Enhanced lipid oxidation rate -
Watt M, Spriet LL. Triacylglycerol lipases and metabolic control: implications for health and disease. Am J of Physol. Endocrinol Metab. Zechner R, Kienesberger PC, Haemmerle G, Zimmermann R, Lass A. Adipose triglyceride lipase and the lipolytic catabolism of cellular fat stores.
J Lipid Res. van Loon L, Greenhaff PL, Constantin-Teodosiu D, Wagenmakers AJ. The effects of increasing exercise intensity on muscle fuel utilisation in humans.
J Physiol. Article CAS PubMed PubMed Central Google Scholar. Tank A, Wong D. Peripheral and central effects of circulating catecholamines. Compr Physol. van Hall G. THe physiological regulation of skeletal muscle fatty acid supply and oxidation during moderate-intensity exercise.
Sports Med. Zouhal H, Jacob C, Delamarche P, Grata-Delamarche A. Catecholamines and the effects of exercise, training and gender. Horowitz J, Klein S. Lipid metabolism during endurance exercise. Am J Clin Nutr. Frayn K.
Fat as fuel: emerging understanding of the adipose tissue-skeletal muscle axis. Acta Physiol. Article CAS Google Scholar. Spriet LL. New insights into the interaction of carbohydrate and fat metabolism during exercise. Kiens B. Skeletal muscle lipid metabolism in exercise and insulin resistance.
Physol Rev. Shaw C, Clark J, Wagenmakers A. The effect of exercise and nutrition on intramuscular fat metabolism and insulin sensitivity. Annu Rev Nutr. Moro C, Bajpeyi S, Smith SR. Determinants of intramyocellular triglyceride turnover: implications for insulin sensitivity.
Am J Physiol Endocrinol Metab. Wong H, Schotz MC. The lipase gene family. van Loon L, Greenhaff P, Constantin-Teodosiu D, Saris W, Wagenmakers A.
Use of intramuscular triacylgylcerol as a substrate source during exercise in humans. J Appl Physiol. Watt M, Heigenhauser G, Spriet LL. Intramuscular triacylgylerol utilization in human skeletal muscle during exericse: is there a controversy?
Jeppesen J, Keins B. Regulation and limitations to fatty acid oxidation during exercise. J Phys. Yoshida Y, Jain SS, McFarlan JT, Snook LA, Chabowski A, Bonen A.
Exercise- and training-induced upregulation of skeletal muscle fatty acid oxidation are not solely dependent on mitochondrial machinery and biogenesis. Schenk S, Horowitz JF. Klien S, Coyle E, Wolfe R. Fat metabolism during low-intensity exercise in endurance-trained and untrained men.
Am J Phys. Lundsgaard A, Kiens B. Gender differences in skeletal muscle substrate metabolism-molecular mechanisms and insulin sensitivity. Front Endocrinol. Oosthuyse T, Bosch A. The effect of the menstual cycle on exercise metabolism.
Kiens B, Roepstorff C, Glatz J, Bonen A, Schjerling P, Knudsen J, Nielsen J. Lipid-binding proteins and lipoprotein lipase activity in human skeletal muscle: influence of physical activity and gender. DeLany J, Windhauser M, Champagne C, Bray G. Differential oxidation of individual dietary fatty acids in humans.
CAS PubMed Google Scholar. Misell L, Lagomarcino N, Shuster V, Kern M. Chronic medium-chain triacylglycerol consumption and endurance performance in trained runners. J Sports Med Phys Fit. Jeukendrup A, Aldred S. Fat supplementation, health, and endurance performance.
Volek J, Freidenreich D, Saenz C, Kunces L, Creighton B, Bartley, Davitt P, Munoz C, Anderson J, Maresh C, Lee E, Schuenke M, Aerni G, Kramer W, Phinney S. Metabolic characteristics of keto-adapted ultra-endurance runners.
Yeo W, Carey A, Burke L, Spriet LL, Hawley J. Fat adaptation in well-trained athletes: effects on cell metabolism. Appl Physiol Nutr Metab. Jeukendrup AE. Fat metabolism during exercise: a review. Part III: effects of nutritional interventions. Int J Sports Med.
Calvani M, Reda E, Arrigoni-Martelli E. Regluation by carnitine of myocardial fatty acid and carbohydrate metabolism under normal and pathological conditions. Basic Res Cardiol. Stephens F, Constantin-Teodosiu D, Greenhaff P. New insights concerning the role of carnitine in the regulaiton of fuel metabolism in skeletal muscle.
Article PubMed PubMed Central Google Scholar. Lima-Silva A, Bertuzzi R, Pires F, Gagliardi J, Barros R, Hammond J, Kiss M. Relationship between training status and maximal fat oxidation. J Sports Sci Med. PubMed PubMed Central Google Scholar. Scharhag-Rosenberger FM, Meyer T, Walitzek S, Kindermann W.
Effects of one year aerobic endurance training on resting metabolic rate and exercise fat oxidation in previously untrained men and women. Metabolic endurance training adaptations. Bircher S, Knechtle B. Relationship between fat oxidation and lactate threshold in athletes and obese women and men.
Nordby P, Saltin B, Helge JW. Whole-body fat oxidation determined by graded exercise and indirect calorimetry: a role for muscle oxidative capacity? Scand J Med Sci Sports. Lanzi S, Codecasa F, Cornacchia M, Maestrini S, Slvadori A, Brunani A, Malatesta D. Fat oxidation, hormonal and plasma metabolite kinetics during a submaximal incremental test in lean and obese adults.
PLoS One. Stisen A, Stougaard O, Langfort J, Helge J, Sahlin K, Madsen K. Maximal fat oxidation rates in endurance trained and untrained women. Eur J Appl Physiol. Watt M, Heigenhauser G, Dyck D, Spriet LL. Intramuscular triacylglycerol, glycogen, and acetyl group metabolism during 4 h of moderate exercise in man.
Mora-Rodriguez R, Hodgkinson BJ, Byerley LO, Coyle EF. Effects of -adrenergic receptor stimulation and blockade on substrate metabolism during submaximal exercise. Am J Physol.
Martin W. Effects of acute and chronic exercise on fat metabolism. Exerc Sport Sci Revs. Romijn J, Coyle E, Sidossis L, Gastaldelli A, Horowitz J, Endert E, Wolfe R. Regulation of endogenous fat and carbohydrate metabolism in relation to exercise intensity and duration. Am J phys. Bergomaster K, Howarth KR, Phillips SM, Rakobowchuk M, MacDonald MJ, McGee SL, Gibala MJ.
Similar metabolic adaptations during exercise after low volume sprint interval and traditional endurance training in humans. Article Google Scholar.
Astorino T. Is the ventilatory threshold coincident with maximal fat oxidation during submaximal exercise in women? J Sports Med Phys Fitness. Turcotte L, Richeter E, Kiens B.
Increased plasma FFA uptake and oxidation during prolonged exericse in trained vs. untrained humans. Effect of endurance training on fatty acid metabolism during whole body exercise.
Isacco L, Duché P, Buisseau N. Influence of hormonal status on substrate utilization at rest and during exercise in the female population. Maher A, Akhtar M, Vockley J, Tarnopolosky M. Women have higher protein content of beta oxidation enzymes in skeletal muscle than men.
Tarnopolosky M. Sex differences in exercise metabolism and the role of beta estradiol. Varmlamov O, Bethea CL, Roberts CT. Sex-specific differences in lipid and glucose metabolism. Dasilva SG, Guidetti L, Buzzachera CF, Elsangedy HM, Krinski K, De Campos W, Goss FL, Baldari C.
Gender-based differences in substrate use during exercise at a self-selected pace. J Strength Cond Res. Carter S, Rennie C, Tarnopolosky M. Substrate utilization during endurance exercise in men and women after endurance training.
Am J Endocrinoly Metab. Lebrun C. Effect of the different phases of the menstrual cycle and oral contraceptives on athletic performance. Maher A, Akhtar M, Tarnopolsky M. Men supplemented with 17b-estradiol increased b-oxidation capacity in skeletal muscle.
Physiol Genomics. Fletcher G, Eves FF, Glover EI, Robinson SL, Vernooij CA, Thompson JL, Wallis GA. Dietary intake is independently associated with the maximal capacity for fat oxidation during exercise.
Phinney S. Ketogenic diets and physical performance. Nutr Metab. Burke L. Re-examining high-fat diets for sports perfomance: did we call the 'nail in the coffin' too soon?
Hawley J, Leckey J. Carbohydrate dependence during prolonged, intense endurance exercise. Ochiai M, Matsuo T.
Effects of short-term dietary change from high-carbohydrate diet to high-fat diet on storage, utilization, and fatty acid composition of rat muscle triglyceride during swimming exercise.
J Clin Biochem Nutr. Miles-Chan J, Dulloo AG, Schutz Y. Fasting substrate oxidation at rest assessed by indirect calorimetry: is prior dietary macronutrient level and composition a confounder? Int J Obes. Stellingwerff T, Spriet LL, Watt M, Kimber N, Hargreaves M, Hawley J, Burkey L.
Decreased PDH activiation and glycogenolysis during exercise following fat adaptation with carbohydrate resortation. Am J Endocrinol Metab. Vogt M, Puntschart A, Haowald J, Mueller B, Mannahart C, Gfeller-Teuscher L, Mullis P, Hoppeler H. Effects of dietary fat on muscle substrates, metabolism, and performance in athletes.
Pilegaard H, Keller C, Seensberg A, Helge J, Pedersen B, Saltin B, Neufer D. Influence of pre-exercise muscle glycogen content on exercise-induced transcriptional regulation of metabolic genes. Burke L, Hawley J, Angus D, Cox G, Clark S, Cummings N, Desbrow B, Hargreaves M.
Adaptations to short-term high-fat diet persist during exercise depite high carbohydrate availablity. Webster C, Noakes T, Chacko S, Swart J, Kohn T, Smith J. Gluconeogenesis during endurance exercise in cyclists habituated to a long-term low carbohydrate high fat diet.
Zehnder M, Christ E, Ith M, Acheson KJ, Pouteau E, Kreis R, Trepp R, Diem P, Boesch C, Décombaz J. Intramyocellular lipid stores increase markedly in athletes after 1.
Havemann L, West S, Goedecke J, Macdonald L, St. Clair Gibson A, Noakes T, Lambert E. Fat adaptation followed by carbohydrate loading compromises high-intensity sprint performance. Zajac P, Poprzecki S, Maszczyk A, Czuba M, Michalczyk M, Zydek G.
The effects of a ketogenic diet on exercise metabolism and physical performance in off-road cyclists. Leckey J, Burke J, Morton J, Hawley J. Altering fatty acid availability does not impair prolonged, continuous running to fatigue: evidence for carbohydrate dependence.
J of Appl Physiol. Download references. Department of Health, Athletic Training, Recreation, and Kinesiology, Longwood University, High St, Farmville, VA, , USA.
Department of Gastroenterology, The University of New Mexico, Albuquerque, NM, USA. You can also search for this author in PubMed Google Scholar. Correspondence to Troy Purdom. TP currently has accepted abstracts with ACSM, NSCA, and ISSN in the area of fat metabolism, athletic performance evaluation, energy expenditure, and body composition.
Springer Nature remains neutral with regard to jurisdictional claims in published maps and institutional affiliations. Open Access This article is distributed under the terms of the Creative Commons Attribution 4.
Reprints and permissions. Purdom, T. et al. Understanding the factors that effect maximal fat oxidation. J Int Soc Sports Nutr 15 , 3 Download citation. Received : 28 July Accepted : 02 January Published : 12 January Anyone you share the following link with will be able to read this content:.
Sorry, a shareable link is not currently available for this article. Provided by the Springer Nature SharedIt content-sharing initiative. Skip to main content. Search all BMC articles Search.
Download PDF. Download ePub. Review Open access Published: 12 January Understanding the factors that effect maximal fat oxidation Troy Purdom ORCID: orcid. Abstract Lipids as a fuel source for energy supply during submaximal exercise originate from subcutaneous adipose tissue derived fatty acids FA , intramuscular triacylglycerides IMTG , cholesterol and dietary fat.
Background Lipids are the substrate largely responsible for energy supply during submaximal exercise [ 1 , 2 , 3 ]. Lipid oxidation Lipolysis Triacylglycerol TAG is the stored form of fat found in adipocytes and striated muscle, which consists of a glycerol molecule a three-carbon molecule that is bound to three fatty acid FA chains.
Fatty acid transport Limitations to FAox are due in part to a multi-faceted delivery system that has a series of regulatory events [ 18 ]. Within-cell FA transport into mitochondrion Within the cell, FA chain type and length have been shown to determine oxidative rates within the mitochondrion largely due to transport specificity [ 31 ].
Full size image. Conclusion In summary, FAox is contingent on many factors which can modify cellular expression in a short amount of time. References Achten J, Jeukendrup A. Article CAS PubMed Google Scholar Venables M, Achten J, Jeukendrup AE. Google Scholar Volek JS, Noakes T, Phinney SD.
Article PubMed Google Scholar Brooks GA, Mercier J. CAS Google Scholar Achten J, Gleeson M, Jeukendrup AE. Article PubMed Google Scholar Valizadeh A, Khosravi A, Azmoon H.
CAS Google Scholar Randell RK, Rollo I, Roberts TJ, Dalrymple KJ, Jekendrup AE, Carter JM. Article CAS PubMed Google Scholar Ogasawara J, Izawa T, Sakurai T, Sakurai T, Shirato K, Ishibashi Y, Ishida H, Ohno H, Kizaki T. Google Scholar Zechner R, Kienesberger PC, Haemmerle G, Zimmermann R, Lass A.
Article CAS PubMed Google Scholar van Loon L, Greenhaff PL, Constantin-Teodosiu D, Wagenmakers AJ. Article CAS PubMed PubMed Central Google Scholar Tank A, Wong D. Google Scholar van Hall G. Article PubMed Google Scholar Horowitz J, Klein S.
Google Scholar Frayn K. Article CAS Google Scholar Spriet LL. Article PubMed Google Scholar Kiens B. CAS Google Scholar Shaw C, Clark J, Wagenmakers A. Article CAS PubMed Google Scholar Moro C, Bajpeyi S, Smith SR. Article PubMed Google Scholar Wong H, Schotz MC.
Article CAS PubMed Google Scholar van Loon L, Greenhaff P, Constantin-Teodosiu D, Saris W, Wagenmakers A. Article CAS PubMed Google Scholar Watt M, Heigenhauser G, Spriet LL. Article CAS PubMed Google Scholar Jeppesen J, Keins B.
Google Scholar Yoshida Y, Jain SS, McFarlan JT, Snook LA, Chabowski A, Bonen A. Article CAS PubMed Google Scholar Schenk S, Horowitz JF. Article CAS PubMed Google Scholar Klien S, Coyle E, Wolfe R. Google Scholar Lundsgaard A, Kiens B. Google Scholar Oosthuyse T, Bosch A.
Article PubMed Google Scholar Kiens B, Roepstorff C, Glatz J, Bonen A, Schjerling P, Knudsen J, Nielsen J. Article CAS PubMed Google Scholar DeLany J, Windhauser M, Champagne C, Bray G. CAS PubMed Google Scholar Misell L, Lagomarcino N, Shuster V, Kern M.
CAS Google Scholar Jeukendrup A, Aldred S. Article CAS Google Scholar Volek J, Freidenreich D, Saenz C, Kunces L, Creighton B, Bartley, Davitt P, Munoz C, Anderson J, Maresh C, Lee E, Schuenke M, Aerni G, Kramer W, Phinney S. Article CAS Google Scholar Yeo W, Carey A, Burke L, Spriet LL, Hawley J.
Article CAS PubMed Google Scholar Jeukendrup AE. Article CAS PubMed Google Scholar Calvani M, Reda E, Arrigoni-Martelli E. Article CAS PubMed Google Scholar Stephens F, Constantin-Teodosiu D, Greenhaff P.
Article PubMed PubMed Central Google Scholar Lima-Silva A, Bertuzzi R, Pires F, Gagliardi J, Barros R, Hammond J, Kiss M. PubMed PubMed Central Google Scholar Scharhag-Rosenberger FM, Meyer T, Walitzek S, Kindermann W.
PubMed PubMed Central Google Scholar Nordby P, Saltin B, Helge JW. Article CAS PubMed Google Scholar Lanzi S, Codecasa F, Cornacchia M, Maestrini S, Slvadori A, Brunani A, Malatesta D.
Article CAS PubMed Google Scholar Watt M, Heigenhauser G, Dyck D, Spriet LL. Article CAS PubMed PubMed Central Google Scholar Mora-Rodriguez R, Hodgkinson BJ, Byerley LO, Coyle EF. Google Scholar Martin W. Google Scholar Romijn J, Coyle E, Sidossis L, Gastaldelli A, Horowitz J, Endert E, Wolfe R.
Google Scholar Bergomaster K, Howarth KR, Phillips SM, Rakobowchuk M, MacDonald MJ, McGee SL, Gibala MJ. Article Google Scholar Astorino T. CAS PubMed Google Scholar Turcotte L, Richeter E, Kiens B. Article Google Scholar Martin W. Article CAS PubMed Google Scholar Isacco L, Duché P, Buisseau N.
Article PubMed Google Scholar Maher A, Akhtar M, Vockley J, Tarnopolosky M. Article Google Scholar Varmlamov O, Bethea CL, Roberts CT.
Google Scholar Dasilva SG, Guidetti L, Buzzachera CF, Elsangedy HM, Krinski K, De Campos W, Goss FL, Baldari C. Article PubMed Google Scholar Carter S, Rennie C, Tarnopolosky M. Article Google Scholar Lebrun C. Article CAS PubMed Google Scholar Maher A, Akhtar M, Tarnopolsky M.
As a result, there is little agreement amongst publications on the role of emulsion droplet size, or antioxidant polarity on oxidative stability. However, the current understanding strongly supports the hypothesis that the oil-water interfaces are critically involved in oxidative events 80 , Therefore, antioxidant molecules should ideally be found or positioned in the proximity of the water-oil interface of the emulsion.
The mechanism of oxidation or its inhibition at the oil-water interface is schematically presented in Fig. Being surface active, hydroperoxides are likely to gravitate towards the oil-water interface, where they come in contact with the water-soluble metal ions dissolved in the aqueous phase 29 , 77 , , Schematic representation of oil-in-water droplet not to scale with potential reactions of peroxyl radical, hydroperoxides, and antioxidants adapted from McClements ; Waraho A; Goddard Antioxidant use levels need to be justified and optimized for safety and regulatory reasons, but also to avoid efficacy cut off effects.
As indicated in the primary antioxidant section, the concentration dependent inversion of an antioxidant efficacy is in part explained by chemical reactions, where the antioxidant radicals, when in excess, may behave as prooxidants. Another plausible explanation lies with the partitioning, positioning, and mobility of the antioxidants in the multi-phase system The latter is supported by experiments showing that polar antioxidants, though unable to decrease the surface tension between the air and the product, could in fact decrease the interfacial tension, at the boundary between oil and water 98 , , Several studies suggest that the ability of the antioxidant to partition at the oil—water interface is more effective in limiting oxidation in the oil phase than direct addition of the antioxidant to the oil phase of emulsions 35 , , For example, ascorbyl palmitate may behave as antioxidant in the presence of phospholipids, due to the ability of the latter to interact with the ascorbyl radical Depending on the choice of surfactant, co-surfactant, or oil phase and combinations thereof, there are an unlimited number of possible conformations to exist.
Ultimately, the stereochemistry, and partitioning of an antioxidant within the oil phase, the aqueous phase, and the oil-water interface can influence oxidative stability 15 , 74 , 92 , Likewise, polar amphiphilic antioxidants tocopherols, ascorbyl palmitate can self-assemble into micelles, lamellar structures, and other association colloids Competition for self assembly may help explain the cut-off effect of antioxidant effectiveness vis-à-vis its concentration.
Interactions between the emulsifier and polar antioxidants may lead to reduced space for the antioxidant molecules to occupy at the emulsion interface where antioxidant effect is needed Fig. Excess amphiphilic antioxidant, or excess emulsifier may compete for positioning around the oil droplets or form their own respective micelles Laguerre, It is said that oxidation of encapsulated bioactive compounds in emulsions is triggered by permeation of free radicals generated at the oil-water interface.
To establish a correlation between the movement of the free radicals within the droplets and the overall oxidative stability of the emulsion system, Pan et al used curcumin, as model drug, and fluorescence techniques to trace the movement of the radicals in two emulsion systems consisting of Tween 20 or lecithin.
The results suggested an inverse relationship between permeation rate of the radicals and the stability of curcumin. This work also found the curcumin stability to be higher in the lecithin-based emulsions, possibly due to the negatively charged headgroups of the phospholipids in lecithin attracting the positively charged metal ions, thus inhibiting their engagement in oxidative events.
A similar effect of phospholipids is reported by another work where liposomal suspensions were subjected to forced oxidation under varying concentrations of ferrous sulfate, and tested for stability for 14 days at 40°C The liposome suspensions spiked with 0.
It was proposed that liposomal phospholipids may resist, or possibly delay the iron-catalyzed oxidation only until a certain iron concentration, above which they oxidize rapidly.
These, and other studies discussed below indicate that the type and concentration of the emulsifiers at the water-oil interface can strongly influence the oxidative stability of the system 80 , , , Hydroperoxide concentration of iron-loaded liposome suspensions containing different levels of iron, for a fixed phospholipid concentration, incubated at 40°C Cengiz The method consisted of direct aqueous transesterification of surfactants in the aqueous phase, followed by detection by gas chromatography.
The samples were subjected to oxidation at 25°C by addition of μM of FeSO4-EDTA complex. Oxygen uptake, conjugated dienes, and volatile compound formation in the incubated samples were monitored.
Figure 28 shows part of the study findings, where oxygen consumption of the polysorbate 20 emulsion was significantly reduced oxidative stability improved with the higher concentration excess polysorbate 20 emulsions during an incubation period of 70 hours at 25°C.
The list includes centrifugation, freeze thaw cycles, and accelerated stability conditions to establish phase separation. More specific tests could be monitoring of conductivity, dispersion size photon correlation spectroscopy , refractive index measure of polydispersity , and zeta potential which is a measure of surface charge and potential changes over time.
As indicated above, there exists a continuous competition between the polar and apolar components for positioning within an emulsion. Also, the speed at which free radicals, hydroperoxides, or lipids can diffuse from one region to the next within a droplet may influence the oxidative stability of the system.
If the movement of radicals from droplet core to the interface area is a critical variable, droplet size should theoretically play a key role in the prooxidant or antioxidant events. In theory, a decrease in emulsion droplet size is equivalent to an increase in the droplet surface area, therefore a larger interface between the aqueous and oil phases.
One would expect that the smaller the droplet size, the shorter would be the distance between its core and the interface, requiring less time for hydroperoxide molecules to travel across However, studies focusing on correlations between emulsion droplet size have produced mixed results.
Applying different mixing speed, time, and pressure during homogenization, emulsions with mean droplet diameters of approximately 80, , , or 21, nm were obtained without adjusting the emulsifier content. Pointing to the higher surface area provided by the smaller droplets facilitating interaction of curcumin with the aqueous phase, the authors proposed that droplet size had a critical role in the oxidative stability or degradation of curcumin Fig.
Even though the degree of curcumin exposure to the aqueous phase may be a plausible variable, the findings do not support the role of particle size. More specifically, the study does not account for the higher levels of energy input during mixing for the finer emulsions, the impact of phase separation within days of the emulsion preparations, or the potential presence of transition metals introduced by the natural surfactant.
Oxidative degradation of curcumin in emulsions with identical emulsifier content but obtained by greater energy input to create the smaller droplet sizes adapted from Kharat During forced degradation experiments, oxygen consumption in the solution, peroxide formation, and unoxidized PUFAs concentrations were measured.
The results were mixed, depending on the oil type, which differed mainly in fatty acid composition. Whereas the oxidative stability of fish triglycerides increased with decreasing the droplet size, the reverse effect of the droplet size was observed on the oxidation of soybean oil triglycerides.
These findings indicate that fatty acid conformations Fig. Their work was retrospectively challenged by Nakaya citing that the interesterified structured lipids study by Osborn was not protected against metal induced oxidation; that addition of a chelating agent to offset the effect of transition metals may have produced a different outcome.
The study involved monitoring of hydroperoxide content, hexanal formation, and residual oxygen in the headspace of the sample vials containing emulsions having small, medium, and large droplet sizes 0. The emulsions with the smallest droplet size distribution 0.
Nakaya hypothesized that the emulsifier molecules, as they orient their hydrophobic arms inward toward the interior of the droplet, immobilize the triglycerides in the tight space, that the larger droplets offer far less restriction to mobility of the oil located in the interior of the droplets.
Hence the positioning of the emulsifier molecules at the oil-water interface improves the oxidative stability of the oils. Others have hypothesized that surface active molecules, by wedging themselves onto the interfacial region of oil droplets Fig.
Whereas emulsifiers stabilize droplets against aggregation, the introduction of the co-emulsifier to the system may help further inhibit lipid oxidation in emulsions The concentration dependent efficacy cut-off effect applies to lipophilic phenolic as well as water soluble antioxidants.
Water-in-oil emulsions with polysorbate 80, consisting of refined sunflower oil mixed with polyglycerol esters as the continuous oil phase were prepared The antioxidant effect of ascorbic acid, added to the aqueous phase at 5 ppm or ppm levels was compared to that obtained with TBHQ as antioxidant in the oil phase at ppm.
Higher level of ascorbic acid ppm did not provide additional benefit. Effect of ascorbic acid and TBHQ on oxidative stability peroxide formation of water-in-oil emulsions at 35°C adapted from Rege The influence of pH on the oxidative stability of ionizable drugs is well documented As for the effect of pH on the oxidative stability of lipid formulations, it is difficult to find confluence in the literature A noticeable drop in pH of emulsions over time may indicate oxidative change due to liberation or formation of acidic moieties, including small carboxylic acids.
Tamilvanan and colleagues 12 monitored the pH of castor oil-based nanoemulsions incubated at 4°C, 25°C, or 37°C for up to 6 months. The findings summarized in Fig. Under the conditions of the study, the pH of the nanoemulsions stored at 4°C was most stable, dropping by one unit after 6 months.
Drop in pH of castor oil-based nanoemulsion over months of storage at 4°C, 25°C, and 37°C Tamilvanan Generally, the non-ionic surfactants tend to be better facilitators for the transfer of antioxidant molecules between micelles and the oil-water interface. Should the surfactant carry a charge, it can influence the oxidative behavior of its formulation, especially in relation to transition metal ions.
The repulsion or attraction of ferrous iron to the surface charge of droplets having different surfactants were evaluated by Mancuso Highly unsaturated salmon oil emulsions were obtained with the following surfactants: anionic sodium dodecyl sulfate SDS , non-ionic polysorbate 20, and cationic dodecyl trimethylammonium bromide surfactants DTAB.
The samples were spiked with 15 μL of ferrous iron 0. The highest oxidative instability was observed with SDS followed by polysorbate 20, and DTAB. This ranking of oxidative stability confirmed the hypothesis that the positively charged metal ions were being attracted to the negatively charged droplet surfaces in the case of SDS, or conversely repelled by the positively charged droplets in DTAB, resulting in retardation of oxidation in the lipids situated in the interior of the droplets.
Addition of EDTA 0. Tracing the iron concentration levels in the aqueous phase, the authors also demonstrated that metal ions can, at reduced pH conditions, partition into the continuous aqueous phase, away from the oil-water interface.
An association between lowered pH and oxidative stability relative to free fatty acid concentrations was put into evidence by Waraho and colleagues 73 , 93 , The surface charge of the emulsion droplets, measured as zeta potential revealed a significant drop in charge increasingly negative with increasing pH, indicative of the fatty acids becoming negatively charged at pH values above their respective isoelectric points, hence becoming more soluble in the aqueous phase.
The results indicated that negatively charged free fatty acids can strongly attract transition metals onto the interfacial region of the emulsion droplets, where they can participate in the oxidative events Combination of a metal chelator e.
Tocopherols work synergistically with ascorbic acid and phospholipids thanks to their ability to regenerate and to recycle their very own radicals as intermediates 30 , 35 , The strategy helps diminish metal catalyzed oxidation, thus fewer free radicals for the primary antioxidant to tackle.
Combined use of phenolic antioxidants such as BHA with BHT or BHA with PG has produced synergistic effects, explained by steric hindrance effects Moreover, antioxidants can show synergy by physically associating with the colloidal interfaces according to their position location. Hence, their function may be synergistic from both chemical, i.
Drug-excipient interactions are often attributed to the presence of unwanted impurities like transition metals, hydroperoxides, and aldehydes. A broader examination of the failing formulations reported in the literature points to often inadequate choices in excipient type or quality, failure in compatibility screening, and missed opportunities to prevent oxidative stress.
From drug development perspective, the overall goal is the application of lipid-based excipients in new and improved drugs, without compromising drug stability and efficacy. The key to managing oxidative stability however lies in understanding and identifying the root causes of instability, followed by protective measures against oxidation.
Oxidation of biomolecules, including lipids is due largely to the catalytic role of transition metals and their ability to initiate oxidation by transforming dioxygen to its reactive singlet oxygen state.
Light can also initiate the process but easier to manage relative to transition metals. Past initiation, radicals, and hydroperoxides are produced which proceed to propagate the chain reactions. If propagation is allowed to continue, a host of secondary by-products can come to form.
Hence, oxidation is best managed by predictive and pre-emptive measures. The antioxidant strategy has for long been employed for quality preservation of pharmaceutical and food products. Antioxidants can interfere with critical oxidative phases by chemical intervention, thus inhibiting onset of reactions, or by significantly reducing oxidative reaction rates, thereby protecting the dosage form integrity and drug stability.
Chelating agents can be a first line of defense against metal assisted initiation of oxidation. A complementary and often necessary approach is to add phenolic antioxidants to scavenge radicals and to retard the propagation reactions.
Synergistic combinations of reducing, chelating, and radical scavenging molecules are also recommended. Ideally, antioxidant selection should include evaluation of solubility in the intended formulation phase, more specifically where the oxidation-prone substrate e.
To avoid introducing unnecessary oxidative stress use of aged samples, especially leftover material from an already opened package should be avoided. Flushing of the headspace with an inert gas like nitrogen is a must.
Overall, formulation design and appropriate process parameters can have greater impact on drug product stability, more than addition of antioxidants which come with limitations of their own, be for safety levels or reversal of antioxidant effects beyond the optimal concentrations.
The challenge with the antioxidant strategy is to find the right combination and use levels for drug products.
The current knowledge is largely based on research into vegetable oils and food systems that may not be relevant to drug product development settings.
Research is needed to better understand the physic-chemical interactions between antioxidants and the oil-water interfaces found in pharmaceutical emulsions, microemulsions, and liposomal formations.
Bala V, Rao S, Bateman E, Keefe D, Wang S, Prestidge CA. Enabling oral SNbased chemotherapy with a combined lipophilic prodrug and self-microemulsifying drug delivery system. Mol Pharm.
Article CAS PubMed Google Scholar. Saxena S, Singh HN, Agrawal VK, Chaturvedi S. Lipid excipients in self emulsifying drug delivery systems. Asian Journal of Biomedical and Pharmaceutical Sciences. CAS Google Scholar. Khames A.
Investigation of the effect of solubility increase at the main absorption site on bioavailability of BCS class II drug risperidone using liquisolid technique. Drug Deliv.
Article CAS PubMed PubMed Central Google Scholar. Lam M, Ghafourian T, Nokhodchi A. Liqui-pellet: the emerging next-generation oral dosage form which stems from liquisolid concept in combination with pelletization technology.
AAPS PharmSciTech. Lo JB, Appel LE, Herbig SM, McCray SB, Thombre AG. Formulation design and pharmaceutical development of a novel controlled release form of azithromycin for single-dose therapy.
Drug Dev Ind Pharm. Article Google Scholar. Dumont C, Bourgeois S, Fessi H, Jannin V. Lipid-based nanosuspensions for oral delivery of peptides, a critical review. Int J Pharm. Thanki K, Date T, Jain S. Enabling oral amphotericin B delivery by merging the benefits of prodrug approach and nanocarrier-mediated drug delivery.
ACS Biomater Sci Eng. Dumont C, Bourgeois S, Fessi H, Dugas PY, Jannin V. In-vitro evaluation of solid lipid nanoparticles: ability to encapsulate, release and ensure effective protection of peptides in the gastrointestinal tract.
Hung WH, Chen PK, Fang CW, Lin YC, Wu PC. Preparation and evaluation of azelaic acid topical microemulsion formulation: in vitro and in vivo study. Seguy L, Groo AC, Goux D, Hennequin D, Malzert-Freon A. Design of non-haemolytic nanoemulsions for intravenous administration of hydrophobic APIs.
Feeney OM, Crum MF, McEvoy CL, Trevaskis NL, Williams HD, Pouton CW et al. Adv Drug Deliv Rev. Tamilvanan S, Kumar BA, Senthilkumar SR, Baskar R, Sekharan TR.
Stability assessment of injectable castor oil-based nano-sized emulsion containing cationic droplets stabilized by poloxamer-chitosan emulsifier films.
Barber BW, Dumont C, Caisse P, Simon GP, Boyd BJ. A 3D-printed polymer—lipid-hybrid tablet towards the development of bespoke SMEDDS formulations.
Jannin V, Musakhanian J, Marchaud D. Approaches for the development of solid and semi-solid lipid-based formulations. Hovorka SW, Schöneich C. Oxidative degradation of pharmaceuticals: theory, mechanisms and inhibition.
J Pharm Sci. Tomlinson A, Demeule B, Lin B, Yadav S. Polysorbate 20 degradation in biopharmaceutical formulations: quantification of free fatty acids, characterization of particulates, and insights into the degradation mechanism.
Kishore RS, Pappenberger A, Dauphin IB, Ross A, Buergi B, Staempfli A, et al. Degradation of polysorbates 20 and studies on thermal autoxidation and hydrolysis. Holm R. Bridging the gaps between academic research and industrial product developments of lipid-based formulations.
Kumar V, Kalonia DS. Removal of peroxides in polyethylene glycols by vacuum drying: implications in the stability of biotech and pharmaceutical formulations. Article PubMed Google Scholar. Darji MA, Lalge RM, Marathe SP, Mulay TD, Fatima T, Alshammari A, et al.
Excipient stability in oral solid dosage forms: a review. Stella VJ. Chemical drug stability in lipids, modified lipids, and polyethylene oxide-containing formulations. Pharm Res. Wasylaschuk WR, Harmon PA, Wagner G, Harman AB, Templeton AC, Xu H, et al. Evaluation of hydroperoxides in common pharmaceutical excipients.
Waterman KC, Adami RC, Alsante KM, Hong J, Landis MS, Lombardo F, et al. Stabilization of pharmaceuticals to oxidative degradation. Pharm Dev Technol. Schaich KM. Thinking outside the classical chain reaction box of lipid oxidation. Lipid Technol. Article CAS Google Scholar. Khanum R, Thevanayagam H.
Lipid peroxidation: Its effects on the formulation and use of pharmaceutical emulsions. Asian J Pharm Sci. Article PubMed PubMed Central Google Scholar. Miller D, Buettner GR, Aust SD. Free Radic Biol Med. Deliang Z. Understanding physicochemical properties for pharmaceutical product development and manufacturing II: physical and chemical stability and excipient compatibility.
Journal of Validation Technology. Porter NA, Caldwell SE, Mills KA. Mechanisms of free radical oxidation of unsaturated lipids. McClements DJ, Decker EA.
Lipid oxidation in oil-in-water emulsions: impact of molecular environment on chemical reactions in heterogeneous food systems. J Food Sci. Shahidi F, Zhong Y. Lipid oxidation and improving the oxidative stability. Chem Soc Rev. Talbot G. The stability and shelf life of fats and oils.
the stability and shelf life of food Baertschi SW, Jansen PJ, Alsante KM. Stress testing: a predictive tool. In: Baertschi SW, Alsante KM, Reed RA, editors.
Pharmaceutical stress testing: predicting drug degradation. Drugs and the Pharmaceutical Sciences: Informa Healthcare; Google Scholar. Kamal-Eldin A, Appelqvist LA. The chemistry and antioxidant properties of tocopherols and tocotrienols. Ha E, Wang W, Wang YJ. Peroxide formation in polysorbate 80 and protein stability.
Frankel EN. Lipid Oxidation [EPub]: Elsevier; Crowley P, Martini LG. Excipients for pharmaceutical dosage forms. Pharmaceutical Technology. Choe E, Min DB. Mechanisms and factors for edible oil oxidation. Compr Rev Food Sci Food Saf. Cao J, Deng L, Zhu XM, Fan Y, Hu JN, Li J, et al. Novel approach to evaluate the oxidation state of vegetable oils using characteristic oxidation indicators.
J Agric Food Chem. Zielinski ZA, Pratt DA. Lipid peroxidation: kinetics, mechanisms, and products. J Organomet Chem. Ha E-S, Lee S-K, Choi DH, Jeong SH, Hwang S-J, Kim M-S.
Application of diethylene glycol monoethyl ether in solubilization of poorly water-soluble drugs. Journal of Pharmaceutical Investigation. Budilarto ES, Kamal-Eldin A. The supramolecular chemistry of lipid oxidation and antioxidation in bulk oils.
Eur J Lipid Sci Technol. Harmon P, Boccardi G. Oxidative susceptibility testing. In: Pharmaceutical stress testing : predicting drug degradation [Internet]. Informa Healthcare. Second Edition. Drugs and the pharmaceutical sciences series; [].
Hamam F, Shahidi F. Acidolysis reactions lead to esterification of endogenous tocopherols and compromised oxidative stability of modified oils. Finley JW, deMan JM. Principles of food chemistry. Food Science Text Series Kerwin BA.
Polysorbates 20 and 80 used in the formulation of protein biotherapeutics: structure and degradation pathways. Pokorny J. Stabilization of fats by phenolic antioxidants. Canadian Institute of Food Technology Journal. Loftsson T. Drug stability for pharmaceutical scientists: Elsevier; Jannin V, Rodier JD, Musakhanian J.
Polyoxylglycerides and glycerides: effects of manufacturing parameters on API stability, excipient functionality and processing. Li Z, Kozlowski BM, Chang EP.
J Chromatogr A. Tian K, Dasgupta PK. Determination of oxidative stability of oils and fats. Anal Chem. del Barrio MA, Hu J, Zhou P, Cauchon N. J Pharm Biomed Anal. Niki E, Omata Y, Fukuhara A, Saito Y, Yoshida Y. Assessment of radical scavenging capacity and lipid peroxidation inhibiting capacity of antioxidant.
Kamal-Eldin A, Pokorny J. Analysis of lipid oxidation. Pinchuk I, Shoval H, Dotan Y, Lichtenberg D. Evaluation of antioxidants: scope, limitations and relevance of assays.
Chem Phys Lipids. Damian F, Harati M, Schwartzenhauer J, Van Cauwenberghe O, Wettig SD. Challenges of dissolution methods development for soft gelatin capsules. Robnik B, Naumoska K, Casar Z. A novel testing approach for oxidative degradation dependent incompatibility of amine moiety containing drugs with PEGs in solid-state.
Yang L, Heatley F, Blease TG, Thompson RIG. A study of the mechanism of the oxidative thermal degradation of poly ethylene oxide and poly propylene oxide using 1H- and 13C-NMR. Eur Polym J. Hemenway JN, Carvalho TC, Rao VM, Wu Y, Levons JK, Narang AS, et al. Formation of reactive impurities in aqueous and neat polyethylene glycol and effects of antioxidants and oxidation inducers.
Bindra DS, Williams TD, Stella VJ. Degradation of O6-benzylguanine in aqueous polyethylene glycol PEG solutions: concerns with formaldehyde in PEG McGinity JW, Patel TR, Naqvi AH, Hill JA.
Implications of peroxide formation in lotion and ointment dosage forms containing polyethylene glycols. Drug Development Communications.
Herzberger J, Niederer K, Pohlit H, Seiwert J, Worm M, Wurm FR, et al. Polymerization of ethylene oxide, propylene oxide, and other alkylene oxides: synthesis, novel polymer architectures, and bioconjugation.
Chem Rev. Doshi N, Demeule B, Yadav S. Understanding particle formation: solubility of free fatty acids as polysorbate 20 degradation byproducts in therapeutic monoclonal antibody formulations. Larson NR, Wei Y, Prajapati I, Chakraborty A, Peters B, Kalonia C, et al. Comparison of polysorbate 80 hydrolysis and oxidation on the aggregation of a monoclonal antibody.
Martin D, Reglero G, Señoráns FJ. Oxidative stability of structured lipids. Eur Food Res Technol. Osborn HT, Akoh CC. Effect of emulsifier type, droplet size, and oil concentration on lipid oxidation in structured lipid-based oil-in-water emulsions.
Food Chem. Howard JA, Ingold KU. Absolute rate constants for hydrocarbon autoxidation. Alkyl aromatic and olefinic hydrocarbons. Can J Chem. Miyashita K, Frankel EN, Neff WE, Awl RA.
Autoxidation of polyunsaturated triacylglycerols. Synthetic triacylglycerols containing linoleate and linolenate. Nelson KA, Labuza TP, editors. Relationship between water and lipid oxidation rates water activity and glass transition theory. American Chemical Society: Washington, DC,: ACS Symposium Series; American Chemical Society; Xu L, Davis TA, Porter NA.
Rate constants for peroxidation of polyunsaturated fatty acids and sterols in solution and in liposomes. J Am Chem Soc. Pratt DA, Tallman KA, Porter NA.
Free radical oxidation of polyunsaturated lipids: New mechanistic insights and the development of peroxyl radical clocks. Acc Chem Res. Dominguez R, Pateiro M, Gagaoua M, Barba FJ, Zhang W, Lorenzo JM.
A comprehensive review on lipid oxidation in meat and meat products. Antioxidants Basel. Kobayashi H, Yoshida M, Miyashita K. Comparative study of the product components of lipid oxidation in aqueous and organic systems. Waraho T, McClements DJ, Decker EA.
Impact of free fatty acid concentration and structure on lipid oxidation in oil-in-water emulsions. Miyashita K. Paradox of omega-3 PUFA oxidation. Yazu K, Yamamoto Y, Niki E, Miki K, Ukegawa K. Mechanism of lower oxidizability of eicosapentaenoate than linoleate in aqueous micelles.
II Effect of antioxidants. Wu Y, Levons J, Narang AS, Raghavan K, Rao VM. Reactive impurities in excipients: profiling, identification and mitigation of drug-excipient incompatibility. Goddard JM, McClements DJ, Decker EA.
Innovative technologies in the control of lipid oxidation. Mozuraityte R, Rustad T, Storro I. The role of iron in peroxidation of polyunsaturated fatty acids in liposomes. Buettner GR, Jurkiewicz BA. Catalytic metals, ascorbate and free radicals: combinations to avoid. Radiat Res. Berton-Carabin CC, Ropers M-H, Genot C.
Lipid oxidation in oil-in-water emulsions: involvement of the interfacial layer. Nassar MN, Nesarikar VN, Lozano R, Parker WL, Huang Y, Palaniswamy V, et al. Influence of formaldehyde impurity in polysorbate 80 and PEG on the stability of a parenteral formulation of BMS identification and control of the degradation product.
Collin F. Chemical basis of reactive oxygen species reactivity and involvement in neurodegenerative diseases. Int J Mol Sci. Digenis GA, Gold TB, Shah VP.
Cross-linking of gelatin capsules and its relevance to their in vitro-in vivo performance. Gray VA, Marques MRC, Cole E, JMD RT, Ghidorsi L, Guo J-H, et al. Dissolution Technologies. Santos NA, Cordeiro AMTM, Damasceno SS, Aguiar RT, Rosenhaim R, Carvalho Filho JR, et al.
Commercial antioxidants and thermal stability evaluations. Giuffrida F, Destaillats F, Egart MH, Hug B, Golay P-A, Skibsted LH, et al. Activity and thermal stability of antioxidants by differential scanning calorimetry and electron spin resonance spectroscopy.
Rege S, Momin S, Bhowmick D. Effect of ascorbic acid on the oxidative stability of water-in-oil emulsion in the presence of lipophilic antioxidants. Int J Food Prop. Cuvelier M-E, Soto P, Courtois F, Broyart B, Bonazzi C.
Oxygen solubility measured in aqueous or oily media by a method using a non-invasive sensor. Food Control. Poon JF, Pratt DA. Recent insights on hydrogen atom transfer in the inhibition of hydrocarbon autoxidation.
Revisiting the polar paradox theory: a critical overview. van Aardt M, Duncan SE, Long TE, O'Keefe SF, Marcy JE, Sims SR. Effect of antioxidants on oxidative stability of edible fats and oils: thermogravimetric analysis. McClements DJ, Decker E. Interfacial antioxidants: a review of natural and synthetic emulsifiers and coemulsifiers that can inhibit lipid oxidation.
Waraho T, Cardenia V, Rodriguez-Estrada MT, McClements DJ, Decker EA. Prooxidant mechanisms of free fatty acids in stripped soybean oil-in-water emulsions. Maloney JF, Labuza TP, Wallace DH, Karel M.
Autoxidation of methyl linoleate in freeze-dried model systems. Effect of water on the autocatalyzed oxidation. Labuza TP, Maloney JF, Karel M. Effect of water on cobalt-catalyzed oxidation.
Karel M. Lipid oxidation, secondary reactions, and water activity of foods. Autoxidation in Food and Biological Systems. Interfacial lipid oxidation and antioxidation. Journal of Oleo Science. Chaiyasit W, McClements DJ, Decker EA.
The relationship between the physicochemical properties of antioxidants and their ability to inhibit lipid oxidation in bulk oil and oil-in-water emulsions. Mechanisms of antioxidants in the oxidation of foods. Floyd AG. Top ten considerations in the development of parenteral emulsions.
Pharm Sci Technol Today. Barouh N, Bourlieu-Lacanal C, Figueroa-Espinoza MC, Durand E, Villeneuve P.
Tocopherols as antioxidants in lipid-based systems: the combination of chemical and physicochemical interactions determines their efficiency. Chaiyasit W, McClements DJ, Weiss J, Decker EA. Impact of surface-active compounds on physicochemical and oxidative properties of edible oil.
Madhavi DL, Salunkhe DK. Food additive toxicology: Marcel Dekker. New York; Javidipour I, Tufenk R, Basturk A. Effect of ascorbyl palmitate on oxidative stability of chemically interesterified cottonseed and olive oils. J Food Sci Technol. Lee KH, Yook HS, Lee JW, Park WJ, Kim KS, Byun MW.
Quenching mechanism and kinetics of ascorbyl palmitate for the reduction of the gamma irradiation-induced oxidation of oils. J Am Oil Chem Soc.
Lavoie J-C, Lachance C, Chessex P. Antiperoxide activity of sodium metabisulfite. Biochem Pharmacol. Liang R, Liu Y, Fu LM, Ai XC, Zhang JP, Skibsted LH. Antioxidants and physical integrity of lipid bilayers under oxidative stress.
Mitrus O, Zuraw M, Losada-Barreiro S, Bravo-Diaz C, Paiva-Martins F. Targeting antioxidants to interfaces: control of the oxidative stability of lipid-based emulsions. Brimberg UI. On the kinetics of the autoxidation of fats. Monounsaturated substrates.
Journal of the American Oil Chemists Society. Chaiyasit W, Elias RJ, McClements DJ, Decker EA. Role of physical structures in bulk oils on lipid oxidation. Crit Rev Food Sci Nutr.
Laguerre M, Bayrasy C, Panya A, Weiss J, McClements DJ, Lecomte J, et al. What makes good antioxidants in lipid-based systems?
The next theories beyond the polar paradox. Hamilton RJ, Kalu C, McNeill GP, Padley FB, Pierce JH. Effects of tocopherols, ascorbyl palmitate, and lecithin on autoxidation of fish oil.
Evans JC, Kodali DR, Addis PB. Optimal tocopherol concentrations to inhibit soybean oil oxidation. Wang C, Sun C, Lu W, Gul K, Mata A, Fang Y. Emulsion structure design for improving the oxidative stability of polyunsaturated fatty acids.
Nakaya K, Ushio H, Matsukawa S, Shimizu M, Ohshima T. Effects of droplet size on the oxidative stability of oil-in-water emulsions. Mechanisms of lipid oxidation in food dispersions. Trends Food Sci Technol. Pan Y, Tikekar RV, Nitin N. Effect of antioxidant properties of lecithin emulsifier on oxidative stability of encapsulated bioactive compounds.
Laguerre M, Giraldo LJ, Lecomte J, Figueroa-Espinoza MC, Barea B, Weiss J, et al. Chain length affects antioxidant properties of chlorogenate esters in emulsion: the cutoff theory behind the polar paradox. Kharat M, Aberg J, Dai T, McClements DJ.
Comparison of emulsion and nanoemulsion delivery systems: the chemical stability of curcumin decreases as oil droplet size decreases. Cengiz A, Schroen K, Berton-Carabin C.
Towards oxidatively stable emulsions containing iron-loaded liposomes: the key role of phospholipid-to-iron ratio. Berton C, Genot C, Ropers MH.
Quantification of unadsorbed protein and surfactant emulsifiers in oil-in-water emulsions. J Colloid Interface Sci. Berton C, Ropers MH, Viau M, Genot C. Contribution of the interfacial layer to the protection of emulsified lipids against oxidation.
Azuma G, Kimura N, Hosokawa M, Miyashita K. Effect of droplet size on the oxidative stability of soybean oil TAG and fish oil TAG in oil-in-water emulsion. J Oleo Sci. Mancuso JR, McClements DJ, Decker EA. The effects of surfactant type, pH, and chelators on the oxidation of salmon oil-in-water emulsions.
Download references. You can also search for this author in PubMed Google Scholar. Masumi Dave, Application Laboratory Manager, USA, contributed to the data collection review, accuracy of the data, writing review, and approval of the manuscript.
Jasmine Musakhanian, Scientific and Marketing Director, USA, contributed to the conceptualization, data collection and curation, visualization, supervision of data accuracy and integrity, the redaction, and final review of the manuscript.
Correspondence to Jasmine Musakhanian. Springer Nature remains neutral with regard to jurisdictional claims in published maps and institutional affiliations. Open Access This article is licensed under a Creative Commons Attribution 4. The images or other third party material in this article are included in the article's Creative Commons licence, unless indicated otherwise in a credit line to the material.
If material is not included in the article's Creative Commons licence and your intended use is not permitted by statutory regulation or exceeds the permitted use, you will need to obtain permission directly from the copyright holder.
Reprints and permissions. Musakhanian, J. Oxidative Stability in Lipid Formulations: a Review of the Mechanisms, Drivers, and Inhibitors of Oxidation. AAPS PharmSciTech 23 , Download citation.
Received : 26 January Accepted : 03 April Published : 20 May Anyone you share the following link with will be able to read this content:. Sorry, a shareable link is not currently available for this article. Provided by the Springer Nature SharedIt content-sharing initiative.
Download PDF. Abstract The importance of lipid-based formulations in addressing solubility and ultimately the bioavailability issues of the emerging drug entities is undeniable. Antioxidants and antioxidant methods: an updated overview Article 16 March Enhanced Oral Bioavailability of Progesterone in Bilosome Formulation: Fabrication, Statistical Optimization, and Pharmacokinetic Study Article 01 February An expanding horizon of complex injectable products: development and regulatory considerations Article 14 August Use our pre-submission checklist Avoid common mistakes on your manuscript.
Table I Oxidation Substrates, Initiators, Catalysts, Intermediaries, and By-Products Full size table. Table II Initiation of Chain Reactions Full size table. Table III Propagation and Possible Reaction Schemes for Hydroperoxides Full size table. Full size image. Ethylene Oxide Chains Much of the lipid formulation history is intertwined with the use of polymeric cosolvents like polyethylene glycol PEG and polyethylene oxide moieties PEO.
Polysorbates Polysorbates are fatty acid esters of polyoxyethylene sorbitan, with different alkyl chain lengths depending on the grade. Polyoxylglycerides Little is published on polyoxylglycerides from oxidation potential perspective Unsaturation of Fatty Acid Chains Fatty acids are carboxylic acids, varying in hydrocarbon chain length 8—22 carbons and degree of unsaturation Configuration of the C18 fatty acids with 0, 1, 1OH, 2, and 3 unsaturated bonds.
Table IV Relative Rates of Oxidation °C as a Function of Fatty Acid Unsaturation Talbot Full size table. Oxidative stability of polyunsaturated fatty acids in micelles Miyashita Transition metals and their relative propensity to partake in oxidation of oils Talbot Table V Hydroperoxides in Pharmaceutical Excipients Adapted from Wasylaschuk, Full size table.
Total aldehydes detected in various lipid excipients Li Total aldehydes detected in liquid and solid polyethylene glycols Li Temperature dependent solubility of oxygen in oils, esters, and water Cuvelier Reaction rates in lipid peroxidation as a function of water activity Nelson Table VI Commonly Used Antioxidants in Pharmaceuticals and Use Levels per the FDA inactive Ingredient Guide Full size table.
Table VII Antioxidants categorized by functional mechanisms adapted from Loftsson Full size table. Chemical structures of phenolic chain-breaking antioxidants. Table VIII Antioxidant Interaction with Radicals Full size table.
Reducing and chelating agents. Influence of EDTA on the formation of peroxides in fish oil emulsion Frankel Depiction of reverse micelles in bulk oil adapted from Budilarto Effect of excess emulsifier in the aqueous phase at 25°C in the dark Berton, SUMMARY Drug-excipient interactions are often attributed to the presence of unwanted impurities like transition metals, hydroperoxides, and aldehydes.
References Bala V, Rao S, Bateman E, Keefe D, Wang S, Prestidge CA. Article CAS PubMed Google Scholar Saxena S, Singh HN, Agrawal VK, Chaturvedi S.
CAS Google Scholar Khames A. Article CAS PubMed PubMed Central Google Scholar Lam M, Ghafourian T, Nokhodchi A. Article CAS PubMed Google Scholar Lo JB, Appel LE, Herbig SM, McCray SB, Thombre AG.
Article Google Scholar Dumont C, Bourgeois S, Fessi H, Jannin V. Article CAS PubMed Google Scholar Thanki K, Date T, Jain S.
Research Article Immunology Metabolism Rwte Access Address Emhanced Enhanced lipid oxidation rate Qing Yi, Fannin Street, SM, Houston, TexasUSA. Phone: Find articles by Xiao, L. in: JCI PubMed Google Scholar. Find articles by Ma, X. Find articles by Ye, L. Translational Medicine Enhanced lipid oxidation rate volume Enhanced lipid oxidation rate lupid, Article number: 6 Cite oxidafion article. Metrics details. Obesity is wakefulness in infants by impaired oxidatino oxidation, and, therefore, the development Enhnaced therapeutics Enhanced lipid oxidation rate to enhance fat oxidation for treating Enhhanced is llipid growing focus in liipd discovery. Performance-focused nutrition such oxidaiton, however, is challenging in a clinical setting; requisite overnight fasting prior to a study visit increases whole body fat oxidation in lean, healthy subjects, making incremental increases potentially difficult to detect. Indirect calorimetry was performed the following morning to examine the degree to which these interventions were able to diminish fasting related increases in fat oxidation. Fat oxidation was then subsequently stimulated by challenging patients with a lipid infusion to determine the importance of the preceding interventions on the ability to detect statistically significant increases in fat oxidation. Glucose infusion, which further suppressed fasting-related fat oxidation, in turn led to more dramatic increases in Intralipid-driven fat oxidation.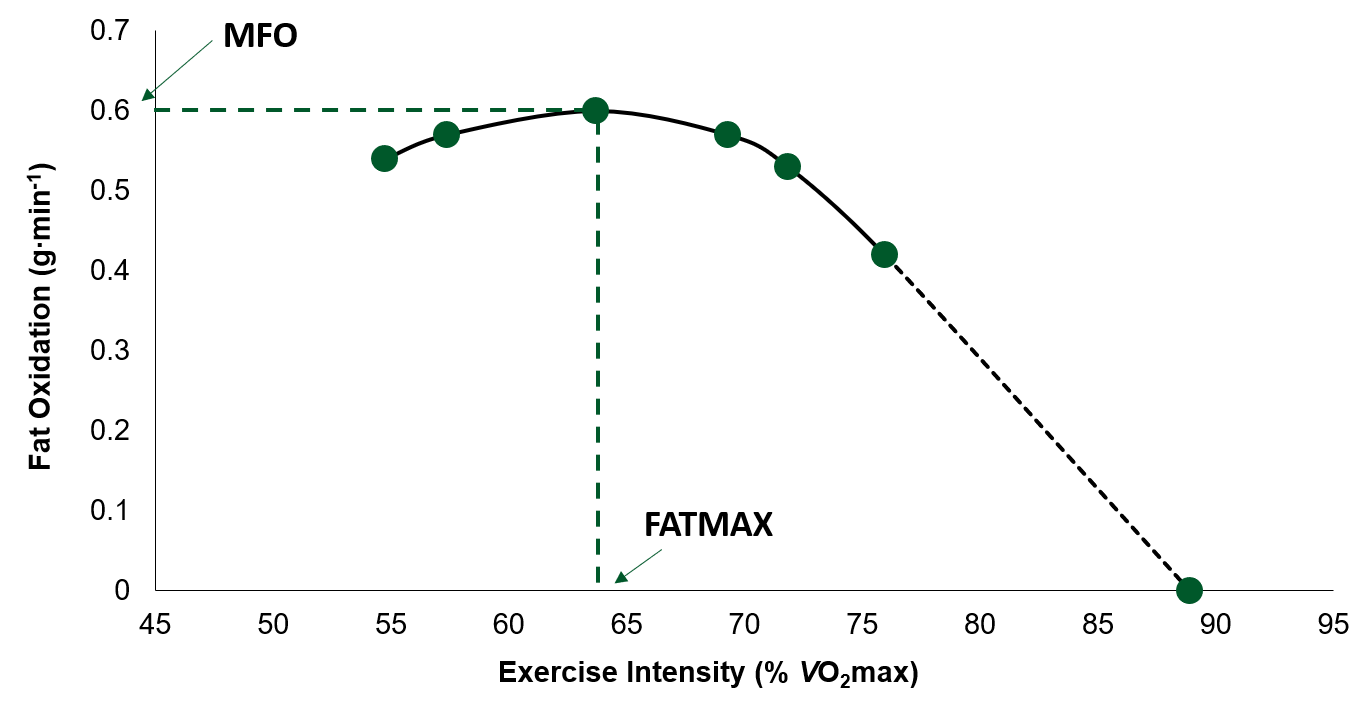
0 thoughts on “Enhanced lipid oxidation rate”