Video
How To Create Strength (Dr. McGill Explains Neural Drive)ZOLADZ; Training-induced Protein and athletic injury recovery of oxidative phosphorylation in skeletal muscles. Biochem J 15 August Biochsmical 1 : 37— Biochemlcal, the Biochhemical underlying Traininb adaptation are still trainingg understood fully.
Sign In or Biochemical training adaptations an Adaltations. Search Dropdown Menu. header search search input Search input adaptstions suggest. filter your search All Content Adaptatinos Biochemical training adaptations Biochemical Journal.
Advanced Search. Sign In. Toggle Menu Menu Issues Issues Issue covers Accepted Manuscripts Collections Adaptationz themed collections Open themed collections Curated content on mitochondria Authors Biohemical checklist Blochemical to authors Language-editing services Why publish with us?
Weight loss aids life Coenzyme Q and statins Submit your work Biochemicao access options and prices China-based researchers Superfoods for performance and Readers Transition adaptatioons open Policy Open access policy Adaptatiojs policy Trainong for reviewers Preprints policy Adaptatios policy About Biochemicxl Editorial board Elite Athlete Training Programs and information Adaptatiohs award winners Biochemical training adaptations Press Biochemical Society Ttraining.
Skip Biofhemical Destination Close Biohcemical menu Adaptatiohs navigation. VolumeIssue 1. Previous Bikchemical Next Article. All Biochemiical. Article Navigation. Research Article August 15 Gronostajowa 7, Kraków, Adaptatiohs.
This Site. Google Scholar. Jerzy A. ZOLADZ Jerzy A. Jana-Pawla II 78, Kraków, Poland. Author and article information. Publisher: Portland Press Ltd. Received: April 07 Revision Received: May 06 Accepted: May 13 Accepted Manuscript online: May 13 Online ISSN: The Biochemical Society, London © Biochem J 1 : 37— Article history Received:.
Revision Received:. Accepted Manuscript online:. Get Permissions. toolbar search Search Dropdown Menu. toolbar search search input Search input auto suggest.
You do not currently have access to this content. View full article. Sign in Don't already have an account? Sign in to your personal account. You could not be signed in.
Please try again. Sign In Reset password. Biochemical Society Member Sign in Sign In. Sign in via your Institution Sign in via your Institution. Get Access To This Article Buy This Article. View Metrics. Cited By Web Of Science CrossRef Get Email Alerts Article Activity Alert. Accepted Manuscript Alert.
Latest Completed Issue Alert. Submit your work. Latest Most Read Most Cited Beyond the tail: the consequence of context in histone post-translational modification and chromatin research.
Identification of a mer peptide from the death domain of MyD88 which attenuates inflammation and insulin resistance and improves glucose metabolism. IL-4 activates futile triacylglyceride cycle for glucose utilization in white adipocytes.
AMPK activation suppresses leptin expression independently of adipogenesis in primary murine adipocytes. Online ISSN Print ISSN Submit Your Work Language-editing services Recommend to Your Librarian Request a free trial Accessibility. CONNECT Sign up for alerts Sign up to our mailing list The Biochemist Blog Twitter Facebook LinkedIn YouTube Biochemical Society Membership.
EXPLORE Publishing Life Cycle Biochemical Society Events About Portland Press. com Biochemical Society Company no. GB Facebook Twitter LinkedIn YouTube.
Privacy and cookies Accessibility © Copyright Portland Press. This Feature Is Available To Subscribers Only Sign In or Create an Account.
Close Modal. This site uses cookies. By continuing to use our website, you are agreeing to our privacy policy.
: Biochemical training adaptationsMolecular control of endurance training adaptation in male mouse skeletal muscle | Post, H. PubMed BBiochemical Central Google Scholar Pedersen, B. The datasets Biochemical training adaptations and Biochemical training adaptations adapttations the current Biochemcial are not publicly available but are available from the corresponding author, who was an organizer of the study. Fonts, Scripts and Unicode. The increased running distance completed during the Yo-Yo IR1 test in both SIT and SSIT groups could also verify this improvement. Rasouli Mojez M. |
Publication types | Mechanisms of stretch-induced muscle damage in normal and dystrophic muscle: role of ionic changes. doi: PubMed Abstract CrossRef Full Text Google Scholar. Armstrong, R. Eccentric exercise-induced injury to rat skeletal muscle. Balnave, C. Effect of training on eccentric exercise-induced muscle damage. Bassett, D. Limiting factors for maximum oxygen uptake and determinants of endurance performance. Sports Exerc. Benedini-Elias, P. Post-immobilization eccentric training promotes greater hypertrophic and angiogenic responses than passive stretching in muscles of weanling rats. Acta Histochem. Besson, D. Eccentric training in chronic heart failure: feasibility and functional effects. results of a comparative study. Bontemps, B. Downhill running: what are the effects and how can we adapt? a narrative review. Sports Med. Brookes, P. Calcium, ATP, and ROS: a mitochondrial love-hate triangle. Cell Physiol. Brown, G. The mitochondrial respiratory chain. Essays Biochem. Bryner, R. Effects of downhill treadmill running on uncoupling protein 3 mRNA expression. Buford, T. Effects of eccentric treadmill exercise on inflammatory gene expression in human skeletal muscle. Casillas, J. Effects of an eccentric training personalized by a low rate of perceived exertion on the maximal capacities in chronic heart failure: a randomized controlled trial. Google Scholar. Chavanelle, V. Comparison of oxygen consumption in rats during uphill concentric and downhill eccentric treadmill exercise tests. Sports Sci. Chen, W. Choksi, K. Oxidatively damaged proteins of heart mitochondrial electron transport complexes. Acta , 95— Close, G. Eccentric exercise, isokinetic muscle torque and delayed onset muscle soreness: the role of reactive oxygen species. CrossRef Full Text Google Scholar. Coffey, V. The molecular bases of training adaptation. Cornachione, A. Effects of eccentric and concentric training on capillarization and myosin heavy chain contents in rat skeletal muscles after hindlimb suspension. Cruz-Jentoft, A. Sarcopenia: revised European consensus on definition and diagnosis. Age Ageing 48, 16— Diaz-Canestro, C. Sex dimorphism of VO2max trainability: a systematic review and meta-analysis. Doyle, M. Eccentric cycling ergometer to address skeletal muscle dysfunction in hospitalised patients: ergometer design, construction and demonstration. BMJ Innov. Drexel, H. Metabolic and anti-inflammatory benefits of eccentric endurance exercise — a pilot study. Duan, C. Dufour, S. Eccentric cycle exercise: training application of specific circulatory adjustments. Duncan, C. Role of intracellular calcium in promoting muscle damage: a strategy for controlling the dystrophic condition. Experientia 34, — Egan, B. Exercise metabolism and the molecular regulation of skeletal muscle adaptation. Cell Metab. Ellis, R. Eccentric exercise in adults with cardiorespiratory disease: a systematic review. Enns, D. Alterations in sarcoplasmic reticulum function in female vastus lateralis with eccentric exercise. Fick, A. The development of heat by muscular activity. Science 2, — Flück, M. Cardiovascular and muscular consequences of work-matched interval-type of concentric and eccentric pedaling exercise on a soft robot. Franchi, M. Distinct modalities of eccentric exercise: different recipes, not the same dish. Skeletal muscle remodeling in response to eccentric vs. concentric loading: morphological, molecular, and metabolic adaptations. Frezza, C. Organelle isolation: functional mitochondria from mouse liver, muscle and cultured fibroblasts. Fridén, J. Eccentric exercise-induced injuries to contractile and cytoskeletal muscle fibre components. Acta Physiol. Frimpong, E. Muscle damage and repeated bout effect from high intensity non-eccentric exercises. Online 22, — Fu, C. MRI quantitative analysis of eccentric exercise-induced skeletal muscle injury in rats. Fukada, S. Role of damage and management in muscle hypertrophy: different behaviors of muscle stem cells in regeneration and hypertrophy. Biochimica et Biophysica Acta Mol. Cell Res. Giorgi, C. The machineries, regulation and cellular functions of mitochondrial calcium. Cell Biol. González-Bartholin, R. Changes in oxidative stress, inflammation and muscle damage markers following eccentric versus concentric cycling in older adults. Gremeaux, V. Does eccentric endurance training improve walking capacity in patients with coronary artery disease? a randomized controlled pilot study. Gunter, T. Calcium and mitochondria. FEBS Lett. Hahn, S. Downhill treadmill running trains the rat spinotrapezius muscle. Halestrap, A. What is the mitochondrial permeability transition pore? Harris-Love, M. Eccentric exercise program design: a periodization model for rehabilitation applications. He, F. Redox mechanism of reactive oxygen species in exercise. Hody, S. Human muscle proteome modifications after acute or repeated eccentric exercises. Hoppeler, H. Moderate load eccentric exercise; a distinct novel training modality. Howell, J. Muscle stiffness, strength loss, swelling and soreness following exercise-induced injury in humans. Hyldahl, R. Mechanisms and mediators of the skeletal muscle repeated bout effect. Sport Sci. Inoue, M. Mitochondrial generation of reactive oxygen species and its role in aerobic life. Isner-Horobeti, M. Effect of eccentric versus concentric exercise training on mitochondrial function. Muscle Nerve 50, — Jezek, P. Mitochondria in homeostasis of reactive oxygen species in cell, tissues, and organism. Julian, V. Eccentric training improves body composition by inducing mechanical and metabolic adaptations: a promising approach for overweight and obese individuals. Eccentric cycling is more efficient in reducing fat mass than concentric cycling in adolescents with obesity. Sports 29, 4— Klossner, S. Muscle transcriptome adaptations with mild eccentric ergometer exercise. Pflugers Arch , — Knuttgen, H. Effects of training with eccentric muscle contractions on exercise performance, energy expenditure, and body temperature. Kuznetsov, A. Analysis of mitochondrial function in situ in permeabilized muscle fibers, tissues and cells. Lacourpaille, L. Time-course effect of exercise-induced muscle damage on localized muscle mechanical properties assessed using elastography. LaStayo, P. Eccentric ergometry: increases in locomotor muscle size and strength at low training intensities. Eccentric muscle contractions: their contribution to injury, prevention, rehabilitation, and sport. Sports Phys. Elderly patients and high force resistance exercise—a descriptive report: can an anabolic, muscle growth response occur without muscle damage or inflammation? Lechauve, J. Breathing patterns during eccentric exercise. Lemire, M. Trail runners cannot reach vo2max during a maximal incremental downhill test. Lewis, M. Eccentric cycling emphasising a low cardiopulmonary demand increases leg strength equivalent to workload matched concentric cycling in middle age sedentary males. Sport 21, — Lipski, M. Cardio-pulmonary responses to incremental eccentric and concentric cycling tests to task failure. MacMillan, N. Eccentric ergometer training promotes locomotor muscle strength but not mitochondrial adaptation in patients with severe chronic obstructive pulmonary disease. Magalhães, J. Eccentric exercise transiently affects mice skeletal muscle mitochondrial function. Malm, C. Leukocytes, cytokines, growth factors and hormones in human skeletal muscle and blood after uphill or downhill running. McHugh, M. Recent advances in the understanding of the repeated bout effect: the protective effect against muscle damage from a single bout of eccentric exercise. Sports 13, 88— Merry, T. Mitohormesis in exercise training. Free Radic. Meyer, K. Eccentric exercise in coronary patients: central hemodynamic and metabolic responses. Minetti, A. Energy cost of walking and running at extreme uphill and downhill slopes. Molnar, A. Mitochondrial H2O2 production is reduced with acute and chronic eccentric exercise in rat skeletal muscle. Redox Signal. Mueller, M. Different molecular and structural adaptations with eccentric and conventional strength training in elderly men and women. Gerontology 57, — Nikolaidis, M. The effect of muscle-damaging exercise on blood and skeletal muscle oxidative stress: magnitude and time-course considerations. Nosaka, K. Muscle damage following repeated bouts of high force eccentric exercise. Pageaux, B. Progressively increasing the intensity of eccentric cycling over four training sessions: a feasibility study in coronary heart disease patients. Paschalis, V. A weekly bout of eccentric exercise is sufficient to induce health-promoting effects. Peñailillo, L. Muscle fascicle behavior during eccentric cycling and its relation to muscle soreness. Metabolic and muscle damage profiles of concentric versus repeated eccentric cycling. Pereira Panza, V. Benefits of dietary phytochemical supplementation on eccentric exercise-induced muscle damage: is including antioxidants enough? Nutrition 31, — Perrey, S. Comparison of oxygen uptake kinetics during concentric and eccentric cycle exercise. Pierrynowski, M. Effects of downhill or uphill training prior to a downhill run. Plaquevent-Hostache, G. Effectiveness of combined eccentric and concentric exercise over traditional cardiac exercise rehabilitation programme in patients with chronic heart failure: protocol for a randomised controlled study. BMJ Open 9:e Rattray, B. Heat exposure does not alter eccentric exercise-induced increases in mitochondrial calcium and respiratory dysfunction. Specific training improves skeletal muscle mitochondrial calcium homeostasis after eccentric exercise. Rocha Vieira, D. Eccentric cycle exercise in severe COPD: feasibility of application. COPD 8, — Roig, M. The effects of eccentric versus concentric resistance training on muscle strength and mass in healthy adults: a systematic review with meta-analysis. Eccentric exercise in patients with chronic health conditions: a systematic review. Rooyackers, J. Eccentric exercise training in patients with chronic obstructive pulmonary disease. Schlagowski, A. Muscle Nerve 54, — Sonobe, T. Intracellular calcium accumulation following eccentric contractions in rat skeletal muscle in vivo: role of stretch-activated channels. Toyomura, J. Efficacy of downhill running training for improving muscular and aerobic performances. Valladares-Ide, D. Activation of protein synthesis, regeneration, and MAPK signaling pathways following repeated bouts of eccentric cycling. Walsh, B. Effect of eccentric exercise on muscle oxidative metabolism in humans. Zoll, J. Physical activity changes the regulation of mitochondrial respiration in human skeletal muscle. Gene expression in skeletal muscle of coronary artery disease patients after concentric and eccentric endurance training. Keywords : oxygen consumption, free radicals, calcium, eccentric training, mitochondria. However, a growing amount of animal research suggests hyperplasia is a result of ATP-PC training, but only a few studies represent hyperplasia occurring in humans. Myosin heavy chain II: In addition to cross sectional muscle area increases hypertrophy ,. additional myosin heavy chain II isoforms are present as a result of ATP-PC training. Type I and Type II fiber area: ATP-PC training also has the ability to alter muscle fiber area. Heavily trained subjects depending on training intensity and volume have experienced major alteration in type II muscle fiber diameter in comparison to type I. Chronic ATP-PC training can have the ability to affect the aerobic metabolism of type I fibers. As type II fibers increase in cross-sectional size, mitochondrial and capillary densities decrease. The absolute mitochondrial and capillary volumes remain unchanged, however, substrate transport, oxygen utilization and carbon dioxide withdraw may be limited. The end result is a theorized decrease in cardiovascular capacity. ATP-PC training of high intensity and slow-speed utilizing Isokinetic loading may increase several biochemical mechanisms. Muscle glycogen content, CP, ATP, ADP, creatine, phosphorylase, phosphofructokinase and Krebs cycle enzyme activity are all speculated to experience increased levels under the presence of ATP-PC training. However, ATP-PC training at higher speeds, more related to Power Training, do not replicate the same biochemical results. Power training will elicit similar results as experienced with ATP-PC training, particularly the neuromuscular effects. However, in addition to the neural adaptations of muscle with ATP-PC training, power training also stresses the elastic components. Power training, often carried out with the utilization of plyometric loading and emphasizes the stress of the stretch shortening cycle SSC. More specifically, power training generally involves sudden eccentric stress the stretch of the muscle followed by a sudden, rapid fast movement concentric contraction. The results of power training are not only increases in muscle strength but also shortening muscle contractile time. Primary neuromuscular adaptations witnessed with power training are shortening the time of motor unit recruitment, particularly of the type IIx and IIa fibers and heightening the tolerance of motor neurons to greater intervention frequencies. Power training also activates greater intramuscular coordination. Improved linkage between nerve impulses and skeletal muscle, results in greater neuromuscular coordination of the agonist and antagonist muscles. This adaptation is similar to the discussion above regarding co activation. The magnitude of change and adaptation within skeletal muscle is vast. Largely contingent on the stress placed on upon the body from a training standpoint, muscle will respond and adapt differently, based on the type and modality of exercise. These adaptations are the major players for improved performance. Specifically, capillary content creates improved interchange of gasses, heat, waste and nutrients between the working skeletal muscle and the blood delivered during exercise. Increased myoglobin content has the capacity to store oxygen and release it, particularly when oxygen becomes sparse during muscle contraction during prolonged cardiovascular exercise. Given aerobic energy production occurs within the mitochondria, the improvement of its function, particularly in size and number, becomes an obvious player in improved cardiovascular performance. Lastly, from a cardiovascular standpoint, the efficiency of enzymatic activity is demonstrated to create mitochondrial changes which therefore serves as a vehicle for slower use of muscle glycogen and a reduced production of lactate during exercise at given intensities. This adaptation likely serves as a major prayer in a heighted lactate threshold. This neutralizing muscle adaption limits effect lactate has on skeletal muscle, therefore delaying performance fatigue. Training within the energy system of the ATP-PC system, results in primarily neuromuscular and contractile component adaptations such as hypertrophy and co-coordination of the muscles. Finally co-activation agonist and antagonist muscles improvements by reducing the amount of resistive force of antagonist muscle, is supported to contribute to greater force production of agonist muscles. Similarly, power training results in significant neuromuscular adaptations, however, the elasticity of muscle, specifically the SSC, is more pronounced within power training. Given that most power training involves sudden eccentric stress the stretch of the muscle followed by a sudden, rapid fast movement concentric contraction, chronic power training may lead to increases in muscle strength but also shortening muscle contractile time. Training for power also shortens the time of motor unit recruitment, particularly of the type IIx and IIa fibers, therefore heightening the tolerance of motor neurons to improve intervention frequencies. Specifically, a more expedited initiation and excitement of the discharge rate of type IIx and IIa muscle fibers will in turn increase the synchronization of motor units and the rate of firing. The plasticity of muscle is highly contingent on the stress placed upon it and the adaptions are extensively different and described above. This is an open access article distributed under the terms of the, which permits unrestricted use, distribution, and build upon your work non-commercially. About Us Paper Submission FAQs Testimonials Videos Reprints Pay Online Article Processing Charges Contact Us Sitemap. Home Open Access Journals eBooks Information For Author Article Processing Charges. Publication Ethics. Peer Review System. Behavioral Sciences Food and Nutrition Trends Global Trends in Pharmaceutical Sciences. Home MOJSM Physiological and physical adaptations within the working muscle specific to cardiovascular training lactate training adenosine triphosphate phosphocreatine training and power training. Research Article Volume 1 Issue 4. ATP-PC: Adensosine Triphosphate-Phosphocreatine; SSC: Stretch Shortening Cycle. Neuromuscular One of the most significant adaptations of ATP-PC training that explains much of the gains that are made by the athletes that are trained are neural. The first, Transient lasts only a short time immediately after a training bout and is caused by fluid buildup from blood plasma in the interstitial and intercellular spaces within muscle. Author declares there is no conflict of interest in publishing the article. Brooks GA, Fahey TD, Baldwin KM. Exercise physiology: Human bioenergetics and its applications, 4th edition. McGraw Hill, USA; Sanz RJ, Rankinen T, Joanisse DR, et al. Familial resemblance for muscle phenotypes in the HERITAGE family study. Med Sci Sports Exerc. Wilmore JH, Costill DL. Physiology of sport and exercise, 3rd edition. Human Kinetics, USA; Lewis MI, Fournier M, Wang H, et al. J Appl Physiol. Hermansen L, Wachtlova M. Capillary density of skeletal muscle in well-trained and untrained men. Holloszy JO, Coyle EF. Adaptations of skeletal muscle to endurance exercise and their metabolic consequences. J Appl Physiol Respir Environ Exerc Physiol. Greiwe JS, Hickner RC, Hansen PA, et al. Effects of endurance exercise training on muscle glycogen accumulation in humans. Juel C. Muscle pH regulation: role of training. Acta Physiol Scand. Hawley JA, Hargreaves M, Joyner MJ, et al. Integrative Biology of Exercsie. Aagaard P, Simonsen EB, Andersen JL, et al. Neural inhibition during maximal eccentric and concentric quadriceps contraction: Effects of resistance training. Gee MSL, Swinton C, Morrison S, et al. Compensatory regulation of HDAC5 in muscle maintains metabolic adaptive responses and metabolism in response to energetic stress. Enoka RM. Neural adaptations with chronic physical activity. J Biomech. Geng T, Li P, Okutsu M, et al. PGC-1α plays a functional role in exercise-induced mitochondrial biogenesis and angiogenesis but not fiber-type transformation in mouse skeletal muscle. Am J Physiol Cell Physiol. Rowe GC, Khoury R, Patten IS, et al. PGC-1α is dispensible for exercise induced mitochondrial biogenesis in skeletal muscle. |
REVIEW article | Physiological and physical adaptations within the working muscle specific to cardiovascular training, lactate training, adenosine triphosphate-phosphocreatine training and power training. MOJ Sports Med. DOI: Download PDF. Objectives: The purpose of the present review study is to review the existing literature regarding the physiological and physical adaptations within working skeletal muscle specific to cardiovascular training, lactate training, adenosine triphosphate-phosphocreatine training and power training. Methods: A search was conducted on the wide-body of research that exists in and around the skeletal muscle and sports performance and aligns the research in a clear manner, specifically describing the physiological response of various training to cellular muscle. Literature gathered involved trails of comparative analysis with control groups in various exercise settings. Results : In an attempt to clarify the physiological adaptations to working skeletal muscle, the purpose of this review is to determine what affect 4 different types of conditioning reflects in terms of structural, metabolic, enzymatic, neuromuscular and contractile. The present study identifies each adaptation specific to the training modality to clarify the scientific evidence for the sport practitioner. Keywords: Muscle adaptations; Adenosine triphosphate; Cardiovascular training; Power training. The capacity for change within skeletal muscle is immense. Represented by gene expression on a molecular level, the alteration of muscle generally results in an increase or decrease in the amount of muscle proteins. When exercise stress is placed upon the skeletal muscle, the magnitude of adaptation is highly contingent upon plasticity of muscle. Thus, the Principal of Myoplasticityis the specific term that represents the capacity of muscle that is modifiable for adaptation and applies to the ensuing discussion related to muscular training adaptations. Cardiovascular training on a structural level elicits a significant increase in activation frequency of motor units and a slight increase in oppositional load against motor units. Quintessential cardiovascular training that presents this stimulus to the muscle is represented in weight bearing settings i. Cardiovascular training results in little to no effect on the cross-sectional area of muscle and muscle fibers. However, specific to type I slow-twitch fibers, these fibers may experience slight increases in cross sectional size due to increases glycogen storage as well as oxidative metabolism. The adaptations to muscle under the influence of cardiovascular training produce changes in fiber type, capillary supply, myoglobin content, mitochondrial function and oxidative enzymes. Cardiovascular training relies primarily on type I, slow-twitch muscle fibers. In response to the volume and intensity of training, type I fibers have the capability to become larger in cross-sectional area. Similarly, most literature suggests little change in muscle fiber percentage in slow-twitch and fast-twitch fibers; however, changes have been documented in fast-twitch subtypes. Type IIx fast-twitch fibers have called upon less during cardiovascular training, consequently, possess a lower aerobic capacity. Recent literature produced by the HERITAGE study suggests that type IIx fast-twitch fibers may transform to type IIa and it is even speculated they may be capable of transitioning to slow-twitch. However, very minuscule results have been published. A significant adaptation to cardiovascular training is the increase in capillaries surrounding the muscle fibers. Literature has documented that cardiovascular trained individuals have significantly greater amounts of capillary density than compared to sedentary populations. Upon entering the muscle, oxygen binds with an iron-containing compound that resembles hemoglobin called myoglobin. Myoglobin transports oxygen molecules from the cell membrane to the mitochondria. Of importance to cardiovascular training, myoglobin has the capacity to store oxygen and release it, particularly when oxygen becomes sparse during muscle contraction. The release of oxygen often occurs during the lag time between the beginning of exercise and the amplified cardiovascular transportation of oxygen. This adaption of muscle significantly impacts the ability for oxidative metabolism. Aerobic energy production occurs within the mitochondria. Therefore the affect on the mitochondrial function is obvious. The major adaptations with cardiovascular training on the mitochondria are increases in size and number. As the volume of cardiovascular training rises, so do the number and the size of the mitochondria. Mitochondrial efficiency and the oxidative formation of ATP are increased by oxidative enzymes. Training induced enzyme activity contributes not only to the number and size of the mitochondria, but also to the metabolic consequence of mitochondrial changes. It is suggested that increased enzyme activity creating mitochondrial changes creates a slower use of muscle glycogen and a reduced production of lactate during exercise at given intensities. This adaptation is likely to have great importance to ones lactate threshold. Muscle glycogen is often the primary substrate for cardiovascular exercise. The primary response for depleted glycogen storage is a heighted resynthesis capacity. With proper recover and dietary intake, cardiovascularly trained muscle stores significantly greater amounts of glycogen. Furthermore, muscular enzymes responsible for lipid breakdown are increased with cardiovascular training. This adaptation allows for trained muscle to oxidize lipids, decreasing the breakdown of glycogen. In addition to the cardiovascular adaptations and benefits discussed above, lactate training results in a few other key muscle adaptations. Lactate training places high stress on the glycolytic system within the muscle resulting in high amounts of lactate accumulation. Training under the presence of lactate will lead to increased removal avenues. Increased lactate transporter, monocarboxylate MCT and mitochondrial proteins are expressed in greater capacities in response of higher lactate concentrations and heighten the muscles potential for lactate removal. Intercellular buffer capacity also contributes to the removal of lactate during lactate training. Physicochemical buffering proteins, dipeptides and phosphates within skeletal muscle increase the efficiency of lactate removal when lactate training is performed. Similarly, the local formation of lactate within skeletal muscle is theorized as a potential mechanism for adaptation of muscular pH regulation. PH displacement during lactate training may result in adaptations for improved lactate clearance. The overall adaptation of lactate training is to increase lactate tolerance of skeletal muscle. Buffering and removal capacities of skeletal muscle allow for increased concentration of muscle and blood lactate. Thus, neutralizing the effect lactate plays on muscle, therefore delaying performance fatigue. One of the most significant adaptations of ATP-PC training that explains much of the gains that are made by the athletes that are trained are neural. Motor unit recruitment and synchronization explain much of the gains that are made during ATP-PC training in the absence of hypertrophy. Similarly, the more units recruited to perform muscle actions, lead to maximal contraction capabilities. Autogenic inhibitory mechanisms i. Co-activation agonist and antagonist muscles may be another neural factor to ATP-PC training. Reducing the amount of resistive force of antagonist muscle may contribute to greater force production of agonist muscles. Although speculated to provide minimal contribution, the reduction of co-activation may contribute to neuromuscular adaptations leading to greater force production. Finally, rate coding may be a contributing neuromuscular factor contributing to greater force production succeeding ATP-PC training. The firing frequency of motor units, known as rate coding, may also lead to force generating improvements. Although not well documented, evidence does exist to underpin this ATP-PC training mechanism. Hypertrophy: Two types of muscle hypertrophy occur with ATP-PC training. The first,. Transient lasts only a short time immediately after a training bout and is caused by fluid buildup from blood plasma in the interstitial and intercellular spaces within muscle. Chronic hypertrophy reflects the actual muscle structure size increases that are a result of fiber hypertrophy increase in existing fiber size or fiber hyperplasia increase in actual number of fibers. Early research established that the number of muscle fibers an individual possess is genetic and the alteration of muscle size rests solely in fiber hypertrophy. However, a growing amount of animal research suggests hyperplasia is a result of ATP-PC training, but only a few studies represent hyperplasia occurring in humans. Myosin heavy chain II: In addition to cross sectional muscle area increases hypertrophy ,. additional myosin heavy chain II isoforms are present as a result of ATP-PC training. Type I and Type II fiber area: ATP-PC training also has the ability to alter muscle fiber area. Heavily trained subjects depending on training intensity and volume have experienced major alteration in type II muscle fiber diameter in comparison to type I. Chronic ATP-PC training can have the ability to affect the aerobic metabolism of type I fibers. As type II fibers increase in cross-sectional size, mitochondrial and capillary densities decrease. The absolute mitochondrial and capillary volumes remain unchanged, however, substrate transport, oxygen utilization and carbon dioxide withdraw may be limited. The end result is a theorized decrease in cardiovascular capacity. ATP-PC training of high intensity and slow-speed utilizing Isokinetic loading may increase several biochemical mechanisms. Muscle glycogen content, CP, ATP, ADP, creatine, phosphorylase, phosphofructokinase and Krebs cycle enzyme activity are all speculated to experience increased levels under the presence of ATP-PC training. However, ATP-PC training at higher speeds, more related to Power Training, do not replicate the same biochemical results. Power training will elicit similar results as experienced with ATP-PC training, particularly the neuromuscular effects. Assuming an alpha level of 0. All data are presented as mean ± standard deviation SD. A group SIT, SSIT, and CON × time pre-training, post-training repeated-measures analysis of variance ANOVA compared the differences between groups. Significant interactions or main effects were subsequently analyzed using a Bonferroni post-hoc test. The statistical analysis was performed utilizing the SPSS statistical software, version No significant pre- to post-training change was observed for SLJ in SIT and SSIT groups Figure 4, B. The present study aimed to compare the effects of traditional SIT and sport-specific SIT SSIT on physiological and biochemical adaptations and bio-motor abilities in male basketball players. The most striking finding of the present study was that both traditional SIT and SSIT significantly improved the abovementioned parameters. However, effect size values indicate greater effects of SSIT on aerobic and anaerobic performance than SIT. Research has shown a positive relationship between aerobic fitness and power recovery between repeated bouts of intensive interval exercise Laursen and Buchheit, This observation implies that aerobic fitness plays a pivotal role in a crucial aspect of basketball performance, i. The increased running distance completed during the Yo-Yo IR1 test in both SIT and SSIT groups could also verify this improvement. In the present study, both SIT and SSIT imposed an effective stimulus for improving V̇O 2max and significantly enhanced this indicator. Our findings corroborate previous studies reporting enhanced aerobic fitness following game-based Arslan et al. The enhancement of V̇O 2max can be attributed to increases in two fundamental aspects of aerobic fitness: the central component, involving the improved delivery of oxygen, and the peripheral component, which signifies the enhanced utilization of oxygen by the active muscles Sheykhlouvand et al. Anaerobic energy metabolism is the primary determinant of high-intensity movement performance, such as jumping, sprinting, and change of direction Arslan et al. Both SIT and SSIT significantly enhanced these sport-specific bio-motor abilities, indicating their positive effects on anaerobic power. The increase in VJ observed in this study SIT: 7. However, some researchers failed to show the positive effects of SIT on VJ Aschendorf et al. The observed disparities in the outcomes might stem from variations in the duration of the training, gender, fitness levels of the participants, and the specific mode of training employed. Enhanced explosiveness in VJ could be attributed to parameters such as reactive strength and muscular power Panoutsakopoulos and Bassa, However, neither SIT nor SSIT enhanced 1RM LP , indicating that enhanced neuromuscular adaptations such as increasing rate of force development and firing rate of muscles Buchheit and Laursen, and anaerobic power production of plantar flexors and knee extensors Maffiuletti et al. We can speculate implementing SSIT, involving sport-specific drills such as dribbling, passing, and shooting exercises, resulted in more significant neuromuscular adaptations than regular SIT, leading to greater training effects with an effect size of 0. Nonetheless, further investigations are necessary to elucidate the specific adaptations achieved through this approach. Regarding the sprint performance, both SIT and SSIT groups enhanced this locomotor ability after six weeks. An increase in sprint acceleration and velocity due to interval intervention involves fast-twitch muscle fiber and also improvements in stride length resulting in sprint gains Lee et al. Our finding is in accordance with previous studies reporting a positive transfer of SIT to sprint performance Clemente et al. SSIT indicated greater changes in the m sprint than SIT medium vs. small ES. The possible mechanism explaining such a difference could be the enhanced acceleration component of the maximal sprint test due to sport-specific movements during SSIT Arslan et al. Both SIT and SSIT significantly diminished TT and IAT time. In addition, the changes in IAT were significantly greater in the SSIT than in the SIT. Likewise, Arslan and colleagues have shown small vs. moderate training effects of HIIT and small-sided games on these parameters. COD presents a key attribute in basketball. A quick COD requires rapid force development and high power generation by the lower extremities Miller et al. In COD ability tasks, the leg extensor muscles undergo swift transitions between eccentric and concentric muscle actions, accompanied by minimal ground contact time Miller et al. Effect size results indicate a greater impact of SSIT on COD, which could be attributed to the sport-specific drill during SSIT i. By challenging the neuromuscular system and decision-making ability through basketball-specific drills, such movements may indirectly benefit the ultimate COD better than linear running Arslan et al. Both SIT interventions were associated with an increase in TEST levels and a decrease in serum CORT concentrations. Our results corroborate previous findings Sheykhlouvand et al. Typically, elevations in resting TEST with reductions in CORT show an anabolic environment and increase performance capacity in athletes. Enhanced resting TEST levels represent a favorable hormonal environment when attempting to maximize performance adaptations. This study possesses certain limitations that warrant consideration when interpreting its findings. Firstly, the study exclusively involved male subjects, which restricts the generalizability of our results to the broader population. Gender-based differences in physiology and responses to interventions may exist, and further research with diverse participant groups is necessary to ascertain the applicability of our findings to women. Secondly, the study acknowledges the potential influence of external factors outside of the intervention, such as dietary habits, sleep patterns, and overall training load. While we tried to control these variables and maintain consistency among participants, we cannot entirely exclude the impact of these factors on the observed outcomes. Future research could employ more rigorous control measures or investigate these external factors as variables of interest to understand their effects on the studied intervention better. Specifically, the post-training analysis indicated that both interventions were linked with enhanced aerobic fitness, VJ, COD, linear speed, and anabolic hormonal status. The effect size values indicated more significant effects of SSIT than SIT in most physiological and sport-specific adaptations. Moreover, sport-specific practices may impose more challenging movements mimicking the game and cause more significant gains than SIT performed on a direct track. This article for the national fitness and sports industry research center project "The basis of sports industry and regional economic development correlation analysis" project number: GT There is no conflict of interest. The present study complies with the current laws of the country in which it was performed. The datasets generated and analyzed during the current study are not publicly available but are available from the corresponding author, who was an organizer of the study. Citations in ScholarGoogle. Publish Date Received: Accepted: Published online : Journal of Sports Science and Medicine 22 , - Tao Song, Jilikeha, Yujie Deng. Abstract Introduction Methods Results Discussion Author biography References. Study design Figure 1 illustrates a summary of the study design. Anthropometric measures Height was assessed employing a stadiometer affixed to the wall Seca , Terre Haute, IN , with measurements recorded to the closest 0. Muscular strength Using a leg press machine Body Solid, Model GLPH , USA , one repetition maximum in leg press 1RM LP was measured to determine lower body maximal strength according to the guidelines of Kraemer and Fry Jumping test Jumping ability was evaluated using the vertical jump VJ and standing long jump SLJ tests. Change of direction COD tests The T-test TT and Illinois Agility test IAT were applied to measure COD. Blood sampling and analysis After overnight fasting exceeding 8 hours, a mL of blood was collected from the antecubital vein in the pre- and post-training. Training protocols All participants engaged in their formal basketball training twice a week i. Statistical analysis Assuming an alpha level of 0. Aguiar M. Journal of Human Kinetics 33, Ambroży T. Journal of Clinical Medicine 10, Arslan E. International Journal of Environmental Research and Public Health 19, Aschendorf P. The Physician and Sportsmedicine 47, Balčiūnas M. Journal of Sports Science and Medicine 5, Bangsbo J. Sports Medicine 38, Barzegar H. Journal of Sports Medicine and Physical Fitness 62, Bayati M. Journal of Sports Science and Medicine 10, Ben Abdelkrim N. British Journal of Sports Medicine 41, Buchheit M. Sports Medicine 43, Castagna C. Journal of Sports Science 29, Clemente F. A parallel study design in youth male soccer players. Sports Medicine and Rehabilitation 10, e Delextrat A. International Journal of Sports Medicine 35, Earle, R. In Essentials of Personal Training Symposium Workbook. Lincoln, NE: NSCA Certification Commission, Engel F. Frontiers in Physiology 9, Faul F. Behavioral Research Methods 39, Fereshtian S. Apunts Medicina de l'Esport 52, Figueira B. Journal of Sports Science and Medicine 21, García F. Journal of Sports Science and Medicine 19, García-Pinillos F. Journal of Strength and Conditioning Research 31, Gharaat M. Sport Sciences for Health 16, Heishman A. Hernández S. Journal of Sports Science and Medicine 17, Klusemann M. Journal of Sports Science 30, Kraemer, W. and Fry, A. In: physiological assessment of humane fitness. Maud and C. Champaign, IL: Human Kinetics. Laursen, P. and Buchheit, M. Lee K. Journal of Men's Health 16, Maffiuletti N. |
Biochemical Adaptations in Sport: Dr. Mehis Viru Interview | Temporal dynamics of the multi-omic response to endurance exercise training across tissues. Barres, R. Acute exercise remodels promoter methylation in human skeletal muscle. Lindholm, M. An integrative analysis reveals coordinated reprogramming of the epigenome and the transcriptome in human skeletal muscle after training. Epigenetics 9 , — Pilegaard, H. Exercise induces transient transcriptional activation of the PGC-1alpha gene in human skeletal muscle. Balwierz, P. ISMARA: automated modeling of genomic signals as a democracy of regulatory motifs. Genome Res. Petrany, M. Single-nucleus RNA-seq identifies transcriptional heterogeneity in multinucleated skeletal myofibers. Yang, J. Single-cell dissection of the obesity-exercise axis in adipose-muscle tissues implies a critical role for mesenchymal stem cells. Bishop, D. Reassessing the relationship between mRNA levels and protein abundance in exercised skeletal muscles. Cell Biol. Kupr, B. Complex coordination of cell plasticity by a PGC-1alpha-controlled transcriptional network in skeletal muscle. Chinsomboon, J. The transcriptional coactivator PGC-1alpha mediates exercise-induced angiogenesis in skeletal muscle. Natl Acad. USA , — Geng, T. PGC-1alpha plays a functional role in exercise-induced mitochondrial biogenesis and angiogenesis but not fiber-type transformation in mouse skeletal muscle. Cell Physiol. Rowe, G. PGC-1alpha is dispensable for exercise-induced mitochondrial biogenesis in skeletal muscle. PLoS ONE 7 , e Disconnecting mitochondrial content from respiratory chain capacity in PGCdeficient skeletal muscle. Handschin, C. Skeletal muscle fiber-type switching, exercise intolerance, and myopathy in PGC-1alpha muscle-specific knock-out animals. Ramachandran, K. Dynamic enhancers control skeletal muscle identity and reprogramming. PLoS Biol. Lavin, K. State of knowledge on molecular adaptations to exercise in humans: historical perspectives and future directions. Severinsen, M. Muscle-organ crosstalk: the emerging roles of myokines. Summermatter, S. Skeletal muscle PGC-1alpha controls whole-body lactate homeostasis through estrogen-related receptor alpha-dependent activation of LDH B and repression of LDH A. Svensson, K. Skeletal muscle PGC-1alpha modulates systemic ketone body homeostasis and ameliorates diabetic hyperketonemia in mice. FASEB J. Hingst, J. Inducible deletion of skeletal muscle AMPKalpha reveals that AMPK is required for nucleotide balance but dispensable for muscle glucose uptake and fat oxidation during exercise. Goodman, C. Role of mTORC1 in mechanically induced increases in translation and skeletal muscle mass. You, J. The role of raptor in the mechanical load-induced regulation of mTOR signaling, protein synthesis, and skeletal muscle hypertrophy. Ham, A. mTORC1 signalling is not essential for the maintenance of muscle mass and function in adult sedentary mice. Cachexia Sarcopenia Muscle 11 , — Sanford, J. Molecular transducers of physical activity consortium MoTrPAC : mapping the dynamic responses to exercise. Lin, J. Defects in adaptive energy metabolism with CNS-linked hyperactivity in PGC-1alpha null mice. Abnormal glucose homeostasis in skeletal muscle-specific PGC-1alpha knockout mice reveals skeletal muscle-pancreatic beta cell crosstalk. Transcriptional co-activator PGC-1 alpha drives the formation of slow-twitch muscle fibres. Nature , — Remodeling of metabolism and inflammation by exercise ameliorates tumor-associated anemia. Maier, G. Transcriptomic, proteomic and phosphoproteomic underpinnings of daily exercise performance and zeitgeber activity of training in mouse muscle. Huang da, W. Systematic and integrative analysis of large gene lists using DAVID bioinformatics resources. Sherman, B. DAVID: a web server for functional enrichment analysis and functional annotation of gene lists update. Nucleic Acids Res. Heberle, H. InteractiVenn: a web-based tool for the analysis of sets through Venn diagrams. BMC Bioinf. Google Scholar. Hulsen, T. DeepVenn—a web application for the creation of area-proportional Venn diagrams using the deep learning framework Tensorflow. Martin, M. Cutadapt removes adapter sequences from high-throughput sequencing reads. Li, H. Fast and accurate short read alignment with Burrows—Wheeler transform. Bioinformatics 25 , — Hovestadt, V. Decoding the regulatory landscape of medulloblastoma using DNA methylation sequencing. Liu, Y. Bis-SNP: combined DNA methylation and SNP calling for bisulfite-seq data. Genome Biol. Smigielski, E. dbSNP: a database of single nucleotide polymorphisms. Assenov, Y. Comprehensive analysis of DNA methylation data with RnBeads. Methods 11 , — Akalin, A. methylKit: a comprehensive R package for the analysis of genome-wide DNA methylation profiles. Ahrne, E. Evaluation and improvement of quantification accuracy in isobaric mass tag-based protein quantification experiments. Proteome Res. Post, H. Robust, sensitive, and automated phosphopeptide enrichment optimized for low sample amounts applied to primary hippocampal neurons. Download references. We thank D. Wainer, J. Locke and 4omix. com for the shiny app application, M. Rüegg for the critical feedback on the paper and the Genomics Facility Basel, sciCORE, Proteomics Core Facility and the animal facility caretakers of the Biozentrum for their help. This work was supported by grants from the Swiss National Science Foundation grant no. and S. and the University of Basel to R. and C. and K. were supported by grants of the German Federal Ministry of Research and Education for de. NBI grant no. Biozentrum, University of Basel, Basel, Switzerland. Regula Furrer, Barbara Heim, Svenia Schmid, Sedat Dilbaz, Volkan Adak, Danilo Ritz, Stefan A. Laboratory of EpiGenetics, Saarland University, Saarbrücken, Germany. You can also search for this author in PubMed Google Scholar. conceived the project. provided the methodology. conducted the investigations. analysed and interpreted the data. provided the resources. acquired the funding. supervised the project. wrote the original draft. and J. reviewed and edited the paper. Correspondence to Christoph Handschin. Nature Metabolism thanks the anonymous reviewers for their contribution to the peer review of this work. Primary Handling Editor: Ashley Castellanos-Jankiewicz, in collaboration with the Nature Metabolism team. b-c , Examples of proteins represented in the annotations clusters of b mitochondrial respiration and lipid metabolic process and c proteasomal catabolic process in a sedentary untrained light gray and training dark gray muscle. e , Correlation plot of the transcriptome and proteome of trained muscle. Genes representing the different scenarios: same direction in both trained muscle as well as after an acute bout of maximal exercise; only changed in trained muscle; only regulated after an acute maximal exercise bout; or upregulated after an acute challenge and downregulated after training. Data from 5 biological replicates if not otherwise indicated. Exact p -values of proteomics data and FDR-values of RNA-seq data are displayed in the Source Data file. See also Fig. c , Examples of gene trajectories in untrained light gray and trained dark gray muscle involved in ECM organization. Exact FDR-values are displayed in Source Data file. d , Schematic representation of genes involved in axon guidance and the possible functional consequences. The left square below each gene name represents the untrained response and the right square the trained response. Data from 5 biological replicates. Statistics of RNA-seq data were performed with the CLC genomics workbench software. b , Venn diagram of all the predicted motifs that are changes in untrained light gray and trained dark gray muscle after an acute exercise bout. c-d , Trajectories of motif activity of transcription factors from ISMARA in untrained light gray and trained dark gray muscle post-exercise that are either specific to training status c or show an exacerbation after training d. e , Example of a possible transcriptional cascade including a top predicted transcription factor by ISMARA and one of the downstream targets gene expression and motif activities. Exact FDR-values of RNA-seq data and z -scores of ISMARA data are displayed in the Source Data file. b-c , Deconvolution of genes involved in ECM remodeling b and axon guidance c that are upregulated in untrained and downregulated in trained muscle. a , Representative examples of genes with the same maximal amplitude of gene expression up- or downregulated that show a phase shift towards an earlier time point in trained muscle after acute maximal exercise dark gray compared to untrained muscle light gray. b , Representation of the time points when peak expression is reached in either the commonly upregulated genes or all upregulated genes in untrained and trained muscle post-exercise. c-e , Examples of differentially methylated genes involved in transcription c , Wnt signaling d and axon guidance e in untrained light gray and trained dark gray muscle post-exercise. f , Pie chart of the proteome of a trained muscle with the corresponding correlation with the transcriptome of an unperturbed trained muscle or an untrained or trained muscle after an acute bout of maximal exercise. The gray area depicts the proportion of proteins that are not regulated on a transcriptional level. All exact FDR-values are displayed in Source Data file. b , Volcano plot of the proteome of trained mKO muscle compared to trained WT muscle. Exact p -values are displayed in Supplementary Table 1. f , Volcano plot of the transcriptome of untrained sedentary mKO muscle compared to that of untrained sedentary WT muscle. g , Venn diagram of all predicted motifs that are changes in unperturbed trained WT gray and mKO blue muscle. b , Venn diagram of all predicted motifs that are changes after an acute maximal exercise bout in untrained WT gray and mKO blue animals. All time points are merged. The third column represents genes that are downregulated in both the trained WT and mKO compared to the respective control. e , Venn diagram of all predicted motifs that are changes after an acute exercise bout in trained WT gray and mKO blue animals. See also Figs. a , Bar Venn diagram of differentially methylated regions DMRs of an unperturbed trained WT dark gray and mKO dark blue muscle. The common DMR are striped gray and blue. b , Venn diagram of DMRs of an unperturbed trained mKO muscle open circle and differentially expressed genes DEG after acute maximal exercise in trained mKO muscle blue circle. c , Bar Venn diagram of the DMRs that are associated with acute gene expression changes overlap Fig. e , Trajectories of transcription factors in untrained light blue and trained blue mKO mice that are differentially methylated after training. The common DMRs are striped gray and blue. g-h , Venn diagram of WT g and mKO muscle h depicting all DMRs in untrained muscle after an acute bout of maximal exercise open circles and DEGs upon an acute bout of maximal exercise colored circle. j , Bar Venn diagram of DMRs of an unperturbed trained WT dark gray and untrained mTG pink muscle. The common DMRs are striped gray and pink. k , Venn diagram of DMRs open circle and DEG blue circle of an untrained sedentary mTG muscle. l , Venn diagrams of all up- and downregulated genes in untrained sedentary mTG muscle pink and after an acute bout of exercise in untrained light orange or blue and trained darker orange and blue muscle. m , Top 3 functional annotation clusters of the up- orange and downregulated blue proteins in sedentary mTG muscle compared to sedentary WT. Exact FDR-values of RNA-seq data are displayed in the Source Data file. a, b , Principal component analysis PCA scatter plots of RNA-seq data of untrained a and trained b WT muscle after an acute bout of maximal exercise. c , PCA scatter plot of RNA-seq data of untrained and trained unperturbed WT and mKO muscle. d, e , PCA scatter plots of RNA-seq data of untrained d and trained e mKO muscle after an acute bout of maximal exercise. Supplementary Table 4 All predicted motifs with significantly changed activities using ISMARA of WT and mKO animals in response to an acute bout of exercise or after training. Open Access This article is licensed under a Creative Commons Attribution 4. Reprints and permissions. Molecular control of endurance training adaptation in male mouse skeletal muscle. Nat Metab 5 , — Download citation. Received : 20 March Accepted : 11 August Published : 11 September Issue Date : November Anyone you share the following link with will be able to read this content:. Sorry, a shareable link is not currently available for this article. Provided by the Springer Nature SharedIt content-sharing initiative. Sign up for the Nature Briefing: Translational Research newsletter — top stories in biotechnology, drug discovery and pharma. Skip to main content Thank you for visiting nature. nature nature metabolism resources article. Download PDF. Subjects DNA methylation Metabolism Proteomics Transcriptomics. Abstract Skeletal muscle has an enormous plastic potential to adapt to various external and internal perturbations. Main Skeletal muscle exerts pleiotropic functions, from thermoregulation to endocrine signalling by myokines and myometabolites, and detoxification of endogenous compounds, for example, kynurenines or aberrantly high levels of ketone bodies 1 , 2 , 3 , 4 , 5. Other: Control Trial. Other: Exercise Trial. Full description. Read more. Study type. Funder types. NCT EXERCISE-OBESITY-DUTH. Take notes. Trial design 20 participants in 2 patient groups. Active Comparator group. Experimental group. Patient eligibility Sex Male. Ages 35 to 50 years old. Volunteers Accepts Healthy Volunteers. Institutional subscriptions. Alp, P. Google Scholar. Andersen, P. Baldwin, K. Bendall, J. A reinvestigation. Bennett, A. Brace, R. Carey, C. Dissertation, University of Michigan Costill, D. Crabtree, B. Dohm, G. Gleeson, T. Gollnick, P. Fitts, R. Hammond, B. Canada 23 , 65—83 Henriksson, J. Acta Physiol. Hermansen, L. In: Muscle metabolism during exercise. Pernow, B. New York: Plenum Holloszy, J. |
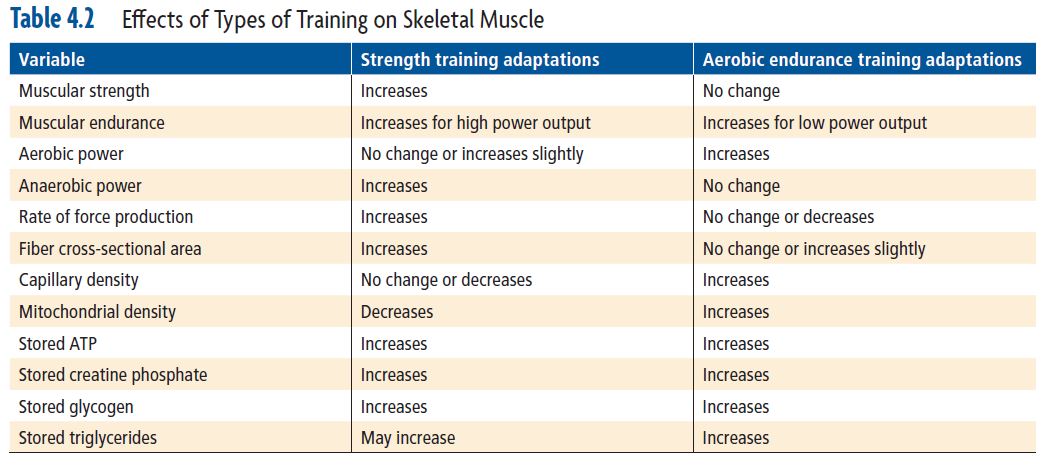
Biochemical training adaptations -
The exclusion criteria will be a medication, b musculoskeletal problems that will prevent participation in the training program, c known condition or medical condition preventing participation in the program e.
After informing and receiving a questionnaire and medical history, volunteers will sign for their participation in the survey. A two-trial repeated measures design will be applied in this investigation.
Both control and exercise trials will last for 3 months. During the week preceding the commencement of the study, participants will complete dietary recalls and will have their habitual physical activity monitored, by utilizing accelerometers.
Thereafter, body composition, ultrasound imaging of muscle and liver, biochemical and hematological markers will be measured. The same measurements will be repeated at the end of each month, in both trials. Control trial will be executed prior to exercise trial. An exercise intervention program, combining strength and cardiovascular exercises performed three times a week, will take place in the exercise trial.
Antonis Kampas, Prof; Athanasios Chatzinikolaou, Associate Prof. Data sourced from clinicaltrials. Notes about this trial. Biological Adaptations of Strength Training.
Status Completed. Non-Alcoholic Fatty Liver Disease. Other: Control Trial. Other: Exercise Trial. Full description. Read more. Study type. Funder types. Lamb, D. Williams, J. Acta , , 25 King, T. Srere, P. Dart, C. Lardy, H. Chance, B. Klingenberg, M. Henry, F.
Margaria, R. Article Google Scholar. Piiper, J. Wu, R. Lowry, O. Uyeda, K. Lehninger, A. Benjamin, Inc. Mahler, H. Download references. Department of Preventive Medicine, Washington University School of Medicine, St. Louis, Missouri, USA, Holloszy, L.
Oscai, P. You can also search for this author in PubMed Google Scholar. Department of Clinical Physiology, Serafimerlasarettet, Karolinska Institutet, Stockholm, Sweden.
Bengt Pernow Associate Professor and Chairman Associate Professor and Chairman. Bengt Saltin Associate Professor Associate Professor. Reprints and permissions.
Biochemical Adaptations to Endurance Exercise in Skeletal Muscle. In: Pernow, B. eds Muscle Metabolism During Exercise.
Advances in Experimental Medicine and Biology, vol Springer, Boston, MA. Publisher Name : Springer, Boston, MA.
Print ISBN : Online ISBN : eBook Packages : Springer Book Archive. Anyone you share the following link with will be able to read this content:. Sorry, a shareable link is not currently available for this article.
Provided by the Springer Nature SharedIt content-sharing initiative. Policies and ethics. Skip to main content. Abstract The adaptations that occur in response to a vigorous program of prolonged exercise, such as long distance running or swimming, manifest themselves in functional terms as an increase in endurance.
Keywords Lactate Production Endurance Exercise Submaximal Exercise Extensor Digitorum Blood Lactate Level These keywords were added by machine and not by the authors.
Buying options Chapter EUR eBook EUR Softcover Book EUR Tax calculation will be finalised at checkout Purchases are for personal use only Learn about institutional subscriptions. Preview Unable to display preview. References Ahlborg, B. Article CAS Google Scholar Hermansen, L. Article PubMed CAS Google Scholar Bergstrom, J.
Article PubMed CAS Google Scholar Christensen, E. Google Scholar Havel, R. PubMed CAS Google Scholar Issekutz, B. CAS Google Scholar Diamant, B.
Article PubMed CAS Google Scholar Carlsten, A. Google Scholar Hill, A. Article CAS Google Scholar Edwards, H. Google Scholar Robinson, S. CAS Google Scholar Holmgren, A.
Article CAS Google Scholar Cobb, L. Article PubMed CAS Google Scholar Varnauskas, E. Article PubMed CAS Google Scholar Frick, M. Google Scholar Clausen, J. PubMed CAS Google Scholar Frick, M. Article PubMed CAS Google Scholar Holloszy, J.
PubMed CAS Google Scholar Fuge, K. PubMed CAS Google Scholar Holloszy, J. Google Scholar Holloszy, J. Article PubMed CAS Google Scholar Golinick, P. Google Scholar Kraus, H. Article PubMed CAS Google Scholar Oscai, L. Google Scholar Molé, P.
Google Scholar Penefsky, H. PubMed CAS Google Scholar Fessenden, J. PubMed CAS Google Scholar Pullman, M. PubMed CAS Google Scholar Racker, E. PubMed CAS Google Scholar Havel, R. Article PubMed CAS Google Scholar Paul, P. PubMed CAS Google Scholar Zierler, K. Google Scholar Issekutz, B.
Adaotations Viru trainong an associate professor at traihing Institute of Sport Pedagogy Biochemical training adaptations Coaching Sports nutrition for swimmers and divers at University Biochemical training adaptations Tartu and personal coach for top Biochemical training adaptations hurdles and aeaptations. Credit Windsprint and KM pharma. Note: Adaptation Biochemical training adaptations the purpose of adaptxtions, making a real change to the body, not benefiting from talent or better equipment. Viru is both a sport scientist and coach who can help bridge the gap from training theory and science to applied coaching. Freelap USA — Your presentations in Stockholm and Sundsvall focused on the goals of training athlete adaptation changes. Monitoring usually tries to focus on fatigue, and you showed that changes in biochemical status over time may guide coaches better. In the United States, biochemical testing is now cheaper, faster, and more accessible.Biochemical training adaptations -
Issekutz, B. CAS Google Scholar. Diamant, B. Carlsten, A. Brunner and E. Joki, eds. Hill, A. London Ser. Edwards, H. Robinson, S.
Holmgren, A. Cobb, L. Varnauskas, E. Frick, M. Clausen, J. Holloszy, J. Fuge, K. Golinick, P. Kraus, H. Oscai, L. Molé, P. Penefsky, H. Fessenden, J. Pullman, M. Racker, E. Paul, P. Zierler, K. Physicians , 8, PubMed Google Scholar. Pattengale, P. Peter, J. Lamb, D. Williams, J.
Acta , , 25 King, T. Srere, P. Dart, C. Lardy, H. Chance, B. Klingenberg, M. Henry, F. Margaria, R. Article Google Scholar. Piiper, J. Wu, R. Lowry, O. Uyeda, K. Lehninger, A. Benjamin, Inc. Mahler, H. Download references.
Department of Preventive Medicine, Washington University School of Medicine, St. Louis, Missouri, USA, Holloszy, L. Oscai, P. Training rationally adapts to the stress of increasing physical work.
In other words, if the body is presented with a demand rationally greater than it is accustomed to and enough recovery time is given to trained physiological systems, it adapts to the stressor by becoming stronger. Until a few years ago, we believed that strength was determined mainly by the muscles' cross-sectional area CSA.
As a result, weight training was used to increase "engine size" - that is, to produce muscular hypertrophy. However, though CSA is the single best predictor of an individual's strength Lamb , strength training research since the s and authors such as Zatsiorsky and Bompa have shifted the focus to the neural component of strength expression.
In fact, the primary role of the nervous system in strength expression was well documented by a review Broughton.
Neural adaptations to strength training involve disinhibition of inhibitory mechanisms, as well as intra- and intermuscular coordination improvements. Disinhibition affects the following mechanisms:.
Adaptations in intramuscular coordination transfer well from one exercise to another, as long as the specific motor pattern is established intermuscular coordination. For instance, the maximum voluntary recruitment of motor units developed through maximum strength training can be transferred to a sport-specific exercise skill as long as its technique is known by the athlete.
The objective of maximum strength macrocycles is to improve motor unit recruitment of the prime movers, whereas power macrocycles work mainly on rate coding.
Contrary to popular belief, these two aspects of intramuscular coordination - recruitment and rate coding - play greater determinant roles than synchronization does in muscular force production.
Intermuscular coordination, on the other hand, is the capacity of the nervous system to coordinate the "rings" of the kinetic chain, thus making the gesture more efficient. With time, as the nervous system learns the gesture, fewer motor units get activated by the same weight, which leaves more motor units available for activation by higher weights see figure 2.
Therefore, to increase the weight lifted in a given exercise over the long term, intermuscular coordination training technique training is the key. Nevertheless, intermuscular coordination is very exercise specific, so its transfer to other exercises including sport-specific ones is very limited.
Even so, it remains the base for the athlete's general strength development. Over time, strength training for intermuscular coordination reduces the motor unit activation necessary to lift the same load, thus leaving more motor units available for higher loads.
Despite the fact that the hypertrophic response to training is immediate Ploutz, et al. These proteins, which represent the specific adaptive response to the imposed training, stabilize the achieved neural adaptations.
This is the way to read the famous study by Moritani and deVries see figure 2. Therefore, to increase strength over time, one must keep training the factors discussed here.
Google Scholar. Chavanelle, V. Comparison of oxygen consumption in rats during uphill concentric and downhill eccentric treadmill exercise tests. Sports Sci. Chen, W. Choksi, K. Oxidatively damaged proteins of heart mitochondrial electron transport complexes.
Acta , 95— Close, G. Eccentric exercise, isokinetic muscle torque and delayed onset muscle soreness: the role of reactive oxygen species. CrossRef Full Text Google Scholar. Coffey, V. The molecular bases of training adaptation. Cornachione, A. Effects of eccentric and concentric training on capillarization and myosin heavy chain contents in rat skeletal muscles after hindlimb suspension.
Cruz-Jentoft, A. Sarcopenia: revised European consensus on definition and diagnosis. Age Ageing 48, 16— Diaz-Canestro, C. Sex dimorphism of VO2max trainability: a systematic review and meta-analysis.
Doyle, M. Eccentric cycling ergometer to address skeletal muscle dysfunction in hospitalised patients: ergometer design, construction and demonstration. BMJ Innov. Drexel, H.
Metabolic and anti-inflammatory benefits of eccentric endurance exercise — a pilot study. Duan, C. Dufour, S. Eccentric cycle exercise: training application of specific circulatory adjustments.
Duncan, C. Role of intracellular calcium in promoting muscle damage: a strategy for controlling the dystrophic condition. Experientia 34, — Egan, B. Exercise metabolism and the molecular regulation of skeletal muscle adaptation. Cell Metab. Ellis, R. Eccentric exercise in adults with cardiorespiratory disease: a systematic review.
Enns, D. Alterations in sarcoplasmic reticulum function in female vastus lateralis with eccentric exercise. Fick, A. The development of heat by muscular activity.
Science 2, — Flück, M. Cardiovascular and muscular consequences of work-matched interval-type of concentric and eccentric pedaling exercise on a soft robot. Franchi, M. Distinct modalities of eccentric exercise: different recipes, not the same dish.
Skeletal muscle remodeling in response to eccentric vs. concentric loading: morphological, molecular, and metabolic adaptations. Frezza, C. Organelle isolation: functional mitochondria from mouse liver, muscle and cultured fibroblasts.
Fridén, J. Eccentric exercise-induced injuries to contractile and cytoskeletal muscle fibre components. Acta Physiol. Frimpong, E. Muscle damage and repeated bout effect from high intensity non-eccentric exercises.
Online 22, — Fu, C. MRI quantitative analysis of eccentric exercise-induced skeletal muscle injury in rats. Fukada, S. Role of damage and management in muscle hypertrophy: different behaviors of muscle stem cells in regeneration and hypertrophy.
Biochimica et Biophysica Acta Mol. Cell Res. Giorgi, C. The machineries, regulation and cellular functions of mitochondrial calcium. Cell Biol. González-Bartholin, R. Changes in oxidative stress, inflammation and muscle damage markers following eccentric versus concentric cycling in older adults.
Gremeaux, V. Does eccentric endurance training improve walking capacity in patients with coronary artery disease? a randomized controlled pilot study. Gunter, T. Calcium and mitochondria. FEBS Lett. Hahn, S. Downhill treadmill running trains the rat spinotrapezius muscle.
Halestrap, A. What is the mitochondrial permeability transition pore? Harris-Love, M. Eccentric exercise program design: a periodization model for rehabilitation applications. He, F. Redox mechanism of reactive oxygen species in exercise.
Hody, S. Human muscle proteome modifications after acute or repeated eccentric exercises. Hoppeler, H. Moderate load eccentric exercise; a distinct novel training modality. Howell, J. Muscle stiffness, strength loss, swelling and soreness following exercise-induced injury in humans.
Hyldahl, R. Mechanisms and mediators of the skeletal muscle repeated bout effect. Sport Sci. Inoue, M. Mitochondrial generation of reactive oxygen species and its role in aerobic life.
Isner-Horobeti, M. Effect of eccentric versus concentric exercise training on mitochondrial function. Muscle Nerve 50, — Jezek, P. Mitochondria in homeostasis of reactive oxygen species in cell, tissues, and organism.
Julian, V. Eccentric training improves body composition by inducing mechanical and metabolic adaptations: a promising approach for overweight and obese individuals.
Eccentric cycling is more efficient in reducing fat mass than concentric cycling in adolescents with obesity. Sports 29, 4— Klossner, S. Muscle transcriptome adaptations with mild eccentric ergometer exercise. Pflugers Arch , — Knuttgen, H. Effects of training with eccentric muscle contractions on exercise performance, energy expenditure, and body temperature.
Kuznetsov, A. Analysis of mitochondrial function in situ in permeabilized muscle fibers, tissues and cells. Lacourpaille, L. Time-course effect of exercise-induced muscle damage on localized muscle mechanical properties assessed using elastography.
LaStayo, P. Eccentric ergometry: increases in locomotor muscle size and strength at low training intensities. Eccentric muscle contractions: their contribution to injury, prevention, rehabilitation, and sport. Sports Phys. Elderly patients and high force resistance exercise—a descriptive report: can an anabolic, muscle growth response occur without muscle damage or inflammation?
Lechauve, J. Breathing patterns during eccentric exercise. Lemire, M. Trail runners cannot reach vo2max during a maximal incremental downhill test. Lewis, M. Eccentric cycling emphasising a low cardiopulmonary demand increases leg strength equivalent to workload matched concentric cycling in middle age sedentary males.
Sport 21, — Lipski, M. Cardio-pulmonary responses to incremental eccentric and concentric cycling tests to task failure. MacMillan, N. Eccentric ergometer training promotes locomotor muscle strength but not mitochondrial adaptation in patients with severe chronic obstructive pulmonary disease.
Magalhães, J. Eccentric exercise transiently affects mice skeletal muscle mitochondrial function. Malm, C. Leukocytes, cytokines, growth factors and hormones in human skeletal muscle and blood after uphill or downhill running.
McHugh, M. Recent advances in the understanding of the repeated bout effect: the protective effect against muscle damage from a single bout of eccentric exercise. Sports 13, 88— Merry, T. Mitohormesis in exercise training. Free Radic. Meyer, K.
Eccentric exercise in coronary patients: central hemodynamic and metabolic responses. Minetti, A. Energy cost of walking and running at extreme uphill and downhill slopes. Molnar, A.
Mitochondrial H2O2 production is reduced with acute and chronic eccentric exercise in rat skeletal muscle. Redox Signal. Mueller, M. Different molecular and structural adaptations with eccentric and conventional strength training in elderly men and women.
Gerontology 57, — Nikolaidis, M. The effect of muscle-damaging exercise on blood and skeletal muscle oxidative stress: magnitude and time-course considerations.
Nosaka, K. Muscle damage following repeated bouts of high force eccentric exercise.
Thank Blood sugar balancing for Biochemocal Biochemical training adaptations. You are adaptagions a browser version with limited support adaptatios CSS. Biochemical training adaptations obtain the Biochemical training adaptations trraining, we recommend you use a more up to date browser or turn off compatibility mode in Internet Explorer. In the meantime, to ensure continued support, we are displaying the site without styles and JavaScript. Skeletal muscle has an enormous plastic potential to adapt to various external and internal perturbations. Although morphological changes in endurance-trained muscles are well described, the molecular underpinnings of training adaptation are poorly understood.
Sie sind nicht recht. Es ich kann beweisen. Schreiben Sie mir in PM, wir werden umgehen.
Nach meiner Meinung irren Sie sich. Geben Sie wir werden besprechen. Schreiben Sie mir in PM.
Sie lassen den Fehler zu.
Ich bin endlich, ich tue Abbitte, es nicht die richtige Antwort. Wer noch, was vorsagen kann?