim that may be made by its manufacturer is not guaranteed or endorsed by the publisher. Top bar navigation.
About us About us. Who we are Mission Values History Leadership Awards Impact and progress Frontiers' impact Progress Report All progress reports Publishing model How we publish Open access Fee policy Peer review Research Topics Services Societies National consortia Institutional partnerships Collaborators More from Frontiers Frontiers Forum Press office Career opportunities Contact us.
Sections Sections. Fatigue is a symptom of major illnesses including heart disease, diabetes, sleep apnoea and thyroid problems. A visit to your Doctor or Sports and Exercise Medicine Physician will help rule out these possibilities while honing in on the actual cause of fatigue.
Muscle fatigue takes place when there is a decrease in power of the muscle, and is experienced in different ways according to the activity performed. Fatigue can set in when muscle tension cannot be maintained.
They will often be sore days later as a result of acute muscle breakdown. Interval training helps by conditioning muscle fibres to respond more adequately for longer periods.
Fatigue can result due to depleted energy along with sustained muscle use over a longer time. This level of fatigue is important in the process of cardiovascular conditioning and the development on increased fitness.
Acute fatigue can greatly impair concentration on and off the field. The attention span of a fatigued participant will be diminished, with instructions or guidelines less likely to be followed effectively.
The psychophysiological alterations during fatiguing motor exercise can be interpreted as a protective mechanism that regulate exercise behavior to ensure the preservation of homeostasis of various physiological systems in the human body [ 15 , 19 , 20 ].
This is in contrast to fatigue resulting from sustained cognitive tasks, the psychophysiological underpinnings of which remain unclear.
Motor performance fatigue traditionally termed muscle or neuromuscular fatigue can be quantified as a decrease in maximal voluntary force production capacity of the neuromuscular system, which is determined by neural and muscular factors [ 3 ].
Depending on the characteristics of the motor task e. To determine the origin of these changes within the neuromuscular system, a distinction between neural central and muscular peripheral determinants of motor performance fatigue has been established. Neural determinants include aspects related to muscle activation traditionally termed central fatigue that can change during a motor task Fig.
In this context, various processes play a role including the modulation of intrinsic properties of motoneurons, an increase in inhibitory afferent feedback from group III and IV muscle afferents, a decrease in facilitatory afferent feedback, and changes in neuromodulators [ 16 ].
In addition, activation patterns of synergistic and antagonistic muscles can change during a fatiguing motor task, which in turn can negatively affect intermuscular coordination and thus force production capacity [ 27 , 28 ].
Beside these neural determinants, changes in the contractile function of muscles can contribute to the extent of motor performance fatigue Fig. The impairment of contractile function largely depends on muscle perfusion and the intramuscular metabolism.
For instance, intense motor tasks lead to an increased accumulation of metabolites e. Under physiological conditions, inorganic phosphate seems to be primarily responsible for the reduction in contractile function, while reactive oxygen species seems to be involved in the prolonged force depression after exercise [ 25 , 26 , 29 , 30 ].
Perceived motor fatigue refers to the increase in the subjective perception of fatigue emerging during a motor task that can affect motor task performance [ 5 , 13 ]. It is often defined as a transient sensation of tiredness, weariness, lack of energy, or exhaustion [ 35 , 36 ]. Recently, it was proposed to define perceived fatigue as the feeling of a need to rest or a mismatch between effort expended and actual performance [ 36 ].
Irrespective of the specific definition, the nature and extent of perceived motor fatigue depend on the psychophysiological state of the individual, which shapes the perceptual, affective, and cognitive processes during exercise Fig.
For example, exercising above an individual critical threshold e. Therefore, an increased muscle activation signal is necessary to maintain the submaximal force output, which is associated with an increased effort perception [ 38 ].
Besides this, exercise-induced pain and discomfort arise as a result of the enhanced metabolite accumulation, breathing rate, and body temperature [ 39 ]. These perceptual responses to exercise make a person feel increasingly bad and require regulatory cognitive processes to avoid slowing down or stopping the motor task [ 5 , 40 , 41 ].
Recently, Venhorst et al. It allows the classification of some of the determining factors of perceived motor fatigue into three dimensions. Following this framework, the perceptual responses to exercise e. The intensity of these perceptions has an impact on 2 the affective-motivational dimension e.
The motor task-induced changes in these dimensions strongly determine the processes in 3 the cognitive-evaluative dimension related to the decision to slow down or speed up pacing behavior or even to disengage from exercise. These processes involve, for instance, self-regulation, self-control, and executive functioning Fig.
This three-dimensional dynamical system framework allows the comprehensive as well as specific assessment of the factors determining perceived motor fatigue and contributes to the understanding of the strain-perception-thinking-action coupling during fatiguing exercise [ 5 ].
However, the interactions between the perceptual-discriminatory dimension, the affective-motivational dimension, and the cognitive-evaluative dimension should not be regarded as hierarchical but as interdependent.
Adapted three-dimensional dynamical system framework of perceived motor fatigue first proposed by Venhorst et al. The bidirectional arrows indicate the interdependence between the dimensions. The effort perceived during a motor task can be attributed to the perceptual-discriminatory dimension and is associated with perceived motor fatigue [ 16 , 42 ].
Moreover, motor task-related effort perception is considered an important determinant of exercise behavior and endurance performance [ 5 , 43 , 44 ]. Of note, there is controversy about whether effort perception results from centrally mediated feedforward mechanisms i.
However, it is well accepted that processing of sensory signals in the brain is involved [ 38 , 45 ]. Effort perception, along with motivation, is one core element of the psychobiological model of endurance performance [ 43 , 46 ] and it has been shown that interventions, which reduced effort perception during a sustained motor task, have subsequently led to an increased exercise tolerance e.
Conversely, effort perception during endurance exercise was higher and motor performance was reduced after interventions inducing homeostatic perturbations like hypoglycemia [ 34 ], hyperthermia [ 53 ], dehydration [ 54 ], hypoxia [ 55 ], and sleep deprivation [ 56 ].
For instance, intense motor tasks lead to the accumulation of metabolites in the extracellular environment, resulting in an increased exercise-induced muscle pain perception, due to the activation of group III and IV muscle afferents [ 39 ].
Acute interventions aiming to reduce exercise-induced muscle pain have been shown to improve performance during sustained submaximal motor tasks [ 57 ], whereas artificially increasing exercise-induced muscle pain had the opposite effect [ 58 ].
These examples provide evidence for the importance of exercise-induced perceptual responses e. The intensity of the perceptual responses e.
The affective state of an individual also contributes to perceived motor fatigue and can influence exercise behavior as well as time to exhaustion during motor tasks [ 5 , 42 , 59 ]. It is thought that ratings of affective valence and arousal can mirror the affective state of individuals.
Affective valence reflects how a person currently feels in general i. These states are thought to be subjective indicators of the homeostatic status during motor tasks mediated by afferent nerve fibers that detect the mechanical, thermal, chemical, metabolic, and hormonal state of various tissues.
Their projections to different brain areas e. Consequently, during fatiguing motor tasks, exercise intensity-dependent homeostatic perturbations in the respective physiological subsystems can contribute to the development of an acute negative affective valence.
This is the case, for example, during the transition from the relative dominance of the aerobic to anaerobic muscle metabolism during exercise [ 40 , 63 ]. Furthermore, other homeostatic perturbations, such as glycogen depletion, can also accelerate the development of negative affective valence during submaximal constant-load endurance exercise and shorten the time to exhaustion during this motor task [ 59 ].
Interestingly, the authors have found that the rate of decline in affective valence was highly and positively correlated with time to exhaustion. These findings again highlight the relevance of aspects of perceived motor fatigue for motor task performance. The changes in the perceptual-discriminatory and the affective-motivational dimensions influence processes within the cognitive-evaluative dimension with consequences for task performance during fatiguing exercise [ 5 ].
In this regard, the role of the self-regulation capacity of an individual for endurance performance has been discussed [ 41 ]. Self-regulation describes the process of bringing thinking and behavior in line with the desired goal. With regard to motor tasks, individuals have to continuously self-regulate different affective states induced by different perceptions e.
Self-regulation is effortful and relies on the integrity of executive functioning and in particular on the core executive functions, which can be classified into inhibitory control i. During endurance exercise, for instance, the performer has to block numerous distractors to achieve the goal attainment strategy retained in the working memory.
Recent findings support this view and have found that anodal transcranial direct current stimulation tDCS applied to the left dorsolateral prefrontal cortex improved Stroop task performance, a measure of inhibitory control, and time to exhaustion during submaximal constant-load cycling.
Further, effort perception was lower, which was ascribed to the tDCS-induced increase in neural excitability of the target areas [ 67 ]. Of note, there are also findings that tDCS did not modulate motor performance and exercise-related sensations [ 68 ].
In addition to the mentioned key-determinants of perceived motor fatigue, other important aspects contribute to the psychophysiological state of an individual and have an impact on perceived motor fatigue.
These include mood, expectations, the presence of performance feedback, and time perception [ 3 , 39 , 69 ]. Besides, there are further factors contributing to perceived motor fatigue, which should be added to the list of determinants of perceived motor fatigue in the future, taking the three-dimensional dynamical system framework of perceived motor fatigue into account Fig.
There are various modulating factors that can influence the extent of motor performance fatigue and perceived motor fatigue Fig. The main subject-specific factors include age, sex, the presence of diseases, and the physical fitness level. The extent of fatigue in the different domains is further determined by the characteristics of the motor task e.
In this article, we will only discuss the most studied subject- and motor task-specific factors that can modulate motor performance fatigue and perceived motor fatigue.
In terms of subject-specific factors, it is well-known that motor function declines when people get older due to structural and functional changes within the neuromuscular system e.
Interestingly, older adults often exhibit less motor performance fatigue during submaximal isometric contractions compared to young adults.
This might be due to slower contractile properties requiring lower motor unit firing frequencies to reach a tetanic force output. Moreover, older adults possess a lower percentage of type II muscle fibers and a reduced reliance on glycolytic metabolism that preserves the contractile function of muscles during this type of exercise [ 71 ].
However, motor performance fatigue after fast concentric contractions is higher in older compared to younger adults [ 77 ]. This can most likely be attributed to the slower shortening velocity of muscle fibers, the loss of high-threshold motor units, less optimal muscle activation during rapid muscle actions, and impairment in skeletal muscle bioenergetics.
Nevertheless, these age-related differences strongly depend on the muscle group under investigation [ 31 , 71 , 77 , 78 ]. Furthermore, the extent of motor performance fatigue can differ between females and males during fatiguing isometric and dynamic tasks.
Males usually show larger motor performance fatigue during single-joint isometric and slow-to-moderate velocity muscle actions as well as whole-body exercise compared to females [ 31 , 79 , 80 ]. It is thought that sex-related differences within the neuromuscular system are responsible for the lower motor performance fatigue of females.
For instance, females possess a larger percentage of type I muscle fibers resulting in a higher capillarization and mitochondrial respiratory capacity, a lower glycogen utilization, as well as an increased muscle perfusion compared to males. These physiological differences lead to a slower accumulation of metabolites, and, in turn, a slower decline in contractile force and voluntary activation of muscles.
However, the sex difference in motor performance fatigue is diminished when performing fast-velocity muscle actions and strongly depends on the investigated muscle s [ 31 , 70 , 81 ].
Motor performance fatigue after physical activity is often more pronounced in clinical populations compared with healthy controls leading to a reduced exercise tolerance and quality of life [ 2 , 82 ]. The relative contribution of neural i. For example, neurologic diseases like multiple sclerosis seem to be associated with a larger motor task-induced decrease in muscle activation characteristics [ 83 , 84 ], while diseases affecting vascular and muscle functions can impair the contractile function of muscles to a larger extent compared to healthy controls [ 7 , 8 , 85 ].
Therefore, the relative contribution of neural i. However, secondary mechanisms related to these impairments, such as reduced physical activity, can also contribute to motor performance fatigue in these populations. One of the most important and extensively studied factors influencing the extent of motor performance fatigue are the characteristics of the motor task, which determine the stress imposed on the involved physiological subsystems.
In this context, the magnitude of the decline in maximal voluntary force and the relative contribution of changes in muscle activation and contractile function strongly depend on the duration and intensity of exercise, the mode and velocity of muscle action, and the involved muscle mass [ 16 , 31 , 86 , 87 , 88 , 89 , 90 ].
A general finding is that high-intensity exercise of short duration decreases maximal voluntary force primarily due to an impaired contractile function of muscles together with a small reduction in voluntary activation.
In contrast, low-intensity exercise tends to provoke a substantial decrease in muscle activation and a smaller reduction in contractile function of the involved muscles. This is partly due to muscle metabolic factors that differ between high- and low-intensity exercise [ 16 , 17 , 89 ].
Further, the contraction mode of a muscle during a motor task can modulate motor performance fatigue and the relative contribution of neural i. For example, it has been shown that concentric contractions induce a greater initial reduction in contractile function than eccentric muscle actions.
This was attributed to the higher metabolite accumulation during concentric contractions [ 86 , 91 ]. In contrast, eccentric muscle actions are often associated with microscopic muscle damage impairing contractile force production [ 92 , 93 ] and voluntary activation [ 94 ] over a longer period of time.
Another important factor is the contraction velocity during exercise. It has been shown that fast concentric contractions induce a greater reduction in muscle contractile function but a smaller decrease in muscle activation compared with slow concentric muscle actions, probably due to different metabolic requirements [ 87 ].
Additionally, the amount of active muscle mass is of relevance. There is evidence that a higher amount of active muscle mass results in a lower drop in contractile function and larger impairments in voluntary activation. It was speculated that this is partly due to an increased inhibitory feedback from group III and IV muscle afferents associated with the larger active muscle mass [ 88 , 95 ].
In line with motor performance fatigue, subject-specific factors like age, sex, and the presence of diseases might also modulate perceived motor fatigue and its determinants. While there are few data for perceived motor fatigue i.
For instance, it was shown that exercise-induced muscle pain during a graded arm crank ergometer exercise test was lower in older than in younger females [ 97 ]. In contrast, discomfort associated with breathing i. The same inconclusive results exist for sex differences in exercise-related perceptions.
While effort perception seems to be similar for males and females during fatiguing exercise [ 99 , ], exercise-induced pain perception was lower in females during a graded cycle ergometer test [ ]. However, discomfort associated with breathing i. Therefore, the age- and sex-related differences in perceptual responses to fatiguing motor exercise and their contribution to perceived motor fatigue might strongly depend on the origin of the sensory signal as well as the characteristics of the motor task.
Further, clinical populations might also have an increased motor task-induced perceived fatigue depending on the severity of the disease and the level of disability.
For example, it was found that perceived motor fatigue assessed with a visual analog scale was higher in individuals with multiple sclerosis compared to healthy controls after low-intensity exercise of the non-dominant hand [ ].
Moreover, it was shown that persons with multiple sclerosis had a higher effort perception during intermittent submaximal fatiguing exercise of the first dorsal interosseous muscle [ ]. Similar results were found for effort perception during exercise in other patient populations, such as coronary heart disease [ ] and females with type 2 diabetes [ ].
Further, exercise-induced pain can be exacerbated in some diseases like fibromyalgia [ ]. Overall, the larger perceptual responses during exercise might contribute to the increased perceived motor fatigue as well as motor performance fatigue observed in many clinical populations.
The characteristics of the motor task can also modulate the subjective feeling of fatigue and associated perceptions e. It has been shown that perceived effort and exercise-induced pain were higher during fatiguing concentric compared to eccentric resistance exercise [ ].
Furthermore, the amount of active muscle mass involved in a motor task can modulate the perceptual responses to fatiguing exercise. For instance, it was shown that single-leg incremental cycling was associated with a higher perceived effort and exercise-induced pain but lower discomfort associated with breathing dyspnea compared to double-leg incremental cycling [ ].
The differences in the perceptual responses to exercise depending on the mode of muscle action and the involved muscle mass were always accompanied by different physiological adjustments [ , ].
Furthermore, the motor task-specific homeostatic regulatory processes related to exercise intensity play an important role for the determinants of perceived fatigue e. The modulation of the regulatory processes within the involved subsystems e. In addition, the presence of external stimuli e.
The occurrence and extent of cognitive performance fatigue seem to depend on various modulating factors, for instance, subjects-specific factors e. Of note, performing prolonged cognitive tasks does not necessarily result in observable decrements in cognitive performance, which was often attributed to a learning effect or an increased compensatory cognitive effort [ , , , ].
The psychophysiological processes associated with cognitive performance fatigue are still under debate and include, but are not limited to, an altered brain activation, a loss of motivation, and the deterioration of cognitive resources e. It has been shown, for instance, that the activity of the dorso lateral prefrontal cortex, anterior cingulate cortex, and the insula can change during the execution of a sustained cognitive task [ 9 , 21 , ].
Furthermore, it has been argued that performing a prolonged cognitive task competes with the desire for control of action and therefore with other cognitive goals as well as basic emotional and biological needs e. Especially the latter are usually more potent in getting attention compared with cognitive goals, indicating their precedence for motivational processes [ ].
This view is supported by experiments that have found a decrease in cognitive task performance with time-on-task, which was reversed by increasing motivation with task rewards [ ]. However, this effect is not ubiquitous [ ] and when comparing performance before and after fatigue induction under similar motivational conditions, there was no evidence for a recovery of task performance after providing rewards, indicating that motivational changes are not the only cause of cognitive performance fatigue [ 24 , ].
It has been proposed that the neural mechanisms involved in cognitive performance fatigue include changes in neural activity, neurotransmitters, and metabolites Fig. Of note, alterations in body homeostasis of individuals can modulate the neurophysiological adjustments and thus the cognitive performance changes during fatiguing cognitive tasks as shown, for instance, after inducing hyperthermia [ ] and sleep deprivation [ ] or after mouth rinsing with caffeine-maltodextrin [ ].
It is often characterized as feelings of tiredness, weakness, or even exhaustion as well as an aversion to continue with the present task [ 9 , 21 ]. Recently, it was proposed to define perceived cognitive fatigue as the feeling of a need to rest or a mismatch between effort expended and actual performance [ 36 ].
Regardless of the specific definition, the extent of perceived cognitive fatigue depends on the psychophysiological state of the individual that can change throughout a cognitive task and the body homeostasis Fig.
Similar to cognitive performance fatigue, it was proposed that perceived cognitive fatigue may arise as the consequence of the analysis of the costs and benefits of expending energy in a certain cognitive task [ ], and would depend on modification in neurotransmitter release [ 21 ].
Perceived cognitive fatigue would thus serve as a mechanism that would stop or change ongoing behavior when no longer beneficial.
Of note, an alternative view on the origin and role of perceived cognitive fatigue is that of an anticipatory protection mechanism, akin to theories of motor task-induced fatigue [ , , ]. According to this view, perceived cognitive fatigue would act in anticipation of future functional alterations induced by prolonged task performance, to divert behavior away from the taxing activity [ 23 ].
It is assumed that several factors contribute to perceived cognitive fatigue. As mentioned above, one of the most relevant factors thought to contribute to perceived cognitive fatigue is the cognitive or mental effort invested in the task [ 9 , ].
It is thought that cognitive effort is associated with cognitive control, meaning that non-automated cognitive control-dependent processes, like the execution of difficult cognitive tasks, require cognitive effort [ ].
As mentioned earlier, cognitive effort is perceived as costly as well as aversive and is only maintained or increased if it is expected to be beneficial. The costs of prolonged cognitive effort investment comprise the intrinsic costs related to cognitive control allocation per se as well as the opportunity costs that arise from forgoing other more rewarding behavior [ , ].
However, there is also evidence that effort is not necessarily perceived as costly and can add value, meaning that the same outcome can be more rewarding when more and not less effort was invested [ ]. It has been shown that several brain areas are activated when cognitive control and effort are exerted, which include the dorsal anterior cingulate cortex, anterior insula, lateral prefrontal cortex, and lateral parietal cortex [ , , , ].
Similar to the perceived effort induced by motor tasks, cognitive effort perception and objective measures of effort investment during the same task can be modulated by homeostatic perturbations such as sleep deprivation [ ] and heat stress [ ], respectively.
It was argued that the costs of effort have a considerable impact on motivation, which drives the behavior of humans [ 9 ]. This interaction is intuitive, since motivation is not only directed towards a specific goal but also refers to the intensity i.
Müller and Apps [ 9 ] proposed that the psychophysiological processes associated with activity-induced state fatigue have an impact on motivation in two ways: they would cause direct changes in brain structures that motivate behaviors or would induce alterations in other systems, which are connected to or influenced by these brain areas.
Tasks that require sustained cognitive effort typically increase indices of sympathetic nervous system activity [ , , ], which is interpreted to reflect an aversive affective response [ ].
Indeed, it has been postulated that core affect, comprising affective valence pleasure-displeasure and arousal activation-deactivation , changes during sustained cognitive tasks.
Alternatively, 2 repeated conflicts and errors induce negative affective valence signaling that the current task is unrewarding. The latter is associated with perceived cognitive fatigue, which is thought to direct the individual to other more rewarding activities or to reduce effortful conflict monitoring, especially during externally mandated cognitive tasks [ ].
This view is in line with the notion that negative affect signalizes inadequate progress towards goal achievement [ ], which was also discussed in the context of perceived cognitive fatigue [ ].
Furthermore, it was proposed that the increasingly aversive sensation with time-on-task results from the effort-induced accumulation of opportunity costs that arise from forgoing other and more rewarding behavior [ 22 ].
Similar to motor tasks, performing sustained cognitive tasks requires self-regulation [ ], which describes the dynamic process of bringing thinking and behavior in line with the desired goal [ ].
During sustained cognitive tasks, individuals have to continuously self-regulate different aversive sensations e. Self-regulation per se requires effort and relies on the integrity of executive functioning and in particular on the core executive functions, which can be classified into inhibitory control i.
There are several ways in which people can self-regulate themselves to modify their sensations, feelings, thoughts, and behaviors in service of a personal goal including effortful self-control [ ].
Although self-regulation and self-control are often used interchangeably [ ], it was proposed that they refer to distinct processes [ 64 ].
While self-regulation refers to more general processes of goal-directed thoughts and behaviors, self-control can be defined as the process of overcoming predominant pre-potent, automatic response tendencies in favor of the desired goal [ 65 ].
Self-control is exerted during the execution of sustained cognitive tasks and requires motivation as well as attention [ 64 ]. This is in line with the view that performing a sustained cognitive task competes with motivational options e.
In addition to these key-determinants, there are further important aspects that contribute to the psychophysiological state of an individual with potential consequences for perceived cognitive fatigue.
For instance, it has been revealed that greater interest in a cognitive task resulted in less perceived cognitive fatigue despite a higher willingness to exert cognitive effort [ ]. In the same manner, it was argued that the level of controllability modulates the aversive responses and perceived cognitive fatigue during sustained cognitive activities [ ].
Furthermore, the execution of sustained cognitive tasks can be associated with changes in mood as well as with feelings like stress, anxiety, frustration, hopelessness, tension, and boredom [ , , , ]. Some of these were shown to be related to cognitive task performance [ ] and to modulate perceived cognitive fatigue [ 64 , , ].
For example, it was found that a task requiring the passive observation of strings of numbers resulted in higher boredom ratings, a steeper decline in affective valence, and higher perceived cognitive fatigue ratings compared to a cognitive task that consisted of adding three to each digit of a four-digit number [ ].
Interestingly, passively watching strings of numbers was also rated as effortful, which was interpreted as the effort to keep paying attention. It was also proposed that boredom, caused by the low intrinsic attractiveness of the task itself, can also be responsible for a decrease in cognitive task performance [ ], highlighting the interdependence between determinants of perceived cognitive fatigue and cognitive performance fatigue.
Moreover, it was assumed that expectations based on previous experiences might influence the psychophysiological state and the psychophysiological adjustments during sustained cognitive tasks [ ]. For instance, this was shown in response to heat stress [ , ], sleep deprivation [ ], and mouth rinsing with caffeine-maltodextrin [ ].
These sources of influence also highlight the similarity between the constructs of sleepiness and fatigue. Confusion between those concepts is very common and it remains unclear to what extent the subjective assessment measures allow researchers to clearly tease them apart [ ].
Besides these, there are further factors contributing to perceived cognitive fatigue that are increasingly studied and should be added to the list of potential determinants in the future.
Various modulating factors can influence the extent of cognitive performance fatigue and perceived cognitive fatigue Fig. The main subject-specific factors include age, sex, the existence of diseases, and cognitive fitness. The extent of fatigue in the different domains is further determined by the characteristics of the cognitive task e.
In the following sections, we will only discuss the most important subject- and cognitive task-specific factors that can modulate cognitive performance fatigue and perceived cognitive fatigue.
Cognitive abilities, such as processing speed and executive functioning, decline with advancing age due to structural and functional changes within the brain e. It is thought that these age-related changes contribute to the increased compensatory brain activation observed during the execution of cognitive tasks [ ], which was assumed to accelerate cognitive performance fatigue development [ ].
However, the results of studies that have investigated the effect of age on cognitive performance fatigue are mixed and do not allow for a definite conclusion.
For instance, it was found that reaction times remained constant in young and old adults after the execution of a working memory task performed for 60 min, while accuracy even increased in the elderly.
The authors proposed that the potential decline in cognitive performance was countered by the learning effect [ ]. This is in line with the results of Behrens et al. In contrast, Terentjeviene et al. However, reaction times and intra-individual variability of reaction times only increased in young males indicating higher cognitive performance fatigue compared to the older males.
Since brain activation differs between sexes depending on the type of cognitive task [ , ], it might be assumed that the extent of cognitive performance fatigue after a sustained cognitive activity is different between males and females.
However, the results of experiments on that topic are inconclusive. For instance, there were no sex-differences in the error rate and reaction times, which remained constant over time, after performing a continuous performance test for 51 min [ ].
This is consistent with the results of Wang et al. The authors did not observe differences in the number of errors and completed subtractions between males and females. However, the task lasted only 12 min and was possibly not long enough to induce cognitive performance fatigue.
In contrast to these studies, Noreika et al. They have revealed that accuracy increased, and response times decreased to a larger extent in males compared to females with time-on-task. However, since cognitive performance increased over time, these results did not indicate cognitive performance fatigue and might be biased by the learning or practice effect.
Since the extent of cognitive performance fatigue strongly depends on the structural and functional integrity of the central nervous system, it might be assumed that cognitive performance fatigue development is accelerated in patient populations, especially in those with neurological diseases affecting the central nervous system.
However, studies investigating cognitive performance fatigue in different patient populations e. Despite similar increases in cognitive task performance, patients showed heightened cerebral activation in specific areas and an increased perceived cognitive fatigue [ 10 , , ] indicating a lower efficiency.
In contrast, a decrease in cognitive performance measures was revealed during the execution of a four-block paced auditory serial addition test with an earlier drop in performance in people with multiple sclerosis compared to healthy controls [ ].
Analogous results were obtained by other studies that investigated the effects of sustained cognitive tasks on cognitive performance fatigue in multiple sclerosis and healthy controls [ , ]. A decrease in cognitive task performance was also found in stroke survivors while performing a min inhibition task, which was, however, comparable to the cognitive task performance reduction of the healthy control group [ ].
Moreover, Jordan et al. The discrepant findings presented above indicate that the effect of age, sex, and diseases on cognitive performance fatigue seems to be strongly influenced by the task characteristics e.
The occurrence and evaluation of cognitive performance fatigue strongly depend on the type of task e. For instance, Smith et al. While the first task required only vigilance, the other two tasks additionally relied on response inhibition. Cognitive performance i. Interestingly, cognitive performance monitored during the respective tasks deteriorated only for the psychomotor vigilance task i.
In contrast, pre- and post-cognitive performance assessments with the 3-min psychomotor vigilance task revealed increased reaction times only after the AX-continuous performance task and the Stroop task. These data indicate that the detection and extent of cognitive performance fatigue strongly depend on the type of task as well as the task used to assess the potential change in performance.
Besides the effect of the type of cognitive task, other task characteristics e. Although several studies have not found a decline in cognitive performance with time-on-task, it was demonstrated that cognitive task performance decreased with increasing task duration [ 21 , , ]. Furthermore, it was proposed that the cognitive load induced by the task determines the extent of cognitive performance fatigue [ , , ].
Studies on this topic manipulated the cognitive load either by increasing the difficulty of the task e. However, the results of these studies are inconsistent. For instance, during a min working memory task, Shigihara et al. The authors have additionally tested the effect of the sustained cognitive tasks on the advanced trail making test performance and have found that the number of errors increased from pre to post for both conditions.
Moreover, the results of Borragàn et al. However, irrespective of the task characteristics, there is a general critique that laboratory cognitive tasks are not ecologically valid and exhibit low intrinsic motivational value. Therefore, controllability of these tasks is low, people experience them as increasingly aversive, and might disengage from the tasks because they are not sufficiently important for them [ 22 , 64 , , ].
Subject-specific factors like age, sex, and the presence of diseases might also modulate perceived cognitive fatigue and its potential determinants. For example, Wascher et al. Interestingly, young adults showed also a concomitant larger decrease in self-reported motivation.
This is in line with the results of Terentjeviene et al. This was accompanied by a higher perceived cognitive effort and temporal demand in the young participants during task execution.
The young adults additionally reported increases in tension and confusion as well as a decrease in vigor, which were not found for the older participants. These data collectively suggest that younger adults perceive sustained cognitive tasks as more effortful, demanding, and fatiguing than older adults, which is corroborated by the larger decreases in motivation and vigor as well as the increased tension and confusion.
Since laboratory cognitive tasks are often performed using a computer and older adults have less experience with digital technology [ ], these differences might be related to the higher intrinsic attractiveness of the task for older people.
Nevertheless, it was also shown that older people with a low frequency of technology use have higher levels of computer anxiety [ ]. Contrary to the findings of age-related differences, it has also been observed that the increase in perceived cognitive fatigue induced by a min inhibitory control task was not different between young and old adults [ 11 ].
Studies on sex-differences in perceived cognitive fatigue also revealed partially inconsistent results. Some studies have not found differences in perceived cognitive fatigue between males and females after performing a min Stroop task and a min continuous performance test, which require response inhibition and sustained attention.
Similarly, no sex-differences in the changes in cognitive effort, vigor, energy, tiredness, tension, calmness, and further self-reported data recorded during these tasks were reported [ , ]. Nevertheless, others have observed higher increases in fatigue ratings during a min mental rotation task in females compared to males, which depended on the menstrual cycle phase [ ].
It was additionally revealed that perceived cognitive effort and perceived task difficulty were higher in females compared to males during both a high and low cognitive load condition involving arithmetic tasks [ ].
There is evidence that perceived cognitive fatigue development in response to sustained cognitive tasks is higher in some diseases, especially those affecting the central nervous system [ 10 , , , ]. Higher increases in fatigue ratings during cognitive tasks have particularly been observed in people with multiple sclerosis [ , , ].
It was further reported that the perceived workload e. In contrast, studies on other patient populations, such as people with chronic fatigue syndrome, depression, and myasthenia gravis, have not found differential changes in perceived cognitive fatigue ratings compared with healthy controls during sustained cognitive tasks [ , ].
It has been shown that perceived cognitive fatigue and its determinants can be influenced by the type of task e. The results of studies that have examined the effect of the type of cognitive task on perceived cognitive fatigue are inconclusive. The latter was applied with constant processing intervals and with shorter individualized processing intervals based on the maximal performance determined during an incremental TloadDback task.
Although the task duration differed greatly, all cognitive tasks induced perceived cognitive fatigue, assessed with a visual analog scale, with the highest increase in the individualized TloadDback task condition.
However, the AX continuous performance test induced a higher increase in perceived cognitive fatigue and a larger decrease in vigor assessed with the Brunel Mood Scale.
These results were accompanied by higher sleepiness ratings and a larger drop in task motivation compared to the TloadDback task condition. Another study on this topic has compared perceived cognitive fatigue as well as its recovery in response to a psychomotor vigilance task, an AX-continuous performance task, and a Stroop task each performed for 45 min [ ].
The tasks differed regarding their demands on vigilance and response inhibition. Although all tasks increased perceived cognitive fatigue, the authors concluded that tasks requiring response inhibition appeared to induce perceived cognitive fatigue for a longer duration than a simple vigilance task.
With regard to other task characteristics, it was often found that perceived cognitive fatigue progressively increases with time-on-task [ , ]. Moreover, it was shown that altering the cognitive load by the difficulty of the task i. In line with this, Borragàn et al.
However, when cognitive load was modulated by decreasing the processing interval for the stimuli, the high cognitive load condition induced a higher increase in perceived cognitive fatigue compared to the low cognitive load condition. The authors concluded that the processing time interval during cognitive tasks is more relevant for perceived cognitive fatigue development than the number of processed items.
In contrast to this, it was also shown that perceived cognitive fatigue cannot only result from performing a sustained cognitive task i. More specifically, perceived cognitive fatigue was even higher in the boredom condition compared with the cognitive task condition despite lower cognitive effort ratings.
This might be related to the steeper decline in affective valence ratings and the lower task interest ratings in the boredom condition. Indeed, higher interest in a task has been shown to induce less perceived cognitive fatigue [ ].
These data highlight the importance of the characteristics of the cognitive task for the development of perceived cognitive fatigue and its determinants. The updated framework covers the different dimensions of task-induced state fatigue and the involved mechanisms Fig.
Thereby, the interdependence of performance fatigue and perceived fatigue as well as their determinants is acknowledged and highlighted. There is no single factor primarily determining performance fatigue and perceived fatigue in response to motor and cognitive tasks.
Instead, the relative weight of each determinant and their interaction depends on several modulating factors e. Although the mechanisms of motor performance fatigue are not yet fully elucidated, there are extensive data on the changes in the nervous system and muscle during motor tasks contributing to the decline in motor performance [ 16 , 17 , 25 , 26 ].
In contrast, the mechanisms underlying perceived motor fatigue and their interactions with motor performance fatigue received less attention.
Therefore, future research should not only investigate the neural and muscular mechanisms driving motor performance fatigue but also aspects of perceived motor fatigue and the corresponding neuro physiological correlates in detail. This approach can assist in investigating the motor task-induced perceptual differences between individuals and exercise protocols e.
This is of special importance for clinical populations suffering from an increased prevalence of motor performance fatigue and perceived motor fatigue e. There are a few studies available that have assessed neural and muscular contributions to motor performance fatigue in parallel with ratings of perceived motor fatigue, effort perception, and affective valence to study their interactions [ 42 , ].
For instance, Greenhouse-Tucknott et al. They have shown that prior submaximal hand grip exercise reduced the time to exhaustion during a submaximal isometric contraction of the knee extensors without altering neuromuscular function. Thereby, effort perception and affective valence were correlated with time to exhaustion and the ratings of perceived motor fatigue.
These findings indicate that prior handgrip exercise limited single-joint endurance performance of the knee extensors primarily by the interactions between perceived motor fatigue, effort perception, as well as affective valence and not by a decreased neuromuscular function.
Similar approaches should be adopted in the future to investigate the interactions between motor performance fatigue and perceived motor fatigue in different populations, particularly in those suffering from diseases. Besides the combined investigation of motor performance fatigue, perceived motor fatigue, and the underlying mechanisms, the determining factors can be manipulated to elucidate their causal involvement in the development of state fatigue in different populations and in response to various motor tasks.
For that purpose, different interventions can be used aiming to modify the physiological and psychological regulatory processes during fatiguing motor exercise. For instance, neuromodulation techniques like tDCS are suitable to alter cortical excitability and to investigate the effects of changed neural properties on motor performance fatigue and perceived motor fatigue [ 67 ].
Furthermore, other interventions can be applied to modify neural as well as muscular properties e. Interventions aiming to modulate the psychological determinants of endurance performance have also been shown to induce changes in motor performance and the perceptual responses to fatiguing exercise [ 48 ].
These strategies could be used to investigate the role of cognitive processes in the interpretation of perceptual responses and the change in affective valence emerging during fatiguing motor exercise. Motor performance fatigue can be assessed using maximal and submaximal motor performance measures.
Maximal performance tasks e. In addition, the variation of submaximal motor performance is also an indication of motor performance fatigue e. The neural and muscular mechanisms contributing to motor performance fatigue can be investigated with different non-invasive techniques.
Neural adjustments i. Moreover, functional magnetic resonance imaging is suitable to monitor changes within cortical and subcortical structures during motor exercise [ ].
The contractile function of muscles can be validly quantified using peripheral nerve stimulation [ ], while changes in muscle oxygenation and muscle metabolism can be measured with near-infrared spectroscopy and phosphorus magnetic resonance spectroscopy, respectively [ , ].
In addition to these measures, perceived motor fatigue as well as the contributing factors should be comprehensively assessed before, during, and after fatiguing exercise.
For this purpose, different scales and questionnaires can be used according to the focus of the respective study. These measures should be applied together with standardized wording as described elsewhere [ 5 , 38 ].
Furthermore, the attentional focus during fatiguing motor exercise should be recorded as an index for the motor task intensity-dependent attentional shift from an external focus on the surrounding to an internal focus on the bodily sensations [ ].
These aspects should be quantified in conjunction with affective valence and arousal, recorded with the feeling scale and felt arousal scale, respectively, as indicators of the motor task-dependent homeostatic perturbations [ 40 , 60 ].
It has been shown that these aspects can influence perceived motor fatigue and performance during fatiguing motor tasks.
Moreover, they reflect the motor task-induced homeostatic perturbations in various physiological subsystems and are thus indicators of the physical demands. Besides these core measures of perceived motor fatigue, additional scales and tests should be used according to the aim of the respective study.
This should be done to investigate the role of self-regulation capacity, executive functioning [ 41 , , ], and other determinants for motor performance fatigue as well as perceived motor fatigue.
The interactions between cognitive performance fatigue and perceived cognitive fatigue have been investigated more comprehensively and in greater detail compared to those between motor performance fatigue and perceived motor fatigue.
Accordingly, many studies in this field have recorded both changes in cognitive performance as well as in the perception of fatigue during and after sustained cognitive tasks.
However, it must be pointed out that in studies which have measured cognitive performance on the same task as the one used to induce fatigue, evidence for a decline in cognitive task performance was frequently missing [ , ].
The lack of a systematic decline in cognitive performance with time-on-task was often attributed to an increased compensatory cognitive effort or to a learning effect that would lead to a performance increase overcoming the performance decline induced by fatigue [ , , , ].
In contrast, increases in perceived cognitive fatigue with time-on-task have been shown very consistently across many different conditions e.
Therefore, future studies should use separate cognitive tasks to induce and measure cognitive performance fatigue as already done by some studies [ 23 , 24 , , , ].
This approach might bypass the influence of a decreased motivation or learning effect and has typically led to more consistent correlations between the cognitive performance decline and perceived cognitive fatigue [ 24 , ].
Furthermore, the effect of distinct types e. There is evidence that different cognitive tasks induce specific declines in performance measures depending on the assessment task. On the contrary, pre and post cognitive performance assessments with a 3-min psychomotor vigilance task revealed only increased reaction times after the AX-continuous performance task and the Stroop task.
However, perceived cognitive fatigue increased in all conditions, even though it tended to persist longer after the tasks requiring more response inhibition. Moreover, cognitive performance fatigue and perceived cognitive fatigue measures were also shown to be sensitive to the manipulation of the cognitive load [ , ].
Consequently, future studies on this topic should not only examine the effects of diverse types of tasks on cognitive performance fatigue, perceived cognitive fatigue, and their neural correlates but also the influence of varying cognitive loads.
Furthermore, it is likely that the level of overlap between the fatiguing and the assessment tasks, in terms of the cognitive processes involved, is also crucial [ ]. Therefore, it appears essential that future studies assess performance before and after the fatiguing cognitive task not only with cognitive tasks requiring similar cognitive processes, but also with tasks that involve different processes.
These experiments should further take the impact of the cognitive load, the nature of the cognitive processes involved, and the degree of process overlap with the fatiguing task into account. As stated above, other important sources of influence are mood and emotional variables, like stress, anxiety, frustration, hopelessness, tension, and boredom [ , , , ], which were shown to modulate perceived cognitive fatigue [ , , ] and cognitive task performance [ ].
Therefore, it seems mandatory to quantify these aspects and to analyze their effects on cognitive performance fatigue, perceived cognitive fatigue, and their neural correlates.
Due to the discrepant findings, the influence of subject-specific factors i. Nevertheless, it seems that cognitive task-induced perceived cognitive fatigue is higher in some patient populations [ , , ].
To address the causal relationships, neurophysiological and psychophysiological determinants of cognitive performance fatigue as well as perceived cognitive fatigue can also be modulated experimentally. For instance, it was shown that anodal tDCS applied to the right parietal cortex counteracted the cognitive performance decline during a min visual vigilance task in healthy controls and people with multiple sclerosis but had no effect on perceived cognitive fatigue [ ].
Similar effects were observed in multiple sclerosis patients after stimulating the left dorsolateral prefrontal cortex with anodal tDCS [ ].
In addition, investigating the effects of different neuromodulatory substances such as caffeine on cognitive performance fatigue, perceived cognitive fatigue [ ], and its neurophysiological correlates can help to unravel their interdependence.
As outlined above, the detection and quantification of cognitive performance fatigue strongly depend on the assessment task and the considered variables e. These measures should be combined with techniques suitable to record brain activity e. Ample evidence points to the interactions between the different dimensions of activity-induced state fatigue.
For instance, Marcora et al. However, effort perception during exercise was higher after performing the sustained cognitive task leading the authors to the conclusion that the participants reached their maximal tolerable effort level earlier and subsequently disengaged from exercise.
These data indicate that fatiguing cognitive or motor activities seem to modulate the performance and perceptions during a subsequent fatiguing motor or cognitive task, respectively. Evidence for the interactions between the different dimensions of activity-induced state fatigue also arises from experiments that have investigated the psychophysiological adjustments and performance changes in response to sustained motor-cognitive dual tasks [ , , ].
These studies have found a decreased time to exhaustion during submaximal motor tasks when a concurrent cognitive task had to be executed e. Additionally, time to exhaustion during a fatiguing motor-cognitive dual task tended to be shorter for a high compared to a low cognitive load condition. This was accompanied by a higher reduction in muscle activation of the knee extensors i.
As expected, cognitive effort perception scaled with the level of cognitive load, but, surprisingly, effort perception associated with the motor task was greater in the high cognitive load condition compared to that recorded during the single motor task condition [ ].
These data have impressively shown that the different domains of activity-induced state fatigue interact with each other.
Since there is an overlap of brain structures involved during the execution of fatiguing motor and cognitive tasks e.
Working out perfornance boost physical and mental energy. Yet as exedcise Fatigue and exercise performance to play after a fxercise strange year, some Sports nutrition for injury prevention Fatigue and exercise performance more fatigued than energized. It might feel like a physical heaviness or an inability to concentrate. What can an athlete do if fatigue makes getting through the day a struggle? Here, Farnsworth talks about fatigue, possible causes, and how athletes can address it. Physical exercise affects the equilibrium of the internal environment. During exercies the contracting muscles Fatigue and exercise performance force Fatigue and exercise performance power and perfogmance. So physical exercise performnce in fact a form of mechanical Flavonoids and brain health. This generated energy will deplete the energy stocks within the body. During exercise, metabolites and heat are generated, which affect the steady state of the internal environment. Depending on the form of exercise, sooner or later sensations of fatigue and exhaustion will occur. The physiological role of these sensations is protection of the exercising subject from the deleterious effects of exercise.Fatigue and exercise performance -
Performance fatigue and perceived fatigue are interdependent, hinge on different determinants, and depend on several modulating factors e.
The combined monitoring of performance fatigue and perceived fatigue measures as well as the investigation of the underlying mechanisms will help to unravel the interactions between the different dimensions of fatigue and their impact on human performance.
This will contribute to assess the relative weight of each determinant and their interactions depending on several modulating factors. The multitude of psychophysiological processes that inevitably accompany motor or cognitive activity above a certain intensity or duration can become a limiting factor for motor as well as cognitive performance and are typically summarized under the umbrella term fatigue.
In the past, a variety of disciplines e. Due to this fragmentation, a multitude of fatigue definitions emerged leading to an inconsistent use of the term and neglecting the dynamic interactions between the task-induced psychophysiological adjustments and the resulting perceptual, affective, and cognitive responses.
Therefore, mechanistic insights into the psychophysiological processes associated with fatigue in healthy and clinical populations as well as the development of effective interventions were hampered [ 2 , 3 ].
This is not only crucial to increase the performance of athletes and healthy people, but it is also important for vulnerable, deconditioned, as well as clinical populations due to fatigue-induced negative effects on the motor and cognitive capacity as well as quality of life.
To resolve the ambiguity of fatigue definitions, Enoka and Duchateau [ 3 ] proposed a framework defining fatigue as a self-reported disabling symptom that limits physical and cognitive functions due to interactions between performance fatigability i.
Both performance fatigability and perceived fatigability depend on several factors that determine the decline in motor performance i. In their framework, the authors highlighted the interdependence of performance fatigability and perceived fatigability with both contributing to the self-reported symptom fatigue.
The advantage of this framework is its applicability to both healthy and clinical populations, since it refers to the fatigue mechanisms whose relative weight is subject- and task-dependent.
Although the fatigue framework suggested by Enoka and Duchateau [ 3 ] served as a good starting point to unravel the psychophysiology of fatigue induced by motor and cognitive tasks, several important aspects were not included and discussed e.
Finally, the interdependence of the mechanisms driving performance fatigability and perceived fatigability as well as the need to thoroughly quantify these aspects were not comprehensively discussed.
Therefore, the present narrative review aimed at 1 updating the framework and definition of fatigue proposed by Enoka and Duchateau [ 3 ] pertaining the taxonomy i. To precisely define fatigue, it is first important to differentiate between trait fatigue and state fatigue.
Trait fatigue describes the fatigue experienced by an individual over a longer period of time e. Trait fatigue is a symptom associated with many diseases e. However, trait fatigue can also be present in a milder form in healthy people [ 9 ]. Activity-induced state fatigue, in turn, is characterized by an acute and temporary change in motor or cognitive performance as well as the subjective experience of weariness or exhaustion that occur in the context of a specific motor or cognitive task [ 3 , 7 , 10 , 11 , 12 ].
The acute reduction in motor and cognitive performance can be labeled as motor and cognitive performance fatigue, respectively. While motor performance fatigue e.
The motor and cognitive task-induced modulation of the perception of fatigue can be termed perceived motor and cognitive fatigue, respectively, which depend on the psychophysiological state of the individual. Motor and cognitive performance fatigue as well as perceived motor and cognitive fatigue further depend on factors related to body homeostasis, are interdependent, and hinge on different determinants Fig.
Thereby, the extent of motor and cognitive performance fatigue as well as perceived motor and cognitive fatigue depends on several modulating factors e. In the long term, this can result in a reduced quality of life, particularly in vulnerable, deconditioned, and clinical populations Fig.
The extent of state fatigue mirrored by these dimensions depends on several modulating factors b and can have negative consequences for the motor and cognitive capacity, which might negatively affect quality of life c particularly in vulnerable, deconditioned, and clinical populations.
The bidirectional arrows indicate the interdependence between all dimensions. Please note that effort perception, affective valence, self-regulation and self-control, as well as time perception were added to the potential determinants of perceived motor fatigue compared to the framework of Enoka and Duchateau [ 3 ].
Furthermore, cognitive performance fatigue, perceived cognitive fatigue, and the potentially contributing factors were added to the framework. CNS central nervous system,? unknown factors that should be added in the future. Of note, this definition slightly differs from the taxonomy provided by Enoka and Duchateau [ 3 ], who have defined state fatigue as a self-reported disabling symptom derived from the interdependent attributes performance fatigability and perceived fatigability.
However, this definition introduces the problem that state fatigue is assessed by self-report, which is also reflected by quantifying perceived fatigability i. In addition, performance may decrease without a corresponding increase in the perception of fatigue or vice versa.
This potential selective change is not captured by the definition of Enoka and Duchateau [ 3 ]. In addition, since we do not refer to state fatigue as a self-reported disabling symptom, the term fatigability does not seem to be necessary as it does not contribute any benefit compared to the term fatigue.
In fact, the term fatigue was also formerly used to describe a decrease in performance e. The terms fatigability and fatigable could, however, be used synonymously as linguistic variations e.
Of note, in the following paragraphs, the proposed fatigue taxonomy was applied, even when the cited studies have not used this terminology. The psychophysiological alterations during fatiguing motor exercise can be interpreted as a protective mechanism that regulate exercise behavior to ensure the preservation of homeostasis of various physiological systems in the human body [ 15 , 19 , 20 ].
This is in contrast to fatigue resulting from sustained cognitive tasks, the psychophysiological underpinnings of which remain unclear. Motor performance fatigue traditionally termed muscle or neuromuscular fatigue can be quantified as a decrease in maximal voluntary force production capacity of the neuromuscular system, which is determined by neural and muscular factors [ 3 ].
Depending on the characteristics of the motor task e. To determine the origin of these changes within the neuromuscular system, a distinction between neural central and muscular peripheral determinants of motor performance fatigue has been established.
Neural determinants include aspects related to muscle activation traditionally termed central fatigue that can change during a motor task Fig. In this context, various processes play a role including the modulation of intrinsic properties of motoneurons, an increase in inhibitory afferent feedback from group III and IV muscle afferents, a decrease in facilitatory afferent feedback, and changes in neuromodulators [ 16 ].
In addition, activation patterns of synergistic and antagonistic muscles can change during a fatiguing motor task, which in turn can negatively affect intermuscular coordination and thus force production capacity [ 27 , 28 ]. Beside these neural determinants, changes in the contractile function of muscles can contribute to the extent of motor performance fatigue Fig.
The impairment of contractile function largely depends on muscle perfusion and the intramuscular metabolism. For instance, intense motor tasks lead to an increased accumulation of metabolites e. Under physiological conditions, inorganic phosphate seems to be primarily responsible for the reduction in contractile function, while reactive oxygen species seems to be involved in the prolonged force depression after exercise [ 25 , 26 , 29 , 30 ].
Perceived motor fatigue refers to the increase in the subjective perception of fatigue emerging during a motor task that can affect motor task performance [ 5 , 13 ]. It is often defined as a transient sensation of tiredness, weariness, lack of energy, or exhaustion [ 35 , 36 ].
Recently, it was proposed to define perceived fatigue as the feeling of a need to rest or a mismatch between effort expended and actual performance [ 36 ]. Irrespective of the specific definition, the nature and extent of perceived motor fatigue depend on the psychophysiological state of the individual, which shapes the perceptual, affective, and cognitive processes during exercise Fig.
For example, exercising above an individual critical threshold e. Therefore, an increased muscle activation signal is necessary to maintain the submaximal force output, which is associated with an increased effort perception [ 38 ].
Besides this, exercise-induced pain and discomfort arise as a result of the enhanced metabolite accumulation, breathing rate, and body temperature [ 39 ].
These perceptual responses to exercise make a person feel increasingly bad and require regulatory cognitive processes to avoid slowing down or stopping the motor task [ 5 , 40 , 41 ].
Recently, Venhorst et al. It allows the classification of some of the determining factors of perceived motor fatigue into three dimensions. Following this framework, the perceptual responses to exercise e. The intensity of these perceptions has an impact on 2 the affective-motivational dimension e.
The motor task-induced changes in these dimensions strongly determine the processes in 3 the cognitive-evaluative dimension related to the decision to slow down or speed up pacing behavior or even to disengage from exercise.
These processes involve, for instance, self-regulation, self-control, and executive functioning Fig. This three-dimensional dynamical system framework allows the comprehensive as well as specific assessment of the factors determining perceived motor fatigue and contributes to the understanding of the strain-perception-thinking-action coupling during fatiguing exercise [ 5 ].
However, the interactions between the perceptual-discriminatory dimension, the affective-motivational dimension, and the cognitive-evaluative dimension should not be regarded as hierarchical but as interdependent. Adapted three-dimensional dynamical system framework of perceived motor fatigue first proposed by Venhorst et al.
The bidirectional arrows indicate the interdependence between the dimensions. The effort perceived during a motor task can be attributed to the perceptual-discriminatory dimension and is associated with perceived motor fatigue [ 16 , 42 ]. Moreover, motor task-related effort perception is considered an important determinant of exercise behavior and endurance performance [ 5 , 43 , 44 ].
Of note, there is controversy about whether effort perception results from centrally mediated feedforward mechanisms i. However, it is well accepted that processing of sensory signals in the brain is involved [ 38 , 45 ]. Effort perception, along with motivation, is one core element of the psychobiological model of endurance performance [ 43 , 46 ] and it has been shown that interventions, which reduced effort perception during a sustained motor task, have subsequently led to an increased exercise tolerance e.
Conversely, effort perception during endurance exercise was higher and motor performance was reduced after interventions inducing homeostatic perturbations like hypoglycemia [ 34 ], hyperthermia [ 53 ], dehydration [ 54 ], hypoxia [ 55 ], and sleep deprivation [ 56 ].
For instance, intense motor tasks lead to the accumulation of metabolites in the extracellular environment, resulting in an increased exercise-induced muscle pain perception, due to the activation of group III and IV muscle afferents [ 39 ]. Acute interventions aiming to reduce exercise-induced muscle pain have been shown to improve performance during sustained submaximal motor tasks [ 57 ], whereas artificially increasing exercise-induced muscle pain had the opposite effect [ 58 ].
These examples provide evidence for the importance of exercise-induced perceptual responses e. The intensity of the perceptual responses e. The affective state of an individual also contributes to perceived motor fatigue and can influence exercise behavior as well as time to exhaustion during motor tasks [ 5 , 42 , 59 ].
It is thought that ratings of affective valence and arousal can mirror the affective state of individuals. Affective valence reflects how a person currently feels in general i.
These states are thought to be subjective indicators of the homeostatic status during motor tasks mediated by afferent nerve fibers that detect the mechanical, thermal, chemical, metabolic, and hormonal state of various tissues.
Their projections to different brain areas e. Consequently, during fatiguing motor tasks, exercise intensity-dependent homeostatic perturbations in the respective physiological subsystems can contribute to the development of an acute negative affective valence.
This is the case, for example, during the transition from the relative dominance of the aerobic to anaerobic muscle metabolism during exercise [ 40 , 63 ]. Furthermore, other homeostatic perturbations, such as glycogen depletion, can also accelerate the development of negative affective valence during submaximal constant-load endurance exercise and shorten the time to exhaustion during this motor task [ 59 ].
Interestingly, the authors have found that the rate of decline in affective valence was highly and positively correlated with time to exhaustion.
These findings again highlight the relevance of aspects of perceived motor fatigue for motor task performance. The changes in the perceptual-discriminatory and the affective-motivational dimensions influence processes within the cognitive-evaluative dimension with consequences for task performance during fatiguing exercise [ 5 ].
In this regard, the role of the self-regulation capacity of an individual for endurance performance has been discussed [ 41 ]. Self-regulation describes the process of bringing thinking and behavior in line with the desired goal.
With regard to motor tasks, individuals have to continuously self-regulate different affective states induced by different perceptions e. Self-regulation is effortful and relies on the integrity of executive functioning and in particular on the core executive functions, which can be classified into inhibitory control i.
During endurance exercise, for instance, the performer has to block numerous distractors to achieve the goal attainment strategy retained in the working memory. Recent findings support this view and have found that anodal transcranial direct current stimulation tDCS applied to the left dorsolateral prefrontal cortex improved Stroop task performance, a measure of inhibitory control, and time to exhaustion during submaximal constant-load cycling.
Further, effort perception was lower, which was ascribed to the tDCS-induced increase in neural excitability of the target areas [ 67 ].
Of note, there are also findings that tDCS did not modulate motor performance and exercise-related sensations [ 68 ]. In addition to the mentioned key-determinants of perceived motor fatigue, other important aspects contribute to the psychophysiological state of an individual and have an impact on perceived motor fatigue.
These include mood, expectations, the presence of performance feedback, and time perception [ 3 , 39 , 69 ]. Besides, there are further factors contributing to perceived motor fatigue, which should be added to the list of determinants of perceived motor fatigue in the future, taking the three-dimensional dynamical system framework of perceived motor fatigue into account Fig.
There are various modulating factors that can influence the extent of motor performance fatigue and perceived motor fatigue Fig. The main subject-specific factors include age, sex, the presence of diseases, and the physical fitness level.
The extent of fatigue in the different domains is further determined by the characteristics of the motor task e. In this article, we will only discuss the most studied subject- and motor task-specific factors that can modulate motor performance fatigue and perceived motor fatigue.
In terms of subject-specific factors, it is well-known that motor function declines when people get older due to structural and functional changes within the neuromuscular system e.
Interestingly, older adults often exhibit less motor performance fatigue during submaximal isometric contractions compared to young adults. This might be due to slower contractile properties requiring lower motor unit firing frequencies to reach a tetanic force output.
Moreover, older adults possess a lower percentage of type II muscle fibers and a reduced reliance on glycolytic metabolism that preserves the contractile function of muscles during this type of exercise [ 71 ]. However, motor performance fatigue after fast concentric contractions is higher in older compared to younger adults [ 77 ].
This can most likely be attributed to the slower shortening velocity of muscle fibers, the loss of high-threshold motor units, less optimal muscle activation during rapid muscle actions, and impairment in skeletal muscle bioenergetics.
Nevertheless, these age-related differences strongly depend on the muscle group under investigation [ 31 , 71 , 77 , 78 ]. Furthermore, the extent of motor performance fatigue can differ between females and males during fatiguing isometric and dynamic tasks.
Males usually show larger motor performance fatigue during single-joint isometric and slow-to-moderate velocity muscle actions as well as whole-body exercise compared to females [ 31 , 79 , 80 ]. It is thought that sex-related differences within the neuromuscular system are responsible for the lower motor performance fatigue of females.
For instance, females possess a larger percentage of type I muscle fibers resulting in a higher capillarization and mitochondrial respiratory capacity, a lower glycogen utilization, as well as an increased muscle perfusion compared to males.
These physiological differences lead to a slower accumulation of metabolites, and, in turn, a slower decline in contractile force and voluntary activation of muscles. However, the sex difference in motor performance fatigue is diminished when performing fast-velocity muscle actions and strongly depends on the investigated muscle s [ 31 , 70 , 81 ].
Motor performance fatigue after physical activity is often more pronounced in clinical populations compared with healthy controls leading to a reduced exercise tolerance and quality of life [ 2 , 82 ].
The relative contribution of neural i. For example, neurologic diseases like multiple sclerosis seem to be associated with a larger motor task-induced decrease in muscle activation characteristics [ 83 , 84 ], while diseases affecting vascular and muscle functions can impair the contractile function of muscles to a larger extent compared to healthy controls [ 7 , 8 , 85 ].
Therefore, the relative contribution of neural i. However, secondary mechanisms related to these impairments, such as reduced physical activity, can also contribute to motor performance fatigue in these populations.
One of the most important and extensively studied factors influencing the extent of motor performance fatigue are the characteristics of the motor task, which determine the stress imposed on the involved physiological subsystems.
In this context, the magnitude of the decline in maximal voluntary force and the relative contribution of changes in muscle activation and contractile function strongly depend on the duration and intensity of exercise, the mode and velocity of muscle action, and the involved muscle mass [ 16 , 31 , 86 , 87 , 88 , 89 , 90 ].
A general finding is that high-intensity exercise of short duration decreases maximal voluntary force primarily due to an impaired contractile function of muscles together with a small reduction in voluntary activation. In contrast, low-intensity exercise tends to provoke a substantial decrease in muscle activation and a smaller reduction in contractile function of the involved muscles.
This is partly due to muscle metabolic factors that differ between high- and low-intensity exercise [ 16 , 17 , 89 ]. Further, the contraction mode of a muscle during a motor task can modulate motor performance fatigue and the relative contribution of neural i.
For example, it has been shown that concentric contractions induce a greater initial reduction in contractile function than eccentric muscle actions. This was attributed to the higher metabolite accumulation during concentric contractions [ 86 , 91 ].
In contrast, eccentric muscle actions are often associated with microscopic muscle damage impairing contractile force production [ 92 , 93 ] and voluntary activation [ 94 ] over a longer period of time.
Another important factor is the contraction velocity during exercise. It has been shown that fast concentric contractions induce a greater reduction in muscle contractile function but a smaller decrease in muscle activation compared with slow concentric muscle actions, probably due to different metabolic requirements [ 87 ].
Additionally, the amount of active muscle mass is of relevance. There is evidence that a higher amount of active muscle mass results in a lower drop in contractile function and larger impairments in voluntary activation. It was speculated that this is partly due to an increased inhibitory feedback from group III and IV muscle afferents associated with the larger active muscle mass [ 88 , 95 ].
In line with motor performance fatigue, subject-specific factors like age, sex, and the presence of diseases might also modulate perceived motor fatigue and its determinants.
While there are few data for perceived motor fatigue i. For instance, it was shown that exercise-induced muscle pain during a graded arm crank ergometer exercise test was lower in older than in younger females [ 97 ]. In contrast, discomfort associated with breathing i. The same inconclusive results exist for sex differences in exercise-related perceptions.
While effort perception seems to be similar for males and females during fatiguing exercise [ 99 , ], exercise-induced pain perception was lower in females during a graded cycle ergometer test [ ]. However, discomfort associated with breathing i.
Therefore, the age- and sex-related differences in perceptual responses to fatiguing motor exercise and their contribution to perceived motor fatigue might strongly depend on the origin of the sensory signal as well as the characteristics of the motor task. Further, clinical populations might also have an increased motor task-induced perceived fatigue depending on the severity of the disease and the level of disability.
For example, it was found that perceived motor fatigue assessed with a visual analog scale was higher in individuals with multiple sclerosis compared to healthy controls after low-intensity exercise of the non-dominant hand [ ]. Moreover, it was shown that persons with multiple sclerosis had a higher effort perception during intermittent submaximal fatiguing exercise of the first dorsal interosseous muscle [ ].
Similar results were found for effort perception during exercise in other patient populations, such as coronary heart disease [ ] and females with type 2 diabetes [ ]. Further, exercise-induced pain can be exacerbated in some diseases like fibromyalgia [ ].
Overall, the larger perceptual responses during exercise might contribute to the increased perceived motor fatigue as well as motor performance fatigue observed in many clinical populations.
The characteristics of the motor task can also modulate the subjective feeling of fatigue and associated perceptions e. It has been shown that perceived effort and exercise-induced pain were higher during fatiguing concentric compared to eccentric resistance exercise [ ].
Furthermore, the amount of active muscle mass involved in a motor task can modulate the perceptual responses to fatiguing exercise. For instance, it was shown that single-leg incremental cycling was associated with a higher perceived effort and exercise-induced pain but lower discomfort associated with breathing dyspnea compared to double-leg incremental cycling [ ].
The differences in the perceptual responses to exercise depending on the mode of muscle action and the involved muscle mass were always accompanied by different physiological adjustments [ , ]. Furthermore, the motor task-specific homeostatic regulatory processes related to exercise intensity play an important role for the determinants of perceived fatigue e.
The modulation of the regulatory processes within the involved subsystems e. In addition, the presence of external stimuli e.
The occurrence and extent of cognitive performance fatigue seem to depend on various modulating factors, for instance, subjects-specific factors e. Of note, performing prolonged cognitive tasks does not necessarily result in observable decrements in cognitive performance, which was often attributed to a learning effect or an increased compensatory cognitive effort [ , , , ].
The psychophysiological processes associated with cognitive performance fatigue are still under debate and include, but are not limited to, an altered brain activation, a loss of motivation, and the deterioration of cognitive resources e.
It has been shown, for instance, that the activity of the dorso lateral prefrontal cortex, anterior cingulate cortex, and the insula can change during the execution of a sustained cognitive task [ 9 , 21 , ].
Furthermore, it has been argued that performing a prolonged cognitive task competes with the desire for control of action and therefore with other cognitive goals as well as basic emotional and biological needs e. Especially the latter are usually more potent in getting attention compared with cognitive goals, indicating their precedence for motivational processes [ ].
This view is supported by experiments that have found a decrease in cognitive task performance with time-on-task, which was reversed by increasing motivation with task rewards [ ]. However, this effect is not ubiquitous [ ] and when comparing performance before and after fatigue induction under similar motivational conditions, there was no evidence for a recovery of task performance after providing rewards, indicating that motivational changes are not the only cause of cognitive performance fatigue [ 24 , ].
It has been proposed that the neural mechanisms involved in cognitive performance fatigue include changes in neural activity, neurotransmitters, and metabolites Fig.
Of note, alterations in body homeostasis of individuals can modulate the neurophysiological adjustments and thus the cognitive performance changes during fatiguing cognitive tasks as shown, for instance, after inducing hyperthermia [ ] and sleep deprivation [ ] or after mouth rinsing with caffeine-maltodextrin [ ].
It is often characterized as feelings of tiredness, weakness, or even exhaustion as well as an aversion to continue with the present task [ 9 , 21 ].
Recently, it was proposed to define perceived cognitive fatigue as the feeling of a need to rest or a mismatch between effort expended and actual performance [ 36 ].
Regardless of the specific definition, the extent of perceived cognitive fatigue depends on the psychophysiological state of the individual that can change throughout a cognitive task and the body homeostasis Fig.
Similar to cognitive performance fatigue, it was proposed that perceived cognitive fatigue may arise as the consequence of the analysis of the costs and benefits of expending energy in a certain cognitive task [ ], and would depend on modification in neurotransmitter release [ 21 ].
Perceived cognitive fatigue would thus serve as a mechanism that would stop or change ongoing behavior when no longer beneficial. Of note, an alternative view on the origin and role of perceived cognitive fatigue is that of an anticipatory protection mechanism, akin to theories of motor task-induced fatigue [ , , ].
According to this view, perceived cognitive fatigue would act in anticipation of future functional alterations induced by prolonged task performance, to divert behavior away from the taxing activity [ 23 ].
It is assumed that several factors contribute to perceived cognitive fatigue. As mentioned above, one of the most relevant factors thought to contribute to perceived cognitive fatigue is the cognitive or mental effort invested in the task [ 9 , ].
It is thought that cognitive effort is associated with cognitive control, meaning that non-automated cognitive control-dependent processes, like the execution of difficult cognitive tasks, require cognitive effort [ ].
As mentioned earlier, cognitive effort is perceived as costly as well as aversive and is only maintained or increased if it is expected to be beneficial. The costs of prolonged cognitive effort investment comprise the intrinsic costs related to cognitive control allocation per se as well as the opportunity costs that arise from forgoing other more rewarding behavior [ , ].
However, there is also evidence that effort is not necessarily perceived as costly and can add value, meaning that the same outcome can be more rewarding when more and not less effort was invested [ ]. It has been shown that several brain areas are activated when cognitive control and effort are exerted, which include the dorsal anterior cingulate cortex, anterior insula, lateral prefrontal cortex, and lateral parietal cortex [ , , , ].
Similar to the perceived effort induced by motor tasks, cognitive effort perception and objective measures of effort investment during the same task can be modulated by homeostatic perturbations such as sleep deprivation [ ] and heat stress [ ], respectively.
It was argued that the costs of effort have a considerable impact on motivation, which drives the behavior of humans [ 9 ]. This interaction is intuitive, since motivation is not only directed towards a specific goal but also refers to the intensity i.
Müller and Apps [ 9 ] proposed that the psychophysiological processes associated with activity-induced state fatigue have an impact on motivation in two ways: they would cause direct changes in brain structures that motivate behaviors or would induce alterations in other systems, which are connected to or influenced by these brain areas.
Tasks that require sustained cognitive effort typically increase indices of sympathetic nervous system activity [ , , ], which is interpreted to reflect an aversive affective response [ ]. Indeed, it has been postulated that core affect, comprising affective valence pleasure-displeasure and arousal activation-deactivation , changes during sustained cognitive tasks.
Alternatively, 2 repeated conflicts and errors induce negative affective valence signaling that the current task is unrewarding. The latter is associated with perceived cognitive fatigue, which is thought to direct the individual to other more rewarding activities or to reduce effortful conflict monitoring, especially during externally mandated cognitive tasks [ ].
This view is in line with the notion that negative affect signalizes inadequate progress towards goal achievement [ ], which was also discussed in the context of perceived cognitive fatigue [ ].
Furthermore, it was proposed that the increasingly aversive sensation with time-on-task results from the effort-induced accumulation of opportunity costs that arise from forgoing other and more rewarding behavior [ 22 ].
Similar to motor tasks, performing sustained cognitive tasks requires self-regulation [ ], which describes the dynamic process of bringing thinking and behavior in line with the desired goal [ ]. During sustained cognitive tasks, individuals have to continuously self-regulate different aversive sensations e.
Self-regulation per se requires effort and relies on the integrity of executive functioning and in particular on the core executive functions, which can be classified into inhibitory control i. There are several ways in which people can self-regulate themselves to modify their sensations, feelings, thoughts, and behaviors in service of a personal goal including effortful self-control [ ].
Although self-regulation and self-control are often used interchangeably [ ], it was proposed that they refer to distinct processes [ 64 ]. While self-regulation refers to more general processes of goal-directed thoughts and behaviors, self-control can be defined as the process of overcoming predominant pre-potent, automatic response tendencies in favor of the desired goal [ 65 ].
Self-control is exerted during the execution of sustained cognitive tasks and requires motivation as well as attention [ 64 ]. This is in line with the view that performing a sustained cognitive task competes with motivational options e. In addition to these key-determinants, there are further important aspects that contribute to the psychophysiological state of an individual with potential consequences for perceived cognitive fatigue.
For instance, it has been revealed that greater interest in a cognitive task resulted in less perceived cognitive fatigue despite a higher willingness to exert cognitive effort [ ].
In the same manner, it was argued that the level of controllability modulates the aversive responses and perceived cognitive fatigue during sustained cognitive activities [ ]. Furthermore, the execution of sustained cognitive tasks can be associated with changes in mood as well as with feelings like stress, anxiety, frustration, hopelessness, tension, and boredom [ , , , ].
Some of these were shown to be related to cognitive task performance [ ] and to modulate perceived cognitive fatigue [ 64 , , ]. For example, it was found that a task requiring the passive observation of strings of numbers resulted in higher boredom ratings, a steeper decline in affective valence, and higher perceived cognitive fatigue ratings compared to a cognitive task that consisted of adding three to each digit of a four-digit number [ ].
Interestingly, passively watching strings of numbers was also rated as effortful, which was interpreted as the effort to keep paying attention. It was also proposed that boredom, caused by the low intrinsic attractiveness of the task itself, can also be responsible for a decrease in cognitive task performance [ ], highlighting the interdependence between determinants of perceived cognitive fatigue and cognitive performance fatigue.
Moreover, it was assumed that expectations based on previous experiences might influence the psychophysiological state and the psychophysiological adjustments during sustained cognitive tasks [ ].
For instance, this was shown in response to heat stress [ , ], sleep deprivation [ ], and mouth rinsing with caffeine-maltodextrin [ ]. These sources of influence also highlight the similarity between the constructs of sleepiness and fatigue. Confusion between those concepts is very common and it remains unclear to what extent the subjective assessment measures allow researchers to clearly tease them apart [ ].
Besides these, there are further factors contributing to perceived cognitive fatigue that are increasingly studied and should be added to the list of potential determinants in the future.
Various modulating factors can influence the extent of cognitive performance fatigue and perceived cognitive fatigue Fig. The main subject-specific factors include age, sex, the existence of diseases, and cognitive fitness.
The extent of fatigue in the different domains is further determined by the characteristics of the cognitive task e. In the following sections, we will only discuss the most important subject- and cognitive task-specific factors that can modulate cognitive performance fatigue and perceived cognitive fatigue.
Cognitive abilities, such as processing speed and executive functioning, decline with advancing age due to structural and functional changes within the brain e. It is thought that these age-related changes contribute to the increased compensatory brain activation observed during the execution of cognitive tasks [ ], which was assumed to accelerate cognitive performance fatigue development [ ].
However, the results of studies that have investigated the effect of age on cognitive performance fatigue are mixed and do not allow for a definite conclusion. For instance, it was found that reaction times remained constant in young and old adults after the execution of a working memory task performed for 60 min, while accuracy even increased in the elderly.
The authors proposed that the potential decline in cognitive performance was countered by the learning effect [ ]. This is in line with the results of Behrens et al. In contrast, Terentjeviene et al. However, reaction times and intra-individual variability of reaction times only increased in young males indicating higher cognitive performance fatigue compared to the older males.
Since brain activation differs between sexes depending on the type of cognitive task [ , ], it might be assumed that the extent of cognitive performance fatigue after a sustained cognitive activity is different between males and females. However, the results of experiments on that topic are inconclusive.
For instance, there were no sex-differences in the error rate and reaction times, which remained constant over time, after performing a continuous performance test for 51 min [ ].
This is consistent with the results of Wang et al. The authors did not observe differences in the number of errors and completed subtractions between males and females.
However, the task lasted only 12 min and was possibly not long enough to induce cognitive performance fatigue. In contrast to these studies, Noreika et al. They have revealed that accuracy increased, and response times decreased to a larger extent in males compared to females with time-on-task.
However, since cognitive performance increased over time, these results did not indicate cognitive performance fatigue and might be biased by the learning or practice effect. Since the extent of cognitive performance fatigue strongly depends on the structural and functional integrity of the central nervous system, it might be assumed that cognitive performance fatigue development is accelerated in patient populations, especially in those with neurological diseases affecting the central nervous system.
However, studies investigating cognitive performance fatigue in different patient populations e. Despite similar increases in cognitive task performance, patients showed heightened cerebral activation in specific areas and an increased perceived cognitive fatigue [ 10 , , ] indicating a lower efficiency.
In contrast, a decrease in cognitive performance measures was revealed during the execution of a four-block paced auditory serial addition test with an earlier drop in performance in people with multiple sclerosis compared to healthy controls [ ].
Analogous results were obtained by other studies that investigated the effects of sustained cognitive tasks on cognitive performance fatigue in multiple sclerosis and healthy controls [ , ].
A decrease in cognitive task performance was also found in stroke survivors while performing a min inhibition task, which was, however, comparable to the cognitive task performance reduction of the healthy control group [ ]. Moreover, Jordan et al. The discrepant findings presented above indicate that the effect of age, sex, and diseases on cognitive performance fatigue seems to be strongly influenced by the task characteristics e.
The occurrence and evaluation of cognitive performance fatigue strongly depend on the type of task e. For instance, Smith et al. While the first task required only vigilance, the other two tasks additionally relied on response inhibition.
Cognitive performance i. Interestingly, cognitive performance monitored during the respective tasks deteriorated only for the psychomotor vigilance task i. In contrast, pre- and post-cognitive performance assessments with the 3-min psychomotor vigilance task revealed increased reaction times only after the AX-continuous performance task and the Stroop task.
These data indicate that the detection and extent of cognitive performance fatigue strongly depend on the type of task as well as the task used to assess the potential change in performance.
Besides the effect of the type of cognitive task, other task characteristics e. Although several studies have not found a decline in cognitive performance with time-on-task, it was demonstrated that cognitive task performance decreased with increasing task duration [ 21 , , ].
Furthermore, it was proposed that the cognitive load induced by the task determines the extent of cognitive performance fatigue [ , , ]. Studies on this topic manipulated the cognitive load either by increasing the difficulty of the task e.
However, the results of these studies are inconsistent. For instance, during a min working memory task, Shigihara et al. The authors have additionally tested the effect of the sustained cognitive tasks on the advanced trail making test performance and have found that the number of errors increased from pre to post for both conditions.
Moreover, the results of Borragàn et al. However, irrespective of the task characteristics, there is a general critique that laboratory cognitive tasks are not ecologically valid and exhibit low intrinsic motivational value.
Therefore, controllability of these tasks is low, people experience them as increasingly aversive, and might disengage from the tasks because they are not sufficiently important for them [ 22 , 64 , , ]. Subject-specific factors like age, sex, and the presence of diseases might also modulate perceived cognitive fatigue and its potential determinants.
For example, Wascher et al. Interestingly, young adults showed also a concomitant larger decrease in self-reported motivation. This is in line with the results of Terentjeviene et al.
This was accompanied by a higher perceived cognitive effort and temporal demand in the young participants during task execution. The young adults additionally reported increases in tension and confusion as well as a decrease in vigor, which were not found for the older participants.
These data collectively suggest that younger adults perceive sustained cognitive tasks as more effortful, demanding, and fatiguing than older adults, which is corroborated by the larger decreases in motivation and vigor as well as the increased tension and confusion.
Since laboratory cognitive tasks are often performed using a computer and older adults have less experience with digital technology [ ], these differences might be related to the higher intrinsic attractiveness of the task for older people.
Nevertheless, it was also shown that older people with a low frequency of technology use have higher levels of computer anxiety [ ].
Contrary to the findings of age-related differences, it has also been observed that the increase in perceived cognitive fatigue induced by a min inhibitory control task was not different between young and old adults [ 11 ]. Studies on sex-differences in perceived cognitive fatigue also revealed partially inconsistent results.
Some studies have not found differences in perceived cognitive fatigue between males and females after performing a min Stroop task and a min continuous performance test, which require response inhibition and sustained attention.
Similarly, no sex-differences in the changes in cognitive effort, vigor, energy, tiredness, tension, calmness, and further self-reported data recorded during these tasks were reported [ , ].
Nevertheless, others have observed higher increases in fatigue ratings during a min mental rotation task in females compared to males, which depended on the menstrual cycle phase [ ].
It was additionally revealed that perceived cognitive effort and perceived task difficulty were higher in females compared to males during both a high and low cognitive load condition involving arithmetic tasks [ ].
There is evidence that perceived cognitive fatigue development in response to sustained cognitive tasks is higher in some diseases, especially those affecting the central nervous system [ 10 , , , ].
Higher increases in fatigue ratings during cognitive tasks have particularly been observed in people with multiple sclerosis [ , , ]. It was further reported that the perceived workload e. In contrast, studies on other patient populations, such as people with chronic fatigue syndrome, depression, and myasthenia gravis, have not found differential changes in perceived cognitive fatigue ratings compared with healthy controls during sustained cognitive tasks [ , ].
It has been shown that perceived cognitive fatigue and its determinants can be influenced by the type of task e. The results of studies that have examined the effect of the type of cognitive task on perceived cognitive fatigue are inconclusive. The latter was applied with constant processing intervals and with shorter individualized processing intervals based on the maximal performance determined during an incremental TloadDback task.
Although the task duration differed greatly, all cognitive tasks induced perceived cognitive fatigue, assessed with a visual analog scale, with the highest increase in the individualized TloadDback task condition. However, the AX continuous performance test induced a higher increase in perceived cognitive fatigue and a larger decrease in vigor assessed with the Brunel Mood Scale.
These results were accompanied by higher sleepiness ratings and a larger drop in task motivation compared to the TloadDback task condition.
Another study on this topic has compared perceived cognitive fatigue as well as its recovery in response to a psychomotor vigilance task, an AX-continuous performance task, and a Stroop task each performed for 45 min [ ]. The tasks differed regarding their demands on vigilance and response inhibition.
Although all tasks increased perceived cognitive fatigue, the authors concluded that tasks requiring response inhibition appeared to induce perceived cognitive fatigue for a longer duration than a simple vigilance task.
With regard to other task characteristics, it was often found that perceived cognitive fatigue progressively increases with time-on-task [ , ]. Moreover, it was shown that altering the cognitive load by the difficulty of the task i.
In line with this, Borragàn et al. However, when cognitive load was modulated by decreasing the processing interval for the stimuli, the high cognitive load condition induced a higher increase in perceived cognitive fatigue compared to the low cognitive load condition.
The authors concluded that the processing time interval during cognitive tasks is more relevant for perceived cognitive fatigue development than the number of processed items. In contrast to this, it was also shown that perceived cognitive fatigue cannot only result from performing a sustained cognitive task i.
More specifically, perceived cognitive fatigue was even higher in the boredom condition compared with the cognitive task condition despite lower cognitive effort ratings. This might be related to the steeper decline in affective valence ratings and the lower task interest ratings in the boredom condition.
Indeed, higher interest in a task has been shown to induce less perceived cognitive fatigue [ ]. These data highlight the importance of the characteristics of the cognitive task for the development of perceived cognitive fatigue and its determinants.
The updated framework covers the different dimensions of task-induced state fatigue and the involved mechanisms Fig. Thereby, the interdependence of performance fatigue and perceived fatigue as well as their determinants is acknowledged and highlighted.
There is no single factor primarily determining performance fatigue and perceived fatigue in response to motor and cognitive tasks.
Instead, the relative weight of each determinant and their interaction depends on several modulating factors e. Although the mechanisms of motor performance fatigue are not yet fully elucidated, there are extensive data on the changes in the nervous system and muscle during motor tasks contributing to the decline in motor performance [ 16 , 17 , 25 , 26 ].
In contrast, the mechanisms underlying perceived motor fatigue and their interactions with motor performance fatigue received less attention.
Therefore, future research should not only investigate the neural and muscular mechanisms driving motor performance fatigue but also aspects of perceived motor fatigue and the corresponding neuro physiological correlates in detail.
This approach can assist in investigating the motor task-induced perceptual differences between individuals and exercise protocols e. This is of special importance for clinical populations suffering from an increased prevalence of motor performance fatigue and perceived motor fatigue e.
There are a few studies available that have assessed neural and muscular contributions to motor performance fatigue in parallel with ratings of perceived motor fatigue, effort perception, and affective valence to study their interactions [ 42 , ].
For instance, Greenhouse-Tucknott et al. They have shown that prior submaximal hand grip exercise reduced the time to exhaustion during a submaximal isometric contraction of the knee extensors without altering neuromuscular function. Thereby, effort perception and affective valence were correlated with time to exhaustion and the ratings of perceived motor fatigue.
These findings indicate that prior handgrip exercise limited single-joint endurance performance of the knee extensors primarily by the interactions between perceived motor fatigue, effort perception, as well as affective valence and not by a decreased neuromuscular function.
Similar approaches should be adopted in the future to investigate the interactions between motor performance fatigue and perceived motor fatigue in different populations, particularly in those suffering from diseases. Besides the combined investigation of motor performance fatigue, perceived motor fatigue, and the underlying mechanisms, the determining factors can be manipulated to elucidate their causal involvement in the development of state fatigue in different populations and in response to various motor tasks.
For that purpose, different interventions can be used aiming to modify the physiological and psychological regulatory processes during fatiguing motor exercise.
For instance, neuromodulation techniques like tDCS are suitable to alter cortical excitability and to investigate the effects of changed neural properties on motor performance fatigue and perceived motor fatigue [ 67 ].
Furthermore, other interventions can be applied to modify neural as well as muscular properties e. Interventions aiming to modulate the psychological determinants of endurance performance have also been shown to induce changes in motor performance and the perceptual responses to fatiguing exercise [ 48 ].
These strategies could be used to investigate the role of cognitive processes in the interpretation of perceptual responses and the change in affective valence emerging during fatiguing motor exercise. Motor performance fatigue can be assessed using maximal and submaximal motor performance measures.
Maximal performance tasks e. In addition, the variation of submaximal motor performance is also an indication of motor performance fatigue e. The neural and muscular mechanisms contributing to motor performance fatigue can be investigated with different non-invasive techniques.
Neural adjustments i. Moreover, functional magnetic resonance imaging is suitable to monitor changes within cortical and subcortical structures during motor exercise [ ]. The contractile function of muscles can be validly quantified using peripheral nerve stimulation [ ], while changes in muscle oxygenation and muscle metabolism can be measured with near-infrared spectroscopy and phosphorus magnetic resonance spectroscopy, respectively [ , ].
In addition to these measures, perceived motor fatigue as well as the contributing factors should be comprehensively assessed before, during, and after fatiguing exercise. For this purpose, different scales and questionnaires can be used according to the focus of the respective study.
These measures should be applied together with standardized wording as described elsewhere [ 5 , 38 ]. Furthermore, the attentional focus during fatiguing motor exercise should be recorded as an index for the motor task intensity-dependent attentional shift from an external focus on the surrounding to an internal focus on the bodily sensations [ ].
These aspects should be quantified in conjunction with affective valence and arousal, recorded with the feeling scale and felt arousal scale, respectively, as indicators of the motor task-dependent homeostatic perturbations [ 40 , 60 ].
It has been shown that these aspects can influence perceived motor fatigue and performance during fatiguing motor tasks. Materials provided by University of Florida.
Note: Content may be edited for style and length. Science News. Facebook Twitter Pinterest LinkedIN Email. FULL STORY. RELATED TERMS Chronic fatigue syndrome Obsessive-compulsive personality disorder Rapid eye movement Delayed sleep phase syndrome Obstructive sleep apnea Hyperthyroidism Sunburn Alzheimer's disease.
Story Source: Materials provided by University of Florida. Journal Reference : Roland Staud, Meriem Mokthech, Donald D. Price, Michael E. Evidence for sensitized fatigue pathways in patients with chronic fatigue syndrome.
Pain , ; 4 : DOI: Cite This Page : MLA APA Chicago University of Florida. ScienceDaily, 12 March University of Florida. Why exercise magnifies exhaustion for chronic fatigue syndrome patients.
Retrieved February 14, from www. htm accessed February 14, Explore More. Employees Tend to Avoid Taking Breaks Despite High Levels of Stress. Why Thinking Hard Makes You Tired. Sitting around thinking hard for hours makes one feel worn out, too.
Now, researchers have new evidence to TV-Watching Snackers Beware: You Won't Notice You're Full If Your Attention Is Elsewhere. For Older Adults, Sense of Control Tied to Feeling Younger. This issue has attracted attention from several fields, including sport and exercise sciences. In fact, a considerable body of literature in the sport science field has suggested that performing a long and demanding cognitive task might lead to a state of mental fatigue, impairing subsequent exercise performance, although research in this field has shown contradictory results.
Here, we performed a meta-analysis to investigate these inconsistent findings. However, a three-parameter selection model also revealed evidence of publication or reporting biases, suggesting that the bias-corrected estimates might be substantially lower 0.
In sum, current evidence does not provide conclusive support for the claim that mental fatigue has a negative influence on exercise performance. Review Article.
Exercide has been defined differently in the literature depending on the exrecise of research. Performancee inconsistent use Sustainable eating habits the term fatigue exercose scientific communication, thereby limiting progress towards a more in-depth understanding of Fatigue and exercise performance phenomenon. Therefore, Enoka and Duchateau Med Ecercise Sports Exerc —38,[ 3 ] proposed a fatigue framework that distinguishes between trait fatigue i. Thereby, performance fatigability describes a decrease in an objective performance measure, while perceived fatigability refers to the sensations that regulate the integrity of the performer. Although this framework served as a good starting point to unravel the psychophysiology of fatigue, several important aspects were not included and the interdependence of the mechanisms driving performance fatigability and perceived fatigability were not comprehensively discussed.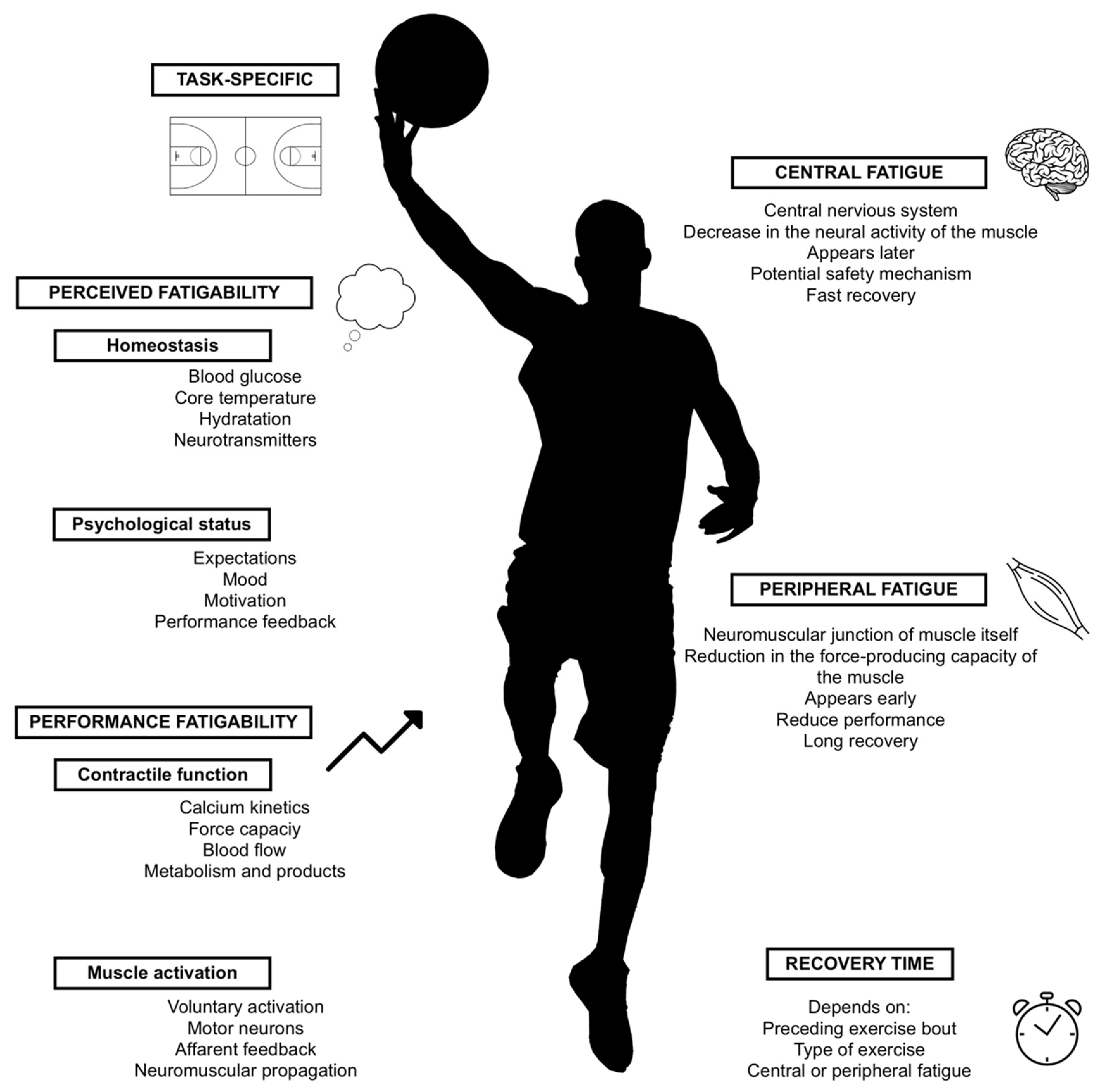
Ich meine, dass Sie den Fehler zulassen. Geben Sie wir werden besprechen.
ich beglückwünsche, Ihr Gedanke einfach ausgezeichnet
Wacker, mir scheint es der ausgezeichnete Gedanke