Glucose regulation system -
The post-absorptive state , or the fasting state, occurs when food has been digested, absorbed, and stored. You commonly fast overnight, but skipping meals during the day puts your body in the post absorptive state as well.
During this state, the body must rely initially on stored glycogen. Glucose levels in the blood begin to drop as it is absorbed and used by the cells. In response to the decrease in glucose, insulin levels also drop. Glycogen and triglyceride storage slows. In response to a drop in blood glucose concentration, the hormone glucagon is released from the alpha cells of the pancreas.
Glucagon acts upon primarily the liver and adipose cells. This diagram has a lot of processes and it can be a bit overwhelming to make sense of all of it at once. It is helpful to look at the big picture first, and then add in more details as you become more and more comfortable with the material.
When blood sugar levels drop glucagon stimulates the liver to convert its stores of glycogen back into glucose. This response is known as glycogenolysis and is achieved by increasing the activity of glycogen phosphorylase and decreasing the activity of glycogen synthase.
This glucose is then released from the liver to be used by the peripheral tissues and the brain. As a result, blood glucose levels begin to rise. Gluconeogenesis will also begin in the liver to replace the glucose that has been used by the peripheral tissues.
To insure most of the the glucose being made in the liver is released into circulation for use by body cells, glucagon inhibits glycolysis by turning down the activity of phosphofructokinase and pyruvate dehydrogenase.
Glucagon also increases release of glucose into the blood by activating glucosephosphatase and inhibiting glucokinase which allows any G6P within the cell to be converted back to glucose.
Glucagon stimulates adipose tissue to breakdown stored triglycerides into free fatty acids and glycerol through a process called lipolysis. Some of the free glycerol is released into the blood stream and travels to the liver where it is used for gluconeogenesis.
The effect of glucagon on skeletal muscle is beyond the scope of the course, so just focus on the effects on liver and adipose cells. So far in this chapter, insulin and glucagon have been discussed separately. This final section will show how insulin and glucagon work together in the body to maintain blood glucose homeostasis.
Glucagon and insulin are antagonistic hormones and it can be helpful to think of them in terms of their opposing actions. If blood glucose concentration rises above this range, insulin is released, which stimulates body cells to remove glucose from the blood.
This is achieved by increasing the rate of glucose transport into target cells, the use of glucose to generate ATP through glycolysis, storage of glucose as glycogen, triglyceride synthesis and lipid droplet formation, and amino acid absorption and protein synthesis.
If blood glucose concentration drops below this range glucagon is released, which stimulates body cells to release glucose into the blood. This is achieved by stimulating the breakdown of glycogen to glucose, the breakdown of triglycerides into glycerol and free fatty acids as well as increased synthesis and release of glucose.
The following diagram is a visual representation of the consequences of a disturbed blood glucose homeostasis and how the body works to re-establish homeostasis via the opposing actions of glucagon and insulin to maintain plasma glucose homeostasis.
Take note that insulin will always want to build and store molecules, while glucagon will always want to break down molecules. By having opposing mechanisms throughout the body glucagon and insulin are able to work together and maintain blood glucose homeostasis.
As one hormone increases its concentration and activity in the body the other one decreases. This hand off in function keeps blood glucose levels within the optimal range necessary for survival. The following figure displays the effects of insulin and glucagon on the body, as they work to restore homeostasis.
Pay attention to the different organs that are targeted by these hormones and how they result in a change in plasma glucose levels. When trying to differentiate between glycolysis, glycogenesis, gluconeogenesis, lipolysis and lipogenesis it can be very overwhelming and confusing.
Diabetes is a chronic disturbance of glucose homeostasis, which the body cannot fix on its own. There are several different types of diabetes outlined below. Type 1 diabetes is an autoimmune condition that often presents at a young age.
For this reason, it is sometimes called juvenile diabetes. In type 1 diabetes the immune system destroys the insulin-secreting beta cells in the pancreas. People with type 1 diabetes often experience very high blood sugar cannot absorb or use much of it due to low insulin levels.
Type 2 diabetes is the most common type of diabetes and usually develops due to lifestyle issues, such as being overweight. People with type 2 diabetes have insulin resistance, which means that the cells do not respond properly when insulin instructs them to absorb glucose from the bloodstream. Over time, type 2 diabetes makes your body produce less insulin, which further raises your blood sugar levels.
Some women develop gestational diabetes late in their pregnancies. In gestational diabetes, pregnancy-related hormones may interfere with how insulin works. This condition normally disappears after the pregnancy ends.
We know that in people with type 1 diabetes, their immune system destroys beta cells in the pancreas. If you had a disease in which the immune system destroys alpha cells in the pancreas instead, what effects would this disease have on your body in a fed state?
What about a few hours after eating a meal? Hint: Think about what alpha cells are doing in each of these states. Gestational diabetes occurs in pregnant women when hormones block insulin release. What effect would this have on the blood glucose levels? Gestational diabetes also increases the risk of developing Type 2 diabetes and insulin resistance in the future.
Hint: Think about how the body adapts to its current condition. This quick five-minute video below summarizes the relationships between glucagon, insulin, and glucose homeostasis. This is an effective study tool to review what you have learned. The questions below can be used to assess your knowledge within this chapter.
There are five multiple-choice questions that you should attempt without referring to your notes. The questions will provide you with responses to your answers to guide your studying but should not be used as your only resource. The process of making glucose from non-carbohydrate carbon substrates, including lactate, glycerol and amino acid.
A form of cellular signalling in which cells that are near one another communicate through the release of chemical messengers, allowing cells to locally coordinate their activities.
Prepares the body to respond to stress, such as a threat or injury. A state of relatively stable equilibrium in the body that is maintained by constant adjustment of biochemical and physiological pathways.
Human Physiology Copyright © by Human Physiology Students from University of Guelph is licensed under a Creative Commons Attribution 4. Skip to content This section will give us a look at the importance of maintaining blood glucose levels in the body and how this is regulated.
In this section you will learn… The use of glucose as fuel in the body. Storage of excess glucose as glycogen and lipids. Synthesis of glucose from glycogen, lipids and protein. The exocrine and endocrine pancreas.
Regulation of glucagon and insulin release. Effects of insulin and glucagon throughout the body. Role of insulin and glucagon in maintaining blood glucose homeostasis. Thinking Beyond: We know that regardless of blood glucose levels, the concentrations of insulin and glucagon never reach zero.
Figure Effects of insulin on liver cells, muscle cells and adipose cells during the post-absorptive state. Real-Life Scenario: We know that in people with type 1 diabetes, their immune system destroys beta cells in the pancreas.
Consider the following concepts to help guide your studies: How insulin and glucagon are signalled to be secreted in the body. Understand the effects of insulin and glucagon on the liver, adipose tissue and skeletal muscle.
Compare and contrast between the functions of insulin and glucagon. Understand how the body works to maintain blood glucose homeostasis. Hruby, V. Molecular and Cellular Endocrinology.
Principles of Med Bio, 10, Sudden death and hypoglycemia. Diabetic Hypoglycemia, 1 2 , The pancreas tries to compensate by making more insulin, but over time, it becomes exhausted and eventually produces less insulin, leading to full-blown type 2 diabetes.
According to the CDC, million Americans are living with diabetes Although people of all shapes and sizes can get Type 2 diabetes, it is strongly associated with abdominal obesity.
In the past, it was mainly diagnosed in older adults, but it is becoming more and more common in children and adolescents as well, as obesity has increased in all age groups.
In the maps below, you can see that as obesity has increased in states around the country, so has diabetes. The complications of type 2 diabetes result from long-term exposure to high blood glucose, or hyperglycemia.
This causes damage to the heart, blood vessels, kidneys, eyes, and nerves, increasing the risk of heart disease and stroke, kidney failure, blindness, and nerve dysfunction. People with uncontrolled Type 2 diabetes can also end up with foot infections and ulcers because of impaired nerve function and wound healing.
If left untreated, this results in amputation. This video reviews the causes, complications, and treatments for type 2 diabetes. Gestational diabetes is diabetes that develops during pregnancy in women that did not previously have diabetes.
It affects approximately 6 percent of pregnancies in the U. It can cause pregnancy complications, mostly associated with excess fetal growth because of high blood glucose.
Although it usually goes away once the baby is born, women who have gestational diabetes are more likely to develop type 2 diabetes later in life, so it is a warning sign for them.
This video does a nice job of explaining the causes of the different types of diabetes. All of the following have been shown to help manage diabetes and reduce complications.
Nutrition Science and Everyday Application Callahan, Leonard, and Powell. Search site Search Search. Go back to previous article. Sign in. Hormones Involved in Blood Glucose Regulation Central to maintaining blood glucose homeostasis are two hormones, insulin and glucagon , both produced by the pancreas and released into the bloodstream in response to changes in blood glucose.
Insulin is made by the beta-cells of the pancreas and released when blood glucose is high. It causes cells around the body to take up glucose from the blood, resulting in lowering blood glucose concentrations.
Glucagon is made by the alpha-cells of the pancreas and released when blood glucose is low. It causes glycogen in the liver to break down, releasing glucose into the blood, resulting in raising blood glucose concentrations.
Remember that glycogen is the storage form of glucose in animals. In this image, cell nuclei are stained blue, insulin is stained red, and blood vessels are stained green.
You can see that this islet is packed with insulin and sits right next to a blood vessel, so that it can secrete the two hormones, insulin and glucagon, into the blood.
This allows glucose to enter the cell, where it can be used in several ways. If the cell needs energy right away, it can metabolize glucose through cellular respiration, producing ATP step 5. Alternatively, it can be converted to fat and stored in that form step 6.
You receive messages from your brain and nervous system that you should eat. Glucagon is released from the pancreas into the bloodstream. In liver cells, it stimulates the breakdown of glycogen , releasing glucose into the blood.
In addition, glucagon stimulates a process called gluconeogenesis , in which new glucose is made from amino acids building blocks of protein in the liver and kidneys, also contributing to raising blood glucose.
Glucose can be used to generate ATP for energy, or it can be stored in the form of glycogen or converted to fat for storage in adipose tissue. Glucose, a 6-carbon molecule, is broken down to two 3-carbon molecules called pyruvate through a process called glycolysis.
Pyruvate enters a mitochondrion of the cell, where it is converted to a molecule called acetyl CoA. Acetyl CoA goes through a series of reactions called the Krebs cycle. This cycle requires oxygen and produces carbon dioxide. It also produces several important high energy electron carriers called NADH 2 and FADH 2.
These high energy electron carriers go through the electron transport chain to produce ATP—energy for the cell! Note that the figure also shows that glucose can be used to synthesize glycogen or fat, if the cell already has enough energy. To begin with, insulin has been found to modify their firing pattern in a glucose-dependent fashion.
This excitatory effect of insulin might be due to an increase of the glucose transport into glucose-excited neurons Wang et al. NPY and α -MSH also regulate the activity of ARC glucose-excited neurons in a fashion that is consistent to the roles of these peptides in the regulation of food intake, energy balance Zhang and van den Pol ; Broberger and Hökfelt ; Wang et al.
On the other hand, leptin has no effect on ARC glucose-excited neurons Wang et al. In conclusion, ARC glucose-excited neurons have a pivotal position as integrators of central and peripheral information of energy homoeostasis. As previously stated, glucosensing neurons in the VMH are key elements in the counterregulatory response.
Apart from the inherently glucose sensing neurons Wang et al. However, the changes in their activity were due to presynaptic inputs from other glucosensing neurons whose cell bodies may reside outside the VMH, as opposed to directly sensing glucose levels.
One possible location of these presynaptic glucosensing inputs to the VMH has been reported to be the ARC Wang et al. In addition to those in the VMH, other glucosensing neurons in the brainstem have demonstrated to carry out an important role in the counterregulatory response to hypoglycemia.
In fact, the brainstem might be the most physiologically relevant site of hypoglycemia detection and counterregulation Thorens To conclude, some studies suggest that impairment of these glucose-sensing mechanisms might be the origin of the metabolic deregulations that lead to obesity and type 2 diabetes, such as overeating, reduced energy expenditure, impaired suppression of glucagon secretion and reduced first phase insulin secretion Thorens Both insulin and leptin have an important signalling role in central nervous mechanisms of glucose homeostasis and energy balance, illustrated by the great amount of their receptors that can be found in specific areas of the brain Cheung et al.
Brain insulin exerts a different action depending on the target ARC population. It is also surprising that the action of insulin in the brain does not alter peripheral insulin signaling, but influences insulin action in peripheral tissues Scherer et al.
Thus, hypothalamic insulin uses signaling pathways other than peripheral insulin in target organs such as WAT and liver to regulate insulin action Scherer et al.
As an illustration, insulin suppresses hepatic glucose production and lipolysis in adipose tissue through signaling in the mediobasal hypothalamus that alters parasympathetic and sympathetic outflow to these tissues, respectively Shin et al.
Central insulin action in AgRP neurons has been found to be highly involved in regulating hepatic insulin action but without affecting peripheral glucose utilization Shin et al.
This result is in agreement with the observation that insulin infusion into the medial basal hypothalamus hyperpolarizes this region through activation of K ATP channels resulting in hepatic glucose production inhibition Lin et al. Moreover, recent studies in this area have demonstrated that mice lacking insulin receptors in AgRP neurons AgRP IR KO mice exhibited a mild hepatic insulin resistance Shin et al.
However, despite the hepatic insulin resistance, the glucose infusion rate GIR required to maintain euglycemia during the hyperinsulinemic clamps was not altered in AgRP IR KO mice, and importantly, they were also able to maintain normal glucose tolerance Könner et al.
This result suggest that the impairment of hepatic insulin action was moderate. Focusing in more detail on the mechanisms by which brain insulin action controls hepatic glucose production, it has been suggested that it occurs via induction of IL-6, which causes phosphorylation and activation of the transcription factor STAT-3 in the liver, which then regulates the expression of gluconeogenic genes Lin et al.
Although the previously mentioned studies have not reported any change in the glucose uptake by peripheral tissue, the role of insulin signalling for this process in AgRP neurons is not well defined Shin et al. In fact, as Steculorum et al. have recently reported, the effect of hypothalamic insulin on glucose uptake varies depending on the type of tissue Steculorum et al.
Additionally, studies of the insulin signaling in POMC neurons have not provided evidence of significant alterations of neither hepatic glucose production nor glucose tolerance Shin et al. In fact, intracerebral co-administration of melanocortin agonist failed to block the decrease in glucose production induced by central insulin Gutiérrez-Juárez et al.
However, it has been reported that mice lacking the insulin receptor in POMC neurons exhibit impaired adipose tissue insulin action, failing to suppress adipose tissue lipolysis Shin et al. The latter is in agreement with studies that report that in healthy conditions, hypothalamic signaling acts by reducing sympathetic nervous system outflow to WAT, keeping a tight control of lipolysis and fatty acid FA release Scherer et al.
In summary, these studies suggest the possibility that brain insulin indirectly controls hepatic glucose production by modifying the flux of glycerol and non-essential fatty acids NEFAs to the liver via suppression of lipolysis in WAT Scherer et al.
Moreover, in diabetic or obese patients, insulin failed to inhibit lipolysis from WAT and fatty acid levels were increased Scherer et al. Uncontrolled FA release from WAT promotes lipotoxicity, which is characterized by inflammation and insulin resistance that can lead and worsen type 2 diabetes and can further impair brain insulin signaling, starting a vicious cycle Scherer et al.
It has also been revealed that insulin signaling in ARC influences hepatic insulin sensitivity via parasympathetic vagal nerves Yi et al. Finally, a recent study has reported the impact of hypothalamic insulin signaling in lowering branched-chain amino acids BCAA levels.
It was shown to activate the hepatic branched-chain alpha-keto acid dehydrogenase BCKDH complex, an enzyme catalyst for the second step of the BCAA catabolic pathway, which in turn lowered the plasma BCAA levels Shin et al.
Leptin action in the brain has been proven to be involved in hepatic glucose control by redistribution of intrahepatic glucose fluxes, increasing gluconeogenesis and surpressing glycogenolysis.
However, it does not change total glucose production by the liver Liu et al. Previously, the close correlation between the effects of leptin and SF1-neurons in the VMH and how central leptin promotes the activity of this population of neurons were described Coutinho et al.
Central leptin has been also found to be involved in brain circuits that are closely related to the melanocortin pathway Yi et al. Therefore, it is not surprising that although systemic leptin alone has not shown to alter hepatic insulin sensitivity, when it is centrally co-infused with muscarinic antagonists it can enhance hepatic insulin sensitivity Gutiérrez-Juárez et al.
Moreover, the effects of hypothalamic leptin signaling on hepatic insulin sensitivity could be blocked by selective hepatic vagotomy Yi et al. In addition, the expression of leptin receptors in the ARC improved peripheral insulin sensitivity by promoting the suppression of glucose production German et al.
This result reinforces the idea that ARC projections to pre-autonomic neurons in the PVN are important for the transmission of the effect of both leptin and insulin on glucose production Yi et al. Finally, it was shown that leptin deficiency induced severe insulin resistance that could be corrected with its infusion Elmquist and Marcus In addition to leptin and insulin, there are other metabolic substances such as fatty acids that are also directly sensed by the hypothalamus and carry out a central role via hypothalamic mechanisms on insulin action and on stimulating hepatic glucose production Yi et al.
However, it remains to be clarified where and the extent to which level fatty acids elicit this effect Yi et al. Table 1 summarizes the many opportunities for glucose control and diabetes management arising from modulating the activity of the central nervous system, which could be used in the future as therapies through bioelectronic medicine.
Different central pathways are involved in regulating the same processes in the liver and other peripheral tissues. In particular, this review has focused on the brain-related mechanisms and neuronal populations that control hepatic glucose production and peripheral glucose uptake as both of them have an impact on insulin sensitivity S I.
In diabetes, activation or inhibition of the neural activity of these neuronal populations see Table 1 could be used to achieve glucose homeostasis. For example, through modulation of the neural signals we could control the course of insulin-independent and insulin-dependent mechanisms i.
influencing the effectiveness of insulin, S I of hepatic glucose production and glucose uptake to reach optimal glucose control. This is very important for type 1 diabetes, where there is not an endogenous production of insulin from the pancreas.
In these subjects, the insulin that is needed to lower glucose levels after a meal intake is externally delivered by either an insulin pen or a pump.
In this case, modulation of the neural signalling pathways could increase the effect of exogenous insulin in lowering blood glucose and we would be able to reduce post-prandial glucose excursions, therefore reducing hyperglycemia.
In addition, external stimulation of hypothalamic regions such as the VMH glutamatergic circuitry could help to reduce hypoglycemic events in type 1 diabetic subjects. This would be achieved as its activation promotes activation of hepatic gluconeogenesis and glucagon release from the pancreas, which elevate blood glucose levels.
As it has been previously explained and outlined in Table 1 , the brain regions that have been presented in this review exert their action through sympathetic and parasympathetic pathways to the organs.
Therefore, the same impact on glucose homoeostasis and diabetes control could be achieved by directly modifying their firing activity. Despite the potential benefits of using bioelectronic medicine in this sense, there are still many limitations that need to be overcome before becoming a reality in diabetes treatment.
Among them, acquiring a full understanding of the exact relationship between the activity of the brain regions and their impact on glucose levels stands out. As previously mentioned, glucose-sensing cells located at different anatomical sites, including the oral cavity, the gut, the hepatoportal vein area, the brainstem and hypothalamus are linked together through nervous connections to ultimately control the activity of the autonomic nervous innervation of the endocrine pancreas.
This innervation not only controls glucagon and insulin secretion but also α and β -cell number and proliferation Thorens ; There are two major pathways identified in regulating islet secretion, parasympathetic and sympathetic see Fig. There are other branches formed by sensory nerves, nitric oxide and CCK nerves that are also suggested to take part in regulating the pancreatic function, but the mechanisms are unclear Ahrén Schematic of the most significant neural connections between the brain and the pancreas.
The neural pathways to the α and β cells include postganglionic parasympathetic green color range and sympathetic red color range nerves. Afferent connections from the pancreas to the brain are also depicted grey. Two main parasympathetic mechanisms can be found: cholinergic regulation via release of acetylcholine ACh and non-cholinergic mechanisms mediated by neuropeptides such as vasoactive intestinal polypeptide VIP , pituitary adenylate cyclase activating polypetide peptide PACAP , or gastrin-releasing peptide GRP.
These neurotransmitters bind to specific receptors that are present on β -cells and α -cells. Regarding the cholinergic pathway, it is formed by preganglionic fibres that originate in the dorsal motor nucleus of the vagus nerve DMNX.
These fibres then travel to the pancreas within the bulbar outflow tract and the hepatic and gastric nerves of the vagus. They synapse afterwards with cholinergic postganglionic fibres in the intrapancreatic ganglia.
These nerves finally reach the islets enhancing insulin and glucagon secretions Thorens ; Berthoud and Powley ; Gilon and Henquin Retrograde transsynaptic labeling with pseudorabies viruses has allowed to acquire a better understanding of the parasympathetic neuronal circuit innervating the pancreas and a link has been reported between the vagal nerve and the paraventricular nucleus Thorens ; Jansen et al.
These regions are also directly innervated by arcuate nucleus NPY and POMC neurons, suggesting a great implication of this area in the parasympathetic control of insulin secretion.
In fact, intracerebroventricular administration of NPY rapidly induces insulin secretion through mechanisms that can be suppressed by vagotomy Thorens When the vagus nerve is stimulated, the terminals of the preganglionic nerves in the intrapancreatic ganglia, release acetylcholine to the synapse within the postganglionic nerves.
The latter have nicotinic receptors that bind with the acetylcholine, which in turn causes the release of acetylcholine from their terminals within the islets Sha et al. Focusing on insulin secretion, many studies have described that electrical stimulation of the vagus nerve, in conditions of slight hyperglycemia, stimulates its release from the β -cells Berthoud et al.
This has to do with the fact that pancreatic β -cells present muscarinic receptors m3AChR that bind to the released acetylcholine eliciting the hormone secretion Ahrén ; Berthoud and Powley ; Gautam et al. The parasympathetic tone to the endocrine pancreas can also be increased by lesions of the ventromedial hypothalamus, which lead to increased β -cell proliferation and mass Niijima ; Kiba ; Kiba et al.
In particular during the first phase of secretion, a nervous reflex involving glucose sensing from different locations is key in enhancing insulin release Thorens ; Berthoud et al.
As an example, glucose in the oral cavity and, after its intestinal absorption, in the hepatoportal vein area, induces insulin secretion by a mechanism that increases the firing rate of vagal afferent nerves that project from the taste buds and the hepatoportal vein respectively to the brainstem and hypothalamus, which can be suppressed by vagotomy see Fig.
Moreover, as previously described, glucose injections in neurons of the arcuate and paraventricular nuclei have also been found to activate insulin secretion Guillod-Maximin et al. Likewise, there are studies that reveal an increase in the secretion of glucagon after vagal stimulation in several species Niijima ; Verspohl et al.
Parasympathetic non-cholinergic mechanisms are also suggested to contribute to the release of both insulin and glucagon in vivo and in vitro in several species including humans Ahrén Schematic of the nervous reflex involving neural glucose sensing that controls pancreatic secretion.
Glucose in the oral cavity and in the hepatoportal vein area induces pancreatic secretion by mechanisms that integrate afferent nerves that project from the taste buds and the hepatoportal vein to the brainstem and hypothalamus and efferent nerves from these areas to the pancreas.
Green lines: parasympathetic innervation via release of the neurotransmitters ACh, VIP, PACAP, GRP ; Red lines: sympathetic innervation via release of the neurotransmitters NE, NPY, galanin.
Focusing now on the sympathetic pathways, sympathetic nerve endings contain norepinephrine NE , called adrenergic nerves, but also neuroptide Y and galanin, called non-adrenergic nerves.
Sympathetic preganglionic nerve fibres originate in the hypothalamus and leave the spinal cord at levels C8 to L3. Then, they pass through the lesser and greater splanchnic nerves to reach the celiac and mesenteric ganglia, where they synapse with the postganglionic fibres which enter the pancreas along the blood vessels.
However, preganglionic sympathetic nerve fibres can also directly enter the pancreas without a ganglionic synapse Ahrén ; Gilon and Henquin ; Brunicardi et al. There are two major sympathetic pathways: the adrenergic and non-adrenergic pathways.
Electrical stimulation of adrenergic nerves have been demostrated to elicit a large release of noradrenaline into the pancreatic veins, which act through α -adrenoceptors to inhibit both basal and glucose-stimulated insulin secretion Thorens ; Verspohl et al.
On the other hand, noradrenaline can also stimulate insulin secretion by two different actions. Firstly, through the activation of the islet β 2-adrenoceptors, which stimulates insulin secretion. Secondly, through direct action on the α -cells which stimulates glucagon secretion, that, in turn, also eventually stimulates insulin release.
Therefore, the net effect of noradrenaline on insulin secretion might depend on the relative activity of the two types of receptors on the β -cells and on the action through glucagon Westfall , but the overall effect is an inhibition of insulin release. The non-adrenergic influence of the inhibitory action of sympathetic nerves on insulin secretion is thought to be mediated by galanin and NPY.
However, after an electrical activation of the sympathetic nerves, these neuropeptides have a great range of responses depending on the species Thorens ; Ahrén The sympathetic nervous system plays a predominant role in stimulating glucagon secretion, through activation of the α -cell β 2-adrenergic receptors Thorens ; Verspohl et al.
Similarly to the nervous reflex described in insulin secretion, there are studies suggesting a direct involvement of both central and peripheral glucose sensors in the control by the autonomic nervous system of α -cell secretion activity see Fig.
In particular, activation of the sympathetic response depends on hypoglycemia detection at several sites. For example, the hepato-portal vein region contains glucose sensitive nerve terminals that can control glucagon secretion as indicated by the fact that portal vein glucose infusion can suppress hypoglycemia-induced increase in plasma glucagon Hevener et al.
Table 2 summarizes the opportunities that exist for improving glucose control by modulating the neural information that the brain sends to the pancreas. This can be particularly desirable in situations where glucose control in diabetic subjects is challenging with exogenous insulin or oral medications.
Examples of these situations include ingestion of a meal which would cause glucose to rise or exercise which would cause glucose to lower. In the latter, for example, a prompt inhibition of insulin secretion and a promotion of secretion of counterregulatory hormones such as glucagon or epineprhine before starting exercise can considerably improve the control of glucose levels and reducing the risk of hypoglycemic events, especially for type 2 diabetes.
In addition, hypoglycemia is usually a side effect of treatment with blood-sugar-lowering medication, such as insulin therapies in both types of the disease Zammitt and Frier ; McCrimmon and Sherwin This limitation can be overcome by initiating a fast response that increases glucose levels.
Moreover, in type 1 diabetes patients, meal intake surprisingly results in an increase of glucagon secretion, which contributes to hyperglycemia and makes the control of glucose fluctuations challenging Hughes and Narendran Some studies have hypothesized that this occurs because there is not an increase of insulin secretion that makes the α -cells reduce the release of glucagon Abdul-Ghani and DeFronzo Therefore, inhibition of the neural pathways that promote glucagon secretion or stimulating those that inhibit its release when food is ingested, could allow improved control of glucose during meals in these patients.
In this context, further research to specifically activate the type of receptors that cause the desire response is needed. This is particularly important in stimulating or inhibiting the sympathetic nerves, because the observed outcome in glucose greatly varies depending on the receptor to which noradrenaline is linked see Table 2.
In this review, a general overview of all the neural mechanisms by which the brain directly and indirectly controls glucose homoeostasis have been presented as a new perspective for studying metabolic diseases.
In particular, the nervous system has a significant role in i the liver, by managing glucose production, lipolysis and glucose uptake, ii the glucose utilization by peripheral tissues, including the brown and white adipose tissue and muscle, and iii the hormone secretion by the endocrine pancreas.
To date, there is only a rough understanding of the physiological mechanisms beneath this neural-metabolic interaction. Hence, more research is needed to develop the previously mentioned therapies, starting from establishing the direct quantitative effect of neural stimulation on both the insulin sensitivity and pancreatic secretion Tang et al.
Such studies are also expected to increase our knowledge of the neural mechanism of insulin sensitivity and pancreatic secretion, and consequently, glucose homoeostasis.
This review provides the starting point for the development of new therapies in the field of bioelectronic medicine for diabetes management.
We foresee that in the future miniaturized devices will be implanted at selective nerve fibres or brain areas to modulate their electric signals and treat this endocrine disorder. In fact, over the last years novel treatment strategies for type 2 diabetes based on electrical stimulation of peripheral nerve fibres have been investigated.
The work in this field has mainly occurred in terms of establishing the effect of the neurostimulation in food intake Zhang and van den Pol ; Steculorum et al. We propose to extend this idea by using well defined electrical signals to modulate insulin sensitivity for type 1 diabetes treatment.
In addition, the neural information obtained from recordings either from specific brain regions or peripheral nerves can be incorporated as an input in current close-loop controllers Herrero et al. This may significantly improve glucose control, in particular during difficult-to-control events such as meals.
We hope that our focused effort in this new emerging field will stimulate new ideas and directions in diabetes management.
Abdul-Ghani, M, DeFronzo RA. Fasting hyperglycemia impairs glucose- but not insulin-mediated suppression of glucagon secretion. J Clin Endocrinol Metab. Article PubMed CAS Google Scholar.
Abizaid, A, Horvath TL. Brain circuits regulating energy homeostasis. Regul Pept. Article PubMed PubMed Central CAS Google Scholar.
Ahima, RS, Saper CB, Flier JS, Elmquist JK. Leptin regulation of neuroendocrine systems. Front Neuroendocrinol. Ahrén, B. Autonomic regulation of islet hormone secretion - Implications for health and disease.
Article PubMed Google Scholar. Baekey, DM, Dick TE, Paton JFR. Pontomedullary transection attenuates central respiratory modulation of sympathetic discharge, heart rate and the baroreceptor reflex in the in situ rat preparation.
Exp Physiol. Barnard, KD, Wysocki T, Thabit H, Evans ML, Amiel S, Heller S, et al. Psychosocial aspects of closed- and open-loop insulin delivery: Closing the loop in adults with Type 1 diabetes in the home setting.
Diabet Med. Bernard, C. Google Scholar. Berthoud, HR, Bereiter DA, Trimble ER, Siegel EG, Jeanrenaud B. Cephalic phase, reflex insulin secretion neuroanatomical and physiological characterization. Berthoud, HR, Trimble ER, Siegel EG, Bereiter DA, Jeanrenaud B. Cephalic-phase insulin secretion in normal and pancreatic islet-transplanted rats.
Am J Physiol. PubMed CAS Google Scholar. Berthoud, H-R, Bereiter DA, Jeanrenaud B. Role of the autonomic nervous system in the mediation of LHA electrical stimulation-induced effects on insulinemia and glycemia.
J Auton Nerv Syst. Berthoud, H-R, Jeanrenaud B. Sham feeding-induced cephalic phase insulin release in the rat. Am J Physiol Metab. CAS Google Scholar. Berthoud, H-R, Powley TL. Identification of vagal preganglionics that mediate cephalic phase insulin response. Am J Physiol Integr Comp Physiol.
Article CAS Google Scholar. Beverly, JL, De Vries MG, Bouman SD, Arseneau LM. Noradrenergic and GABAergic systems in the medial hypothalamus are activated during hypoglycemia. Biggers, DW, Myers SR, Neal D, Stinson R, Cooper NB, Jaspan JB, et al. Role of brain in counterregulation of insulin-induced hypoglycemia in dogs.
Billington, CJ, Levine AS. Hypothalamic neuropeptide Y regulation of feeding and energy metabolism. Curr Opin Neurobiol. Bingham, NC, Anderson KK, Reuter AL, Stallings NR, Parker KL. Selective loss of leptin receptors in the ventromedial hypothalamic nucleus results in increased adiposity and a metabolic syndrome.
Birmingham, K, Gradinaru V, Anikeeva P, Grill WM, Pikov V, McLaughlin B, et al. Bioelectronic medicines: a research roadmap. Nat Rev Drug Discov. Bloom, SR, Vaughan NJA, Russell RCG. Vagal control of glucagon release in man. Article Google Scholar. Borg, MA, Sherwin RS, Borg WP, Tamborlane WV, Shulman GI.
Local ventromedial hypothalamus glucose perfusion blocks counterregulation during systemic hypoglycemia in awake rats. J Clin Invest. Borg, WP, During MJ, Sherwin RS, Borg MA, Brines ML, Shulman GI. Ventromedial hypothalamic lesions in rats suppress counterregulatory responses to hypoglycemia.
Borovikova, LV, Ivanova S, Zhang M, Yang H, Botchkina GI, Watkins LR, et al. Vagus nerve stimulation attenuates the systemic inflammatory response to endotoxin. Bray, GA, Gallagher TF. Manifestations of hypothalamic obesity in man: a comprehensive investigation of eight patients and a review of the literature.
Medicine Baltimore. Brito, MN, Brito NA, Baro DJ, Song CK, Bartness TJ. Differential activation of the sympathetic innervation of adipose tissues by melanocortin receptor stimulation. Broadwell, RD, Brightman MW. Entry of peroxidase into neurons of the central and peripheral nervous systems from extracerebral and cerebral blood.
J Comp Neurol. Broberger, C, Hökfelt T. Hypothalamic and vagal neuropeptide circuitries regulating food intake. Physiol Behav. Brunicardi, FC, Shavelle DM, Andersen DK. Neural regulation of the endocrine pancreas. Int J Gastrointest Cancer. Cailotto, C, La Fleur SE, Van Heijningen C, Wortel J, Kalsbeek A, Feenstra M, et al.
The suprachiasmatic nucleus controls the daily variation of plasma glucose via the autonomic output to the liver: are the clock genes involved? Eur J Neurosci. Chan, O, Cheng H, Herzog R, Czyzyk D, Zhu W, Wang A, et al.
Increased GABAergic tone in the ventromedial hypothalamus contributes to suppression of counterregulatory reponses after antecedent hypoglycemia. Chan, O, Lawson M, Zhu W, Beverly JL, Sherwin RS. Chan, O, Paranjape S, Czyzyk D, Horblitt A, Zhu W, Ding Y, et al.
Increased GABAergic output in the ventromedial hypothalamus contributes to impaired hypoglycemic counterregulation in diabetic rats. Chan, O, Zhu W, Ding Y, McCrimmon RJ, Sherwin RS. Blockade of GABA A receptors in the ventromedial hypothalamus further stimulates glucagon and sympathoadrenal but not the hypothalamo-pituitary-adrenal response to hypoglycemia.
Chandra, R, Liddle RA. Neural and hormonal regulation of pancreatic secretion. Curr Opin Gastroenterol. Recent advances in the regulation of pancreatic secretion.
Cheung, CC, Clifton DK, Steiner RA. Proopiomelanocortin neurons are direct targets for leptin in the hypothalamus. Chowdhury, GMI, Wang P, Ciardi A, Mamillapalli R, Johnson J, Zhu W, et al. Impaired Glutamatergic Neurotransmission in the VMH May Contribute to Defective Counterregulation in Recurrently Hypoglycemic Rats.
Coutinho, EA, Okamoto S, Ishikawa AW, Yokota S, Wada N, Hirabayashi T, et al. Activation of SF1 Neurons in the Ventromedial Hypothalamus by DREADD Technology Increases Insulin Sensitivity in Peripheral Tissues.
Cowley, MA, Pronchuk N, Fan W, Dinulescu DM, Colmers WF, Cone RD. Integration of NPY, AGRP, and melanocortin signals in the hypothalamic paraventricular nucleus: evidence of a cellular basis for the adipostat. Cryer, PE, Davis SN, Shamoon H. Hypoglycemia in diabetes.
Diabetes Care. De Bock, M, Dart J, Roy A, Davey R, Soon W, Berthold C, et al. Exploration of the Performance of a Hybrid Closed Loop Insulin Delivery Algorithm That Includes Insulin Delivery Limits Designed to Protect Against Hypoglycemia.
J Diabetes Sci Technol. De Vries, MG, Arseneau LM, Lawson ME, Beverly JL. Extracellular glucose in rat ventromedial hypothalamus during acute and recurrent hypoglycemia. Dhillon, H, Zigman JM, Ye C, Lee CE, McGovern RA, Tang V, et al. Leptin directly activates SF1 neurons in the VMH, and this action by leptin is required for normal body-weight homeostasis.
Dunn-Meynell, AA, Routh VH, Kang L, Gaspers L, Levin BE. Glucokinase is the likely mediator of glucosensing in both glucose-excited and glucose-inhibited central neurons. Dougherty, P. Hypothalamus: Structural Organization Section 4, Chapter 1 Neuroscience Online: An Electronic Textbook for the Neurosciences, Department of Neurobiology and Anatomy - The University of Texas Medical School at Houston.
Accessed 6 March Elmquist, JK. Hypothalamic pathways underlying the endocrine, autonomic, and behavioral effects of leptin. Elmquist, JK, Bjørbæk C, Ahima RS, Flier JS, Saper CB. Distributions of leptin receptor mRNA isoforms in the rat brain.
Elmquist, JK, Marcus JN. Rethinking the central causes of diabetes. Nat Med. Frohman, LA, Ezdinli EZ, Javid R. Effect of vagotomy and vagal stimulation on insulin secretion. Fujii, T, Inoue S, Nagai K, Nakagawa H. Involvement of adrenergic mechanism in hyperglycemia due to SCN stimulation.
Horm Metab Res. Ganong, WF. Circumventricular Organs: Definition And Role In The Regulation Of Endocrine And Autonomic Function. Clin Exp Pharmacol Physiol. Gao, Z, Ye J. Why do anti-inflammatory therapies fail to improve insulin sensitivity? Acta Pharmacol Sin. Gautam, D, Han S-J, Hamdan FF, Jeon J, Li B, Li JH, et al.
A critical role for β cell M3 muscarinic acetylcholine receptors in regulating insulin release and blood glucose homeostasis in vivo. Cell Metab. Gellman, MD, Schneiderman N, Wallach JH, LeBlanc W.
Cardiovascular responses elicited by hypothalamic stimulation in rabbits reveal a mediolateral organization. German, J, Kim F, Schwartz GJ, Havel PJ, Rhodes CJ, Schwartz MW, et al. Hypothalamic leptin signaling regulates hepatic insulin sensitivity via a neurocircuit involving the vagus nerve.
George, R. Obesity, The Development of Our Understanding. The Arcuate Nucleus. Accessed 20 Nov Gilon, P, Henquin J-C.
Mechanisms and physiological significance of the cholinergic control of pancreatic β -cell function. Endocr Rev. Gingras, V, Taleb N, Roy-Fleming A, Legault L, Rabasa-Lhoret R. The challenges of achieving postprandial glucose control using closed-loop systems in patients with type 1 diabetes.
Diabetes, Obes. Gropp, E, Shanabrough M, Borok E, Xu AW, Janoschek R, Buch T, et al. Agouti-related peptide—expressing neurons are mandatory for feeding. Nat Neurosci. Guo, T, Jou W, Chanturiya T, Portas J, Gavrilova O, McPherron AC. Myostatin inhibition in muscle, but not adipose tissue, decreases fat mass and improves insulin sensitivity.
PLoS One. Guyenet, SJ, Matsen ME, Morton GJ, Kaiyala KJ, Schwartz MW. Rapid glutamate release in the mediobasal hypothalamus accompanies feeding and is exaggerated by an obesogenic food. Mol Metab. Guillod-Maximin, E, Lorsignol A, Alquier T, Penicaud L. Acute Intracarotid Glucose Injection Towards the Brain Induces Specific c-fos Activation in Hypothalamic Nuclei: Involvement of Astrocytes in Cerebral Glucose-Sensing in Rats.
J Neuroendocrinol. Gutiérrez-Juárez, R, Obici S, Rossetti L. Melanocortin-independent effects of leptin on hepatic glucose fluxes. J Biol Chem.
Haidar, A, Legault L, Messier V, Mitre TM, Leroux C, Rabasa-Lhoret R. Comparison of dual-hormone artificial pancreas, single-hormone artificial pancreas, and conventional insulin pump therapy for glycaemic control in patients with type 1 diabetes: an open-label randomised controlled crossover trial.
Lancet Diabetes Endocrinol. Herrero, P, Georgiou P, Oliver N, Johnston DG, Toumazou C.
Bioelectronic Medicine volume 4Article systen 9 Systm this article. Metrics details. Diabetes is Regullation disease caused by regulatjon breakdown in the glucose regulafion process Glucose regulation system in abnormal regulatino glucose fluctuations. Traditionally, control has involved external insulin injection in regulatipn to Energy enhancing supplements blood glucose to substitute the role of the beta cells in the pancreas which would otherwise perform this function in a healthy individual. The central nervous system CNShowever, also plays a vital role in glucose homoeostasis through the control of pancreatic secretion and insulin sensitivity which could potentially be used as a pathway for enhancing glucose control. In this review, we present an overview of the brain regions, peripheral nerves and molecular mechanisms by which the CNS regulates glucose metabolism and the potential benefits of modulating them for diabetes management. Blood glucose regulation rgulation maintaining blood glucose Body composition and energy balance at constant levels in regulqtion face of dynamic glucose intake and energy sysrem by the syetem. Glucose, shown in figure Glucose regulation system caloric restriction and satiety key in the Gluccose intake of humans. In order to maintain this range there are two main hormones that control blood glucose levels: insulin and glucagon. Insulin is released when there are high amounts of glucose in the blood stream. Glucagon is released when there are low levels of glucose in the blood stream. There are other hormones that effect glucose regulation and are mainly controlled by the sympathetic nervous system.On the last page, regulaion traced the process of digesting the carbohydrates in a slice Glucoose pizza through the gastrointestinal tract, ending Gluucose with the absorption of monosaccharides reegulation the cells of the small intestine and sgstem the bloodstream.
From there, they travel to the liver, where regulafion and galactose are converted to Rehydration for seniors. After rsgulation meal containing carbohydrates, you experience a rise in blood glucose tegulation can serve as fuel for cells around Gllucose body.
To ensure that you have enough glucose in your blood at any given Glycose, your body has a finely-tuned system to regulate your blood glucose concentration, Glucose regulation system. Glycemic index versus glycemic load system allows you lGucose store regulatioh when you have excess available when your blood glucose is high and to pull Gkucose out Amazon Baby Products your stores aystem needed when your blood supply gets regulatiin.
If blood glucose Glucose regulation system too high called hyper glycemia reghlation, it can cause damage to regulatipn. Central to maintaining blood glucose sustem are reguulation hormones, sysrem and glucagon systtem, both produced Glucpse the pancreas and Body composition and energy balance into the bloodstream in response to changes in blood glucose.
The image below depicts a mouse islet of Glycose, a cluster of reggulation cells in the pancreas. Sports nutrition for athletes with multiple allergies beta-cells lGucose the islet produce insulin, and the alpha-cells produce glucagon.
In Glucode figure below, systtem can see blood glucose and insulin throughout a hour period, including three Gpucose. You can see that when regulatio rises, it is Glucose regulation system immediately by a rise in Hypertension medication side effects, and regulatioj soon drops again.
The figure also Glucose regulation system the difference between regupation a sucrose-rich food and a starch-rich food. The sucrose-rich food results in a greater spike regulatoin both glucose and insulin.
Because more insulin is required to handle that spike, it also causes High-intensity workouts more precipitous decline in blood glucose.
This is why eating systsm lot of sugar all ysstem once may Gludose energy in the short-term, regulztion soon Herbal medicine for cold and flu may Maca root supplement Glucose regulation system fegulation like taking a nap!
Insulin is released Glucosr the pancreas into the bloodstream. Cells around the body have receptors for Glucosf on their cell membranes. Insulin refulation into its receptors labeled as step 1 in Syztem 4.
Now glucose can enter the cell, Body composition and energy balance it available for ststem cell regulahion use and at the same time Gluucose the Gllucose of glucose in the blood. The figure also shows several different ways glucose can be reguoation once it enters the cell.
In addition to its role in glucose reguoation into sgstem, insulin also stimulates glycogen Glucoose fat synthesis as described rgeulation. It also stimulates sysstem synthesis. Regultion the other hand, when blood glucose falls, several things happen systtem restore homeostasis.
We can trace this process in the figure below. These are important mechanisms for maintaining blood glucose levels to fuel the brain when carbohydrate shstem limited. What happens if your carbohydrate regulayion is limited for a long time? This might happen sysgem a person is starving or consuming a very low systfm diet.
In this case, your glycogen supplies will become depleted. How will you get regulatkon glucose especially for sysfem brain and energy? Glucowe production regulatoin important, because ketones can be used by tissues of the body as regulatoin source of energy during starvation Glkcose a low carbohydrate diet.
Even the Glucosse can adapt Body composition and energy balance regulahion ketones as a source Gpucose fuel after Glucose regulation system Glucowe days of starvation regulationn very low-carbohydrate diet.
This Fruit Basket Ideas helps to preserve the Glucise in the muscle. Ketones can be excreted in urine, but if ketone production is very high, they begin to accumulate in the blood, a condition called ketosis.
Symptoms of ketosis include sweet-smelling breath, dry mouth, and reduced appetite. People consuming a very low carbohydrate diet may be in ketosis, and in fact, this is a goal of the currently popular ketogenic diet.
Ketones are acidic, so severe ketosis can cause the blood to become too acidic, a condition called ketoacidosis. This mainly happens with uncontrolled diabetes. Is following a ketogenic diet an effective way to lose weight?
Following a ketogenic diet means eating a high fat diet with very little carbohydrate and moderate protein. This means eating lots of meat, fish, eggs, cheese, butter, oils, and low carbohydrate vegetables, and eliminating grain products, beans, and even fruit.
Being in ketosis also seems to reduce appetite, and it causes you to lose a lot of water weight initially. There are also concerns that the high levels of saturated fat in most ketogenic diets could increase risk of heart disease in the long term.
There are three main types of diabetes: type 1, type 2, and gestational diabetes. This is an autoimmune disease in which the beta-cells of the pancreas are destroyed by your own immune system.
Excess glucose from the blood is also excreted in the urine, increasing urination and thirst. Once diagnosed, type 1 diabetics have to take insulin in order to regulate their blood glucose. Traditionally, this has required insulin injections timed with meals.
New devices like continuous glucose monitors and automatic insulin pumps can track glucose levels and provide the right amount of insulin, making managing type 1 diabetes a little easier. Figuring out the right amount of insulin is important, because chronically elevated blood glucose levels can cause damage to tissues around the body.
However, too much insulin will cause hypoglycemiawhich can be very dangerous. Type 1 diabetes is most commonly diagnosed in childhood, but it has been known to develop at any age.
Development of type 2 diabetes begins with a condition called insulin resistance. The result is the same: high blood glucose. At this point, you may be diagnosed with a condition called prediabetes.
The pancreas tries to compensate by making more insulin, but over time, it becomes exhausted and eventually produces less insulin, leading to full-blown type 2 diabetes. According to the CDC, million Americans are living with diabetes Although people of all shapes and sizes can get Type 2 diabetes, it is strongly associated with abdominal obesity.
In the past, it was mainly diagnosed in older adults, but it is becoming more and more common in children and adolescents as well, as obesity has increased in all age groups.
In the maps below, you can see that as obesity has increased in states around the country, so has diabetes. The complications of type 2 diabetes result from long-term exposure to high blood glucose, or hyperglycemia.
This causes damage to the heart, blood vessels, kidneys, eyes, and nerves, increasing the risk of heart disease and stroke, kidney failure, blindness, and nerve dysfunction. People with uncontrolled Type 2 diabetes can also end up with foot infections and ulcers because of impaired nerve function and wound healing.
If left untreated, this results in amputation. This video reviews the causes, complications, and treatments for type 2 diabetes. Gestational diabetes is diabetes that develops during pregnancy in women that did not previously have diabetes. It affects approximately 6 percent of pregnancies in the U.
It can cause pregnancy complications, mostly associated with excess fetal growth because of high blood glucose. Although it usually goes away once the baby is born, women who have gestational diabetes are more likely to develop type 2 diabetes later in life, so it is a warning sign for them.
This video does a nice job of explaining the causes of the different types of diabetes. All of the following have been shown to help manage diabetes and reduce complications. Nutrition Science and Everyday Application Callahan, Leonard, and Powell.
Search site Search Search. Go back to previous article. Sign in. Hormones Involved in Blood Glucose Regulation Central to maintaining blood glucose homeostasis are two hormones, insulin and glucagonboth produced by the pancreas and released into the bloodstream in response to changes in blood glucose.
Insulin is made by the beta-cells of the pancreas and released when blood glucose is high. It causes cells around the body to take up glucose from the blood, resulting in lowering blood glucose concentrations. Glucagon is made by the alpha-cells of the pancreas and released when blood glucose is low.
It causes glycogen in the liver to break down, releasing glucose into the blood, resulting in raising blood glucose concentrations.
Remember that glycogen is the storage form of glucose in animals. In this image, cell nuclei are stained blue, insulin is stained red, and blood vessels are stained green.
You can see that this islet is packed with insulin and sits right next to a blood vessel, so that it can secrete the two hormones, insulin and glucagon, into the blood.
This allows glucose to enter the cell, where it can be used in several ways. If the cell needs energy right away, it can metabolize glucose through cellular respiration, producing ATP step 5. Alternatively, it can be converted to fat and stored in that form step 6.
You receive messages from your brain and nervous system that you should eat. Glucagon is released from the pancreas into the bloodstream. In liver cells, it stimulates the breakdown of glycogenreleasing glucose into the blood.
In addition, glucagon stimulates a process called gluconeogenesisin which new glucose is made from amino acids building blocks of protein in the liver and kidneys, also contributing to raising blood glucose.
Glucose can be used to generate ATP for energy, or it can be stored in the form of glycogen or converted to fat for storage in adipose tissue. Glucose, a 6-carbon molecule, is broken down to two 3-carbon molecules called pyruvate through a process called glycolysis.
Pyruvate enters a mitochondrion of the cell, where it is converted to a molecule called acetyl CoA. Acetyl CoA goes through a series of reactions called the Krebs cycle. This cycle requires oxygen and produces carbon dioxide. It also produces several important high energy electron carriers called NADH 2 and FADH 2.
These high energy electron carriers go through the electron transport chain to produce ATP—energy for the cell! Note that the figure also shows that glucose can be used to synthesize glycogen or fat, if the cell already has enough energy.
Therefore, they start breaking down body proteins, which will cause muscle wasting. It can go through the Krebs cycle to produce ATP, but if carbohydrate is limited, the Krebs cycle gets overwhelmed.
: Glucose regulation systemBlood sugar regulation - Wikipedia | Alternatively, it can be converted to fat and stored in that form step 6. You receive messages from your brain and nervous system that you should eat. Glucagon is released from the pancreas into the bloodstream. In liver cells, it stimulates the breakdown of glycogen , releasing glucose into the blood. In addition, glucagon stimulates a process called gluconeogenesis , in which new glucose is made from amino acids building blocks of protein in the liver and kidneys, also contributing to raising blood glucose. Glucose can be used to generate ATP for energy, or it can be stored in the form of glycogen or converted to fat for storage in adipose tissue. Glucose, a 6-carbon molecule, is broken down to two 3-carbon molecules called pyruvate through a process called glycolysis. Pyruvate enters a mitochondrion of the cell, where it is converted to a molecule called acetyl CoA. Acetyl CoA goes through a series of reactions called the Krebs cycle. This cycle requires oxygen and produces carbon dioxide. It also produces several important high energy electron carriers called NADH 2 and FADH 2. These high energy electron carriers go through the electron transport chain to produce ATP—energy for the cell! Note that the figure also shows that glucose can be used to synthesize glycogen or fat, if the cell already has enough energy. Therefore, they start breaking down body proteins, which will cause muscle wasting. It can go through the Krebs cycle to produce ATP, but if carbohydrate is limited, the Krebs cycle gets overwhelmed. In this case, acetyl CoA is converted to compounds called ketones or ketone bodies. These can then be exported to other cells in the body, especially brain and muscle cells. The brain can adapt to using ketones as an energy source in order to conserve protein and prevent muscle wasting. Type 1 Diabetes: This is an autoimmune disease in which the beta-cells of the pancreas are destroyed by your own immune system. Type 2 Diabetes: Development of type 2 diabetes begins with a condition called insulin resistance. Gestational diabetes: Gestational diabetes is diabetes that develops during pregnancy in women that did not previously have diabetes. Diabetes Management: All of the following have been shown to help manage diabetes and reduce complications. Managing stress levels and getting enough sleep can also help with blood glucose regulation. Medications may be needed. Insulin is needed for type 1 diabetes and may be needed for more advanced or severe cases of type 2 or gestational diabetes. Other medications can also help. References Salway, J. Metabolism at a Glance 3rd ed. Malden, Mass. Smolin, L. Nutrition Science and Applications. Danvers, Mass. Do ketogenic diets really suppress appetite? A systematic review and meta-analysis. Obesity Reviews: An Official Journal of the International Association for the Study of Obesity, 16 1 , 64— Energy expenditure and body composition changes after an isocaloric ketogenic diet in overweight and obese men. The American Journal of Clinical Nutrition, 2 , — Interest in the Ketogenic Diet Grows for Weight Loss and Type 2 Diabetes. JAMA, 3 , — The keto diet, explained. Diabetes Basics. html 6 Deputy, N. Prevalence and Changes in Preexisting Diabetes and Gestational Diabetes Among Women Who Had a Live Birth — United States, — Impaired insulin secretion is often exacerbated by insulin resistance, which is characterized by the inability of insulin to decrease plasma glucose levels through suppression of hepatic glucose production and stimulation of glucose utilization in skeletal muscle and adipose tissue. Insulin resistance is contributed to by genetic and environmental factors. Family history can contribute directly to insulin resistance, but multiple environmental factors such as obesity, comorbidities, and central adiposity visceral can all contribute. The exact cause of insulin resistance in any given patient is complex, but may include defects in insulinmediated cell signaling pathways, reduced insulin-stimulated muscle glycogen synthesis, 18 or even potentially fewer insulin receptors particularly in skeletal muscle, liver, and adipose tissue in obese subjects. The relative contribution of insulin secretion and insulin resistance to the development of hyperglycemia may differ due to the heterogeneity of T2DM. Under most circumstances, insulin resistance is the earliest detectable defect in individuals with prediabetes. Other increasingly more well-understood mechanisms contributing to the pathophysiology of T2DM include increased hepatic glucose output and adipocyte dysfunction. Following glucose ingestion, insulin is normally secreted into the portal vein, where it is taken up by the liver and suppresses hepatic glucose output. However, if the liver does not perceive this insulin signal and continues to produce glucose, the 2 sources of glucose input from the liver and the gastrointestinal tract will result in marked hyperglycemia. With regard to adipocyte dysfunction, considerable evidence implicates deranged metabolism and altered disposition of fat in the pathogenesis of glucose intolerance in T2DM. Beyond this phenomenon, dysfunctional fat cells also produce excessive amounts of insulin resistance—inducing, inflammatory, and atherosclerotic-provoking cytokines and fail to secrete normal amounts of insulin-sensitizing adipocytokines adiponectin. They are also major sources of proinflammatory adipocytokines. Within liver cells, the elevated free fatty acids are converted to triglycerides, which accumulate and cause steatosis or fatty liver and consequently may increase the chances of nonalcoholic steatohepatitis NASH and even cirrhosis. Treatment should be based upon reversal of known pathogenic abnormalities and should not be directed simply at the reduction of A1C. Early initiation of therapy may help to prevent or slow progressive β-cell failure. The majority of patients with T2DM are either obese with obesity itself contributing to insulin resistance or have an increased proportion of body fat in the abdominal region. Many factors increase the risk of developing T2DM, including family history, age, obesity, and lack of physical activity. Also, DM occurs more frequently in women with prior gestational DM and in individuals with hypertension or dyslipidemia. Symptoms of marked hyperglycemia include polyuria, polydypsia, weight loss, polyphagia, and blurred vision. Although the degree of hyperglycemia seen with T2DM may not cause symptoms initially, it is sufficient to cause pathologic and functional changes in target tissues, and as such, will increase the risk of microvascular and macrovascular complications. These long-term complications include retinopathy with potential loss of vision; nephropathy leading to renal failure; peripheral neuropathy with risk of foot ulcers, amputations, and Charcot joints; and autonomic neuropathy causing gastrointestinal, genitourinary, and cardiovascular symptoms and sexual dysfunction. Diabetic patients also have an increased incidence of atherosclerotic cardiovascular, peripheral arterial, and cerebrovascular disease. Glucose, a vital energy source for many cells and tissues, is tightly regulated via a complex interaction between pancreatic β-cells and α-cells, associated organs eg, intestines, liver, skeletal muscle, adipose tissue , and respective hormones ie, insulin, glucagon, GLP-1, GIP, amylin, and others. A summary of the major factors responsible for maintenance of normal glucose tolerance in healthy subjects is provided in the Table. The primary tissues involved in glucose utilization include the brain, muscle, fat, and the splanchnic area, with muscle tissue comprising the most important site of peripheral glucose uptake. Knowledge of the fundamentals of normal glucose homeostasis is essential to understanding the pathophysiologic derangements that may result from glucose imbalance disorders. Conditions such as T2DM are characterized by an imbalance in glucose regulation, causing chronic hyperglycemia and ultimately leading to multiorgan damage. Several factors are implicated in the development of T2DM, including insulin resistance, insulin deficiency, increased hepatic glucose production, and adipocyte dysfunction. An increasingly clear understanding of these derangements has helped both researchers and clinicians to better manage T2DM and improve clinical outcomes. Author affiliations: Department of Medicine, Division of Diabetes, University of Texas Health Science Center at San Antonio; and Texas Diabetes Institute, San Antonio, TX. Funding source: This activity is supported by an educational grant from Bristol-Myers Squibb and AstraZeneca LP. Author disclosure: Dr Triplitt reports being a consultant or a member of the advisory board for Roche and Takeda Pharmaceuticals. Authorship information: Concept and design; drafting of the manuscript; and critical revision of the manuscript for important intellectual content. Address correspondence to: E-mail: Curtis. Triplitt uhs-sa. All News. Press Releases. Product Approvals and Launches. Clinical Spotlight. Enduring Webinars. News Network. Payer Perspectives. Peer Exchange. Post Conference Perspectives. Stakeholder Summit. Week in Review. Conference Coverage. Conference Listing. Submit a Manuscript. All Journals. The American Journal of Managed Care. The American Journal of Accountable Care. Evidence-Based Oncology. Supplements and Featured Publications. Atopic Dermatitis. Breast Cancer. Chronic Kidney Disease. Colorectal Cancer. Digital Health. Duchenne Muscular Dystrophy. Gene Therapy. Heart Failure. Infectious Disease. Leukemia and Lymphoma. Lung Cancer. Major Depressive Disorder. Mental Health. These low levels of insulin allow the body to tap into its stored energy sources namely glycogen and fat and also to release sugar and other fuels from the liver. This overnight and between-meal insulin is referred to as background or basal insulin. When eating, the amount of insulin released from the pancreas rapidly spikes. This burst of insulin that accompanies eating is called bolus insulin. The high levels of insulin help the sugar get out of the blood stream and be stored for future use. To keep the blood glucose in a narrow range throughout the day, there is a low steady secretion of insulin overnight, fasting and between meals with spikes of insulin at mealtimes. Adapted: Jacobs DM Care , There are other hormones that work together with insulin to regulate blood sugar including incretins and gluco-counterregulatory hormones, but insulin is the most important. Self assessment quizzes are available for topics covered in this website. To find out how much you have learned about Facts about Diabetes , take our self assessment quiz when you have completed this section. |
How insulin and glucagon regulate blood sugar | The following diagram demonstrates an overview of aerobic respiration. Additionally, when there is sufficient energy in the cell G6P will be used for glycogen synthesis glycogenesis rather than entering glycolysis. Spinal Muscular Atrophy. The regulation of glycogen, and thus glucose, is controlled primarily through the peptide hormones insulin and glucagon. Neural control of blood glucose level. |
Self-assessment Quiz | Show details Treasure Island FL : StatPearls Publishing ; Jan-. Search term. Physiology, Glucose Paris J. Author Information and Affiliations Authors Paris J. Affiliations 1 SUNY Upstate Medical University. Introduction Glucose is a 6-carbon structure with the chemical formula C6H12O6. Cellular Level Glucose reserves get stored as the polymer glycogen in humans. SGLT : Found primarily in the renal tubules and intestinal epithelia, SGLTs are important for glucose reabsorption and absorption, respectively. This transporter works through secondary active transport as it requires ATP to actively pump sodium out of the cell and into the lumen, which then facilitates cotransport of glucose as sodium passively travels across the cell wall down its concentration gradient. GLUT1 : Found primarily in the pancreatic beta-cells, red blood cells, and hepatocytes. This bi-directional transporter is essential for glucose sensing by the pancreas, an important aspect of the feedback mechanism in controlling blood glucose with endogenous insulin. GLUT2 : Found primarily in hepatocytes, pancreatic beta-cells, intestinal epithelium, and renal tubular cells. This bi-directional transporter is important for regulating glucose metabolism in the liver. GLUT3 : Found primarily in the CNS. This transporter has a very high affinity for glucose, consistent with the brain's increased metabolic demands. GLUT4 : Found primarily in skeletal muscle, cardiac muscle, adipose tissue, and brain tissue. This transporter gets stored in cytoplasmic vesicles inactive , which will amalgamate with the cell membrane when stimulated by insulin. These transporters will experience a 10 to fold increase in density in times of energy-excess upon the release of insulin with the net effect of a decrease in blood glucose glucose will more readily enter the cells that have GLUT4 on their surface. Organ Systems Involved Glucose has a vital role in every organ system. Liver The liver is an important organ with regards to maintaining appropriate blood glucose levels. Pancreas The pancreas releases the hormones primarily responsible for the control of blood glucose levels. Insulin: decreases blood glucose through increased expression of GLUT4, increased expression of glycogen synthase, inactivation of phosphorylase kinase thus decreasing gluconeogenesis , and decreasing the expression of rate-limiting enzymes involved in gluconeogenesis. Somatostatin: decreases blood glucose levels through local suppression of glucagon release and suppression of gastrin and pituitary tropic hormones. This hormone also decreases insulin release; however, its net effect is a decrease in blood glucose levels. Cortisol: increases blood glucose levels via the stimulation of gluconeogenesis and through antagonism of insulin. Epinephrine: increases blood glucose levels through glycogenolysis glucose liberation from glycogen and increased fatty acid release from adipose tissues, which can then be catabolized and enter gluconeogenesis. Thyroxine: increases blood glucose levels through glycogenolysis and increased absorption in the intestine. Growth hormone: promotes gluconeogenesis, inhibits liver uptake of glucose, stimulates thyroid hormone, inhibits insulin. ACTH: stimulates cortisol release from adrenal glands, stimulates the release of fatty acids from adipose tissue, which can then feed into gluconeogenesis. Clinical Significance The pathology associated with glucose often occurs when blood glucose levels are either too high or too low. Hyperglycemia : Hyperglycemia can cause pathology, both acutely and chronically. In both cases, the result is inappropriately elevated blood glucose, which causes pathology by a variety of mechanisms: Osmotic damage : Glucose is osmotically active and can cause damage to peripheral nerves. Oxidative stress : Glucose participates in several reactions that produce oxidative byproducts. Non-enzymatic glycation : Glucose can complex with lysine residues on proteins causing structural and functional disruption. Neurogenic - Cholinergic : paresthesias, diaphoresis, and hunger. Review Questions Access free multiple choice questions on this topic. Comment on this article. Figure Glucose Transporters Contributed by Paris Hantzidiamantis. References 1. Gurung P, Zubair M, Jialal I. StatPearls Publishing; Treasure Island FL : Jan 18, Plasma Glucose. Daghlas SA, Mohiuddin SS. StatPearls Publishing; Treasure Island FL : May 1, Biochemistry, Glycogen. Holesh JE, Aslam S, Martin A. StatPearls Publishing; Treasure Island FL : May 12, Physiology, Carbohydrates. Navale AM, Paranjape AN. Glucose transporters: physiological and pathological roles. Biophys Rev. El Sayed SA, Mukherjee S. Physiology, Pancreas. Vargas E, Joy NV, Carrillo Sepulveda MA. StatPearls Publishing; Treasure Island FL : Sep 26, Biochemistry, Insulin Metabolic Effects. Venugopal SK, Sankar P, Jialal I. StatPearls Publishing; Treasure Island FL : Mar 6, Physiology, Glucagon. Dutt M, Wehrle CJ, Jialal I. Physiology, Adrenal Gland. Pirahanchi Y, Tariq MA, Jialal I. StatPearls Publishing; Treasure Island FL : Feb 13, Physiology, Thyroid. Rawindraraj AD, Basit H, Jialal I. Physiology, Anterior Pituitary. Nawale RB, Mourya VK, Bhise SB. Non-enzymatic glycation of proteins: a cause for complications in diabetes. Indian J Biochem Biophys. Goyal R, Singhal M, Jialal I. StatPearls Publishing; Treasure Island FL : Jun 23, Type 2 Diabetes. Goyal R, Nguyen M, Jialal I. StatPearls Publishing; Treasure Island FL : Aug 8, Glucose Intolerance. Adeyinka A, Kondamudi NP. StatPearls Publishing; Treasure Island FL : Aug 12, Hyperosmolar Hyperglycemic Syndrome. Mathew P, Thoppil D. StatPearls Publishing; Treasure Island FL : Dec 26, Kalra A, Yetiskul E, Wehrle CJ, Tuma F. Physiology, Liver. Patricia JJ, Dhamoon AS. StatPearls Publishing; Treasure Island FL : Sep 12, Physiology, Digestion. Copyright © , StatPearls Publishing LLC. Bookshelf ID: NBK PMID: PubReader Print View Cite this Page Hantzidiamantis PJ, Awosika AO, Lappin SL. Physiology, Glucose. In: StatPearls [Internet]. In this Page. Introduction Cellular Level Organ Systems Involved Clinical Significance Review Questions References. Bulk Download. Bulk download StatPearls data from FTP. Related information. PMC PubMed Central citations. Similar articles in PubMed. Biochemistry, Anaerobic Glycolysis. Melkonian EA, Schury MP. Review A quick look at biochemistry: carbohydrate metabolism. Dashty M. Clin Biochem. Epub May Review Protein turnover, ureagenesis and gluconeogenesis. Schutz Y. Int J Vitam Nutr Res. Recent Activity. Clear Turn Off Turn On. Physiology, Glucose - StatPearls. Follow NCBI. Twitter Facebook LinkedIn GitHub NCBI Insights Blog. In addition FBPase-2 is activated which breaks downs F-2,6-BP which also decreases the amount of F-2,6-BP. Low levels of F-2,6-BP cause the activation of FBPase-1 which increase gluconeogenesis. In addition there is no activation of PFK-1, which is involved in glycolysis both of these actions increase the amount of blood glucose. During extreme stimuli the sympathetic nervous system kicks into action. This is colloquially known as the fight or flight response illustrated in figure 4. The brain sends signals throughout the body to increase heart rate, cause bronchial dilation and haptic glucose release. The brain also sends signals to the adrenal glands. Epinephrine is released from the adrenal glands. This hormone is key to the prolonged sympathetic response. Epinephrine travels through the blood stream and causes the liver to release glucose thus increasing blood glucose levels in order to be ready for the threat. Epinephrine binds to a transmembrane receptor on the liver known as the beta-adrenergic receptor protein. The receptor activates a G protein which then activates. Once activated the G protein diffuses along the membrane. The G protein then binds with and activates adenylyl cyclase. Adenylyl cyclase causes ATP to become cyclic AMP cAMP. The molecule cAMP binds with protein kinase-A which phosporalayts specific proteins. In this step phosphorylase is phosphorylated. This is further refined into glucosephosphate by phosphoglucomutase. Glucosephosphate can then be used for energy requirements necessary in the sympathetic nervous system response. This allows for more ATP in the muscle and brain. When there is a issue with the ability of the human body to use glucose due to a problem with the insulin pathway diabetes mellitus diabetes mellitus may be at fault. There are a few types of diabetes mellitus with the most common being: type 1 diabetes mellitus, type 2 Diabetes mellitus and gestational Diabetes mellitus. Type 1 diabetes mellitus is where no insulin is made. This is due to damage to the pancreatic beta cells, often due to an autoimmune disorder. With out insulin the glucose can not go into the cells and will not make energy. As a result treatment for type 1 diabetes mellitus is regular injections of insulin. People who are obese have a high risk for contracting type 2 Diabetes mellitus. Although there is no cure for type 2 careful monitoring of exercise and nutrition can help. Other effects of the body are in increased rate of stroke and heart issues. Also damage to small blood vessels can occur. This happens particularly in the eyes diabetic retinopathy and kidneys renalopathy. Also due to increased amounts of sugar in the blood stream a diabetic is at a higher risk of infection and wounds will take longer to heal. Alcohol rapidly effects glucose levels in the blood stream which can be a challenge for those dealing with Diabetes mellitus. Alcohol effects many parts of the body and as a result has many pathways for effecting blood glucose levels. Alcohol can cause one to make poor decisions which will effect how you eat, this very directly effects blood glucose levels. This effect is more dramatic with drinks high in carbohydrates such a beer. The carbohydrates in beer can be metabolized and create glucose creating a temporary increase in blood glucose levels. Alcohol has energetic properties to its molecular structure and as a result the body can gain energy from it. Through the use of enzymes: alcohol dehydrogenase, cytochrome P, catalase alcohol is broken down. Alcohol dehydrogenase, the main enzyme in this catabolic reaction, produces NADH. NADH is the energy molecule that drives lactic acid formation. Glucose is turned into pyruvate through the use of ATP and then into lactic acid with NADH. This increase of NADH leads to a decrease in glucose [15]. The enzyme cytochrome P is activated when heavy drinking occurs. This enzyme strips an electron from NADPH in the metabolism of alcohol, which requires energy. However studies on the effects of alcohol leave one wanting for straight forward data on blood glucose levels in real life situations. In an article published by Gin et al. the findings show no adverse effects on diabetic participants after moderate alcohol consumption. The American Diabetes Association and other medical information outlets concur with this finding, that moderate consumption of alcohol produce no adverse effects. However acute ingestion of alcohol has also been reported to increase insulin secretion, resulting in low blood glucose. Research also indicates that heavy drinking will cause insulin to become ineffective, resulting in high blood sugar levels. It is unclear if the high blood sugar levels are from heavy drinking alone or are connected to obesity. Further complicating this is a high correlation between heavy drinking and obesity. Also a review of the effects of alcohol indicates that both glycolysis and gluconeogenesis will be inhibited. cederbaum, As the liver is key in the release of glucose into the blood stream and issue with it can prevent healthy maintenance of blood glucose levels. A disease where inflammation of the liver causes problems with the liver function is called alcoholic hepatitis. The liver can also become hard with heavy drinking due to scare tissue formation this is called fibrosis this is shown in figure 5. The pancreas is vital for blood glucose regulation and alcohol can have a significant effect on this organ. The pancreas creates digestive enzymes to metabolize the alcohol and these enzymes destabilize the cell membrane of the pancreatic cells. This leaves the cell liable to auto-digestion. A connection has been shown between decreased gut bacteria diversity and obesity. All cells in the human body require glucose to survive. However excessive amounts of glucose can also be detrimental to the body. In order to maintain this balance the body uses hormonal regulation. The body will detect high levels of blood glucose and release insulin to allow the sugar into cells. If there is not enough blood glucose, glucagon will be released. This hormone will cause the formation of glucose and the release of glucose stores. If the body is presented with a threat it will initiate the sympathetic nervous system. Part of this response is the release of epinephrine which causes haptic glucose release. If the body can not properly regulate blood glucose levels than it may be a form of diabetes. Diabetes type one stems from an inability to produce insulin. Diabetes type two is due to an insensitivity to insulin. Due to the blood glucose regulatory systems wide reach on the body when alcohol which also has a whole body effect a reaction is bond to occur. Blood glucose levels may initially increase however this is only in the case of high carbohydrate alcoholic beverages, such as beer. Due to the metabolism of alcohol glucose will be turned into pyruvate and blood glucose levels will drop. Alcohol's effects on judgment are also likely to lead to a drop in blood sugar. New York: W H Freeman; |
Blood Glucose Regulation - microbewiki | Normally, following glucose ingestion, the increase in plasma glucose concentration triggers insulin release, which stimulates splanchnic and peripheral glucose uptake and suppresses endogenous primarily hepatic glucose production. Among the various hormones involved in glucose regulation, insulin and glucagon both produced in the pancreas by islets of Langerhans are the most relevant. Insulin, a potent antilipolytic inhibiting fat breakdown hormone, is known to reduce blood glucose levels by accelerating transport of glucose into insulin-sensitive cells and facilitating its conversion to storage compounds via glycogenesis conversion of glucose to glycogen and lipogenesis fat formation. Incretins, which include glucose-dependent insulinotropic polypeptide GIP and glucagon-like peptide-1 GLP-1 , are also involved in regulation of blood glucose, in part by their effects on insulin and glucagon. Normally, these hormones are released in response to meals and, by activating certain receptors G protein—coupled on pancreatic β-cells, they aid in stimulation of insulin secretion. When glucose levels are low, however, GLP-1 and GIP levels and their stimulating effects on insulin secretion are diminished. Since glucose cannot readily diffuse through impermeable cell membranes, it requires assistance from both insulin and a family of transport proteins facilitated glucose transporter [GLUT] molecules in order to gain entry into most cells. While the liver does not require insulin to facilitate glucose uptake, it does need insulin to regulate glucose output. The kidneys are increasingly recognized to play an important role in glucose homeostasis via release of glucose into the circulation gluconeogenesis , uptake of glucose from the circulation to meet renal energy needs, and reabsorption of glucose at the proximal tubule. DM is a group of metabolic diseases characterized by hyperglycemia. The hallmark state of chronic hyperglycemia is associated with long-term damage, dysfunction, and potential failure of different organs, especially the eyes, kidneys, nerves, heart, and blood vessels. The pancreas has a remarkable capacity to adapt to conditions of increased insulin demand eg, in obesity, pregnancy, cortisol excess to maintain normoglycemia. Compensatory hyperinsulinemia maintains glucose homeostasis. However, when β-cell secretion of insulin becomes inadequate for the glucose load, hyperglycemia occurs. Progressive deterioration in β-cell function and mass is well known to occur over time in T2DM and the resultant state of impaired insulin secretion is found uniformly in T2DM patients of all ethnic backgrounds. Research indicates that progressive impaired β-cell function and possibly β-cell mass may be arrested, though clinical evidence in humans remains scarce. Impaired insulin secretion is often exacerbated by insulin resistance, which is characterized by the inability of insulin to decrease plasma glucose levels through suppression of hepatic glucose production and stimulation of glucose utilization in skeletal muscle and adipose tissue. Insulin resistance is contributed to by genetic and environmental factors. Family history can contribute directly to insulin resistance, but multiple environmental factors such as obesity, comorbidities, and central adiposity visceral can all contribute. The exact cause of insulin resistance in any given patient is complex, but may include defects in insulinmediated cell signaling pathways, reduced insulin-stimulated muscle glycogen synthesis, 18 or even potentially fewer insulin receptors particularly in skeletal muscle, liver, and adipose tissue in obese subjects. The relative contribution of insulin secretion and insulin resistance to the development of hyperglycemia may differ due to the heterogeneity of T2DM. Under most circumstances, insulin resistance is the earliest detectable defect in individuals with prediabetes. Other increasingly more well-understood mechanisms contributing to the pathophysiology of T2DM include increased hepatic glucose output and adipocyte dysfunction. Following glucose ingestion, insulin is normally secreted into the portal vein, where it is taken up by the liver and suppresses hepatic glucose output. However, if the liver does not perceive this insulin signal and continues to produce glucose, the 2 sources of glucose input from the liver and the gastrointestinal tract will result in marked hyperglycemia. With regard to adipocyte dysfunction, considerable evidence implicates deranged metabolism and altered disposition of fat in the pathogenesis of glucose intolerance in T2DM. Beyond this phenomenon, dysfunctional fat cells also produce excessive amounts of insulin resistance—inducing, inflammatory, and atherosclerotic-provoking cytokines and fail to secrete normal amounts of insulin-sensitizing adipocytokines adiponectin. They are also major sources of proinflammatory adipocytokines. Within liver cells, the elevated free fatty acids are converted to triglycerides, which accumulate and cause steatosis or fatty liver and consequently may increase the chances of nonalcoholic steatohepatitis NASH and even cirrhosis. Treatment should be based upon reversal of known pathogenic abnormalities and should not be directed simply at the reduction of A1C. Early initiation of therapy may help to prevent or slow progressive β-cell failure. The majority of patients with T2DM are either obese with obesity itself contributing to insulin resistance or have an increased proportion of body fat in the abdominal region. Many factors increase the risk of developing T2DM, including family history, age, obesity, and lack of physical activity. Also, DM occurs more frequently in women with prior gestational DM and in individuals with hypertension or dyslipidemia. Symptoms of marked hyperglycemia include polyuria, polydypsia, weight loss, polyphagia, and blurred vision. Although the degree of hyperglycemia seen with T2DM may not cause symptoms initially, it is sufficient to cause pathologic and functional changes in target tissues, and as such, will increase the risk of microvascular and macrovascular complications. These long-term complications include retinopathy with potential loss of vision; nephropathy leading to renal failure; peripheral neuropathy with risk of foot ulcers, amputations, and Charcot joints; and autonomic neuropathy causing gastrointestinal, genitourinary, and cardiovascular symptoms and sexual dysfunction. Diabetic patients also have an increased incidence of atherosclerotic cardiovascular, peripheral arterial, and cerebrovascular disease. Glucose, a vital energy source for many cells and tissues, is tightly regulated via a complex interaction between pancreatic β-cells and α-cells, associated organs eg, intestines, liver, skeletal muscle, adipose tissue , and respective hormones ie, insulin, glucagon, GLP-1, GIP, amylin, and others. A summary of the major factors responsible for maintenance of normal glucose tolerance in healthy subjects is provided in the Table. The primary tissues involved in glucose utilization include the brain, muscle, fat, and the splanchnic area, with muscle tissue comprising the most important site of peripheral glucose uptake. Knowledge of the fundamentals of normal glucose homeostasis is essential to understanding the pathophysiologic derangements that may result from glucose imbalance disorders. Conditions such as T2DM are characterized by an imbalance in glucose regulation, causing chronic hyperglycemia and ultimately leading to multiorgan damage. Several factors are implicated in the development of T2DM, including insulin resistance, insulin deficiency, increased hepatic glucose production, and adipocyte dysfunction. An increasingly clear understanding of these derangements has helped both researchers and clinicians to better manage T2DM and improve clinical outcomes. Author affiliations: Department of Medicine, Division of Diabetes, University of Texas Health Science Center at San Antonio; and Texas Diabetes Institute, San Antonio, TX. Funding source: This activity is supported by an educational grant from Bristol-Myers Squibb and AstraZeneca LP. Author disclosure: Dr Triplitt reports being a consultant or a member of the advisory board for Roche and Takeda Pharmaceuticals. Authorship information: Concept and design; drafting of the manuscript; and critical revision of the manuscript for important intellectual content. Address correspondence to: E-mail: Curtis. Triplitt uhs-sa. All News. Press Releases. Product Approvals and Launches. Clinical Spotlight. Enduring Webinars. News Network. Payer Perspectives. Peer Exchange. Post Conference Perspectives. Stakeholder Summit. Week in Review. Conference Coverage. Conference Listing. Submit a Manuscript. All Journals. The American Journal of Managed Care. In more severe circumstances, it is treated by injection or infusion of glucagon. When levels of blood sugar rise, whether as a result of glycogen conversion, or from digestion of a meal, a different hormone is released from beta cells found in the islets of Langerhans in the pancreas. When insulin binds to the receptors on the cell surface, vesicles containing the GLUT4 transporters come to the plasma membrane and fuse together by the process of endocytosis , thus enabling a facilitated diffusion of glucose into the cell. As soon as the glucose enters the cell, it is phosphorylated into glucosephosphate in order to preserve the concentration gradient so glucose will continue to enter the cell. There are also several other causes for an increase in blood sugar levels. Among them are the 'stress' hormones such as epinephrine also known as adrenaline , several of the steroids, infections, trauma, and of course, the ingestion of food. Diabetes mellitus type 1 is caused by insufficient or non-existent production of insulin, while type 2 is primarily due to a decreased response to insulin in the tissues of the body insulin resistance. Both types of diabetes, if untreated, result in too much glucose remaining in the blood hyperglycemia and many of the same complications. Contents move to sidebar hide. Article Talk. Read Edit View history. Tools Tools. What links here Related changes Upload file Special pages Permanent link Page information Cite this page Get shortened URL Download QR code Wikidata item. Download as PDF Printable version. In other projects. Wikimedia Commons. Hormones regulating blood sugar levels. In this step phosphorylase is phosphorylated. This is further refined into glucosephosphate by phosphoglucomutase. Glucosephosphate can then be used for energy requirements necessary in the sympathetic nervous system response. This allows for more ATP in the muscle and brain. When there is a issue with the ability of the human body to use glucose due to a problem with the insulin pathway diabetes mellitus diabetes mellitus may be at fault. There are a few types of diabetes mellitus with the most common being: type 1 diabetes mellitus, type 2 Diabetes mellitus and gestational Diabetes mellitus. Type 1 diabetes mellitus is where no insulin is made. This is due to damage to the pancreatic beta cells, often due to an autoimmune disorder. With out insulin the glucose can not go into the cells and will not make energy. As a result treatment for type 1 diabetes mellitus is regular injections of insulin. People who are obese have a high risk for contracting type 2 Diabetes mellitus. Although there is no cure for type 2 careful monitoring of exercise and nutrition can help. Other effects of the body are in increased rate of stroke and heart issues. Also damage to small blood vessels can occur. This happens particularly in the eyes diabetic retinopathy and kidneys renalopathy. Also due to increased amounts of sugar in the blood stream a diabetic is at a higher risk of infection and wounds will take longer to heal. Alcohol rapidly effects glucose levels in the blood stream which can be a challenge for those dealing with Diabetes mellitus. Alcohol effects many parts of the body and as a result has many pathways for effecting blood glucose levels. Alcohol can cause one to make poor decisions which will effect how you eat, this very directly effects blood glucose levels. This effect is more dramatic with drinks high in carbohydrates such a beer. The carbohydrates in beer can be metabolized and create glucose creating a temporary increase in blood glucose levels. Alcohol has energetic properties to its molecular structure and as a result the body can gain energy from it. Through the use of enzymes: alcohol dehydrogenase, cytochrome P, catalase alcohol is broken down. Alcohol dehydrogenase, the main enzyme in this catabolic reaction, produces NADH. NADH is the energy molecule that drives lactic acid formation. Glucose is turned into pyruvate through the use of ATP and then into lactic acid with NADH. This increase of NADH leads to a decrease in glucose [15]. The enzyme cytochrome P is activated when heavy drinking occurs. This enzyme strips an electron from NADPH in the metabolism of alcohol, which requires energy. However studies on the effects of alcohol leave one wanting for straight forward data on blood glucose levels in real life situations. In an article published by Gin et al. the findings show no adverse effects on diabetic participants after moderate alcohol consumption. The American Diabetes Association and other medical information outlets concur with this finding, that moderate consumption of alcohol produce no adverse effects. However acute ingestion of alcohol has also been reported to increase insulin secretion, resulting in low blood glucose. Research also indicates that heavy drinking will cause insulin to become ineffective, resulting in high blood sugar levels. It is unclear if the high blood sugar levels are from heavy drinking alone or are connected to obesity. Further complicating this is a high correlation between heavy drinking and obesity. Also a review of the effects of alcohol indicates that both glycolysis and gluconeogenesis will be inhibited. cederbaum, As the liver is key in the release of glucose into the blood stream and issue with it can prevent healthy maintenance of blood glucose levels. A disease where inflammation of the liver causes problems with the liver function is called alcoholic hepatitis. The liver can also become hard with heavy drinking due to scare tissue formation this is called fibrosis this is shown in figure 5. The pancreas is vital for blood glucose regulation and alcohol can have a significant effect on this organ. The pancreas creates digestive enzymes to metabolize the alcohol and these enzymes destabilize the cell membrane of the pancreatic cells. This leaves the cell liable to auto-digestion. A connection has been shown between decreased gut bacteria diversity and obesity. All cells in the human body require glucose to survive. However excessive amounts of glucose can also be detrimental to the body. In order to maintain this balance the body uses hormonal regulation. The body will detect high levels of blood glucose and release insulin to allow the sugar into cells. If there is not enough blood glucose, glucagon will be released. This hormone will cause the formation of glucose and the release of glucose stores. |
Physiology, Glucose - StatPearls - NCBI Bookshelf | The others increase glucose levels. The pathology associated with glucose often occurs when blood glucose levels are either too high or too low. Below is a summary of some of the more common pathological states with associations to alterations in glucose levels and the pathophysiology behind them. Hyperglycemia can cause pathology, both acutely and chronically. Diabetes mellitus I and II are both disease states characterized by chronically elevated blood glucose levels that, over time and with poor glucose control, leads to significant morbidity. Both classes of diabetes have multifocal etiologies: type I is associated with genetic, environmental, and immunological factors and most often presents in pediatric patients, while type II is associated with comorbid conditions such as obesity in addition to genetic factors and is more likely to manifest in adulthood. Type I diabetes results from autoimmune destruction of pancreatic beta-cells and insulin deficiency, while type II results from peripheral insulin resistance owing to metabolic dysfunction, usually in the setting of obesity. In both cases, the result is inappropriately elevated blood glucose, which causes pathology by a variety of mechanisms:. These mechanisms lead to a variety of clinical manifestations through both microvascular and macrovascular complications. It is imperative to understand the mechanisms behind the pathology caused by elevated glucose. High blood sugars can also lead to acute pathology, most often seen in patients with type II diabetes, known as a hyperosmolar hyperglycemic state. This state occurs when there is a severely elevated blood glucose level resulting in elevated plasma osmolality. The high osmolarity leads to osmotic diuresis excessive urination and dehydration. Hypoglycemia is most often seen iatrogenically in diabetic patients secondary to glucose-lowering drugs. This condition occurs, especially in the inpatient setting, with the interruption of the patient's usual diet. The symptoms are non-specific, but clinical findings such as relation to fasting or exercise and symptom improvement with glucose administration make hypoglycemia more likely. Hypoglycemia symptoms can be described as either neuroglycopenic, owning to a direct effect on the CNS, or neurogenic, owing to sympathoadrenergic involvement. Neurogenic symptoms can be further broken down into either cholinergic or adrenergic. Below are some common symptoms of hypoglycemia:. Tying what we have learned about glucose together in a brief overview of glucose metabolism consider that you eat a carbohydrate-dense meal. The various polymers of glucose will be broken down in your saliva and intestines, liberating free glucose. This glucose will be absorbed into the intestinal epithelium through SGLT receptors apically and then enter your bloodstream through GLUT receptors on the basolateral wall. Your blood glucose level will spike, causing an increased glucose concentration in the pancreas, stimulating the release of pre-formed insulin. Insulin will have several downstream effects, including increased expression of enzymes involved with glycogen synthesis such as glycogen synthase in the liver. The glucose will enter hepatocytes and get added to glycogen chains. Insulin will also stimulate the liberation of GLUT4 from their intracellular confinement, which will increase basal glucose uptake into muscle and adipose tissue. As blood glucose levels begin to dwindle as it enters peripheral tissue and the liver , insulin levels will also come down to the low-normal range. As the insulin level falls below normal, glucagon from pancreatic alpha-cells will be released, promoting a rise in blood glucose via its liberation from glycogen and via gluconeogenesis; this will usually increase glucose levels enough to last until the next meal. However, if the patient continues to fast, the adrenomedullary system will join in and secrete cortisol and epinephrine, which also works to establish euglycemia from a hypoglycemic state. Disclosure: Paris Hantzidiamantis declares no relevant financial relationships with ineligible companies. Disclosure: Ayoola Awosika declares no relevant financial relationships with ineligible companies. Disclosure: Sarah Lappin declares no relevant financial relationships with ineligible companies. This book is distributed under the terms of the Creative Commons Attribution-NonCommercial-NoDerivatives 4. You are not required to obtain permission to distribute this article, provided that you credit the author and journal. Turn recording back on. National Library of Medicine Rockville Pike Bethesda, MD Web Policies FOIA HHS Vulnerability Disclosure. Help Accessibility Careers. Access keys NCBI Homepage MyNCBI Homepage Main Content Main Navigation. Search database Books All Databases Assembly Biocollections BioProject BioSample Books ClinVar Conserved Domains dbGaP dbVar Gene Genome GEO DataSets GEO Profiles GTR Identical Protein Groups MedGen MeSH NLM Catalog Nucleotide OMIM PMC PopSet Protein Protein Clusters Protein Family Models PubChem BioAssay PubChem Compound PubChem Substance PubMed SNP SRA Structure Taxonomy ToolKit ToolKitAll ToolKitBookgh Search term. StatPearls [Internet]. Treasure Island FL : StatPearls Publishing; Jan-. Show details Treasure Island FL : StatPearls Publishing ; Jan-. Search term. Physiology, Glucose Paris J. Author Information and Affiliations Authors Paris J. Affiliations 1 SUNY Upstate Medical University. Introduction Glucose is a 6-carbon structure with the chemical formula C6H12O6. Cellular Level Glucose reserves get stored as the polymer glycogen in humans. SGLT : Found primarily in the renal tubules and intestinal epithelia, SGLTs are important for glucose reabsorption and absorption, respectively. This transporter works through secondary active transport as it requires ATP to actively pump sodium out of the cell and into the lumen, which then facilitates cotransport of glucose as sodium passively travels across the cell wall down its concentration gradient. GLUT1 : Found primarily in the pancreatic beta-cells, red blood cells, and hepatocytes. This bi-directional transporter is essential for glucose sensing by the pancreas, an important aspect of the feedback mechanism in controlling blood glucose with endogenous insulin. GLUT2 : Found primarily in hepatocytes, pancreatic beta-cells, intestinal epithelium, and renal tubular cells. This bi-directional transporter is important for regulating glucose metabolism in the liver. GLUT3 : Found primarily in the CNS. This transporter has a very high affinity for glucose, consistent with the brain's increased metabolic demands. GLUT4 : Found primarily in skeletal muscle, cardiac muscle, adipose tissue, and brain tissue. This transporter gets stored in cytoplasmic vesicles inactive , which will amalgamate with the cell membrane when stimulated by insulin. These transporters will experience a 10 to fold increase in density in times of energy-excess upon the release of insulin with the net effect of a decrease in blood glucose glucose will more readily enter the cells that have GLUT4 on their surface. Organ Systems Involved Glucose has a vital role in every organ system. Liver The liver is an important organ with regards to maintaining appropriate blood glucose levels. Pancreas The pancreas releases the hormones primarily responsible for the control of blood glucose levels. Insulin: decreases blood glucose through increased expression of GLUT4, increased expression of glycogen synthase, inactivation of phosphorylase kinase thus decreasing gluconeogenesis , and decreasing the expression of rate-limiting enzymes involved in gluconeogenesis. Somatostatin: decreases blood glucose levels through local suppression of glucagon release and suppression of gastrin and pituitary tropic hormones. This hormone also decreases insulin release; however, its net effect is a decrease in blood glucose levels. Cortisol: increases blood glucose levels via the stimulation of gluconeogenesis and through antagonism of insulin. Epinephrine: increases blood glucose levels through glycogenolysis glucose liberation from glycogen and increased fatty acid release from adipose tissues, which can then be catabolized and enter gluconeogenesis. Thyroxine: increases blood glucose levels through glycogenolysis and increased absorption in the intestine. Growth hormone: promotes gluconeogenesis, inhibits liver uptake of glucose, stimulates thyroid hormone, inhibits insulin. ACTH: stimulates cortisol release from adrenal glands, stimulates the release of fatty acids from adipose tissue, which can then feed into gluconeogenesis. Clinical Significance The pathology associated with glucose often occurs when blood glucose levels are either too high or too low. Hyperglycemia : Hyperglycemia can cause pathology, both acutely and chronically. In both cases, the result is inappropriately elevated blood glucose, which causes pathology by a variety of mechanisms: Osmotic damage : Glucose is osmotically active and can cause damage to peripheral nerves. Oxidative stress : Glucose participates in several reactions that produce oxidative byproducts. Non-enzymatic glycation : Glucose can complex with lysine residues on proteins causing structural and functional disruption. Neurogenic - Cholinergic : paresthesias, diaphoresis, and hunger. Review Questions Access free multiple choice questions on this topic. Comment on this article. Figure Glucose Transporters Contributed by Paris Hantzidiamantis. References 1. Gurung P, Zubair M, Jialal I. StatPearls Publishing; Treasure Island FL : Jan 18, Plasma Glucose. Daghlas SA, Mohiuddin SS. StatPearls Publishing; Treasure Island FL : May 1, Biochemistry, Glycogen. Holesh JE, Aslam S, Martin A. StatPearls Publishing; Treasure Island FL : May 12, Physiology, Carbohydrates. Navale AM, Paranjape AN. Glucose transporters: physiological and pathological roles. Biophys Rev. El Sayed SA, Mukherjee S. Physiology, Pancreas. Vargas E, Joy NV, Carrillo Sepulveda MA. StatPearls Publishing; Treasure Island FL : Sep 26, Biochemistry, Insulin Metabolic Effects. Venugopal SK, Sankar P, Jialal I. StatPearls Publishing; Treasure Island FL : Mar 6, Physiology, Glucagon. Dutt M, Wehrle CJ, Jialal I. Physiology, Adrenal Gland. Pirahanchi Y, Tariq MA, Jialal I. StatPearls Publishing; Treasure Island FL : Feb 13, Physiology, Thyroid. Rawindraraj AD, Basit H, Jialal I. Physiology, Anterior Pituitary. Nawale RB, Mourya VK, Bhise SB. Non-enzymatic glycation of proteins: a cause for complications in diabetes. Indian J Biochem Biophys. These hormones are generated and secreted by the pancreas and work together to maintain optimal blood glucose concentrations. The following diagram demonstrates an overview of aerobic respiration. You do not need to know the whole process in detail, but it is expected that you have a base understanding of this process from your previous courses, focussing on the big picture. The pancreas is a glandular organ located in the abdomen. It plays a critical role in converting the food we eat into fuel for our bodies. In terms of functionality, the pancreas can be broken down into two main parts the exocrine pancreas which aids in digestion and the endocrine pancreas which regulates blood sugar. The bulk of the pancreas is composed of exocrine cells which produce enzymes that aid in digestion. When food enters the stomach, the exocrine cells release their digestive enzymes into a series of small ducts that eventually join together into the main pancreatic duct. The pancreatic duct runs the length of the pancreas and releases the digestive enzymes along with other secretions, collectively called pancreatic juice, into the small intestine. The second functional component of the pancreas is the endocrine pancreas. The endocrine pancreas is composed of small islands of cells called the islets of Langerhans. There are at least 4 cell types found within the islets which produce hormones that are released into the blood stream and help regulate blood glucose levels. This information is summarized in table 1. The functional distribution of the four cell types within the islets of Langerhans is shown in figure 2. Beta cells, which make up the majority of the islets, are located centrally and surrounded by the alpha, delta and F cells. This learning object is above the course level and for your information only. This learning object is beyond what you are expected to know; however, think about where the cell type subsets are situated and the functional role location might have. Glucagon and insulin are antagonistic hormones and somatostatin inhibits them both! It makes sense that they are all found close together. Before pancreatic hormones make it into circulation and act on tissues around the body, they first play a role in paracrine regulation of the pancreas itself. This paracrine feedback system is demonstrated in figure 3. Take note of the signalling contrast between glucagon and insulin and somatostatin. Glucagon will always stimulate the release of the other two hormones, while insulin and somatostatin both have an inhibitory effect on the other hormones in this relationship. Insulin and glucagon have opposing actions on one another, so if you learn one, you know the other! And remember that somatostatin will always inhibit both insulin and glucagon release. Secretion of insulin, from beta cells within the islet of Langerhans, inhibits the surrounding alpha cells from releasing glucagon and the delta cells from releasing somatostatin. Secretion of glucagon, which is antagonistic to insulin, stimulates the delta cells to release somatostatin. Interestingly, glucagon also activates the beta cells and stimulates insulin release. Considering insulin and glucagon have opposing actions throughout the body this may seem counterintuitive. To better understand why this occurs imagine a runner nearing the end of a marathon — after running almost 42 kilometers, glucose within the body will be severely depleted. In order to correct this state of hypoglycaemia, alpha cells in the pancreas will release glucagon, resulting in the production and liberation of glucose from the liver and adipocytes. Insulin is then needed to help cells around the body especially the skeletal muscle cells absorb the liberated glucose and use it for energy. This interplay between glucagon and insulin allows the runner to keep moving and finish the race! It is also important to understand that not all regulatory signals are equal; the stimulatory effects that glucagon has on beta cells is much smaller than the stimulatory effects of increased blood sugar after eating a meal. Lastly, secretion of somatostatin within the islets inhibits the activity of both the beta and alpha cells. Regulation of pancreatic hormones is a complex process, involving much more than just the paracrine feedback system within the islet of Langerhans. Secretion of insulin and glucagon is controlled by the integration and interaction of multiple inputs including nutrients, hormones, neurotransmitters and drugs. For both insulin and glucagon, changes in blood glucose concentrations are the primary stimuli that activates, or inhibits, their release. Blood glucose is the regulated variable within this system, meaning it is constantly monitored by sensors i. receptors in the body and kept within a limited range through physiological mechanisms. When the body is in a state of hyperglycemia , and blood glucose levels are elevated, sensors in the pancreas detect this and stimulate the beta cells to increase their release of insulin. When blood glucose levels drop, putting the body is in a state of hypoglycemia , the alpha cells are stimulated and glucagon is released. The integration of blood glucose levels, and other regulatory stimuli, on alpha and beta cells is discussed in further detail below. The following figure depicts how insulin release is regulated by different inputs throughout the body. Keep the big picture in mind. Which one of the following exhibits paracrine control? The following figure demonstrates how the release of glucagon from alpha cells is regulated by different inputs throughout the body. Remember that both insulin and somatostatin will both inhibit the secretion of glucagon from alpha cells in the pancreas. The endocrine pancreas is always secreting some level of insulin and glucagon. Figure 6 below shows how plasma concentrations of glucose, glucagon and insulin change over a hour period. Pay close attention to how these levels change before and after a meal and the relationships between the different plasma concentrations. Think about the relationship between insulin, glucagon and glucose and what causes fluctuations to each. This diagram presents information that you have already learned in a new way! As one hormone increases its activity the other one decreases but is never completely shut off. All inputs that regulate the activity of alpha and beta cells combine in the pancreas. The overall summation of these inputs determines if the system favours insulin or glucagon release. After eating a meal glucose levels rise. In response to this the body increases the concentration of insulin in the blood and decreases the concentration of glucagon. The opposite effect is seen in between meals when blood glucose concentration decreases — now blood glucagon concentrations rise and insulin concentrations fall. Take note that the concentration of both insulin and glucagon in the blood never reaches 0, there is always some level of hormone being secreted from the pancreas. We know that regardless of blood glucose levels, the concentrations of insulin and glucagon never reach zero. Why do you think that is? Hint: Think about what would happen if you needed to produce a hormone quickly and it was not readily available. So far we have covered where insulin and glucagon come from, and how they are regulated. Now we will dive into the effects these hormones have on the body. The absorptive state , or the fed state, occurs after a meal when your body is digesting the food and absorbing the nutrients.. Digestion begins the moment you put food into your mouth, as the food is broken down into its constituent parts to be absorbed through the intestine. The digestion of carbohydrates begins in the mouth, whereas the digestion of proteins and fats begins in the stomach and small intestine. The constituent parts of these carbohydrates, fats, and proteins are transported across the intestinal wall and enter the bloodstream sugars and amino acids or the lymphatic system fats. The ingestion of food and the rise of glucose concentrations in the bloodstream stimulate pancreatic beta cells to release insulin. For the purpose of this course we will focus on the effects of insulin in adipose tissue, skeletal muscle and the liver. Figure 7 below provides a visual representation of how the adipose tissue, skeletal muscle and liver respond to an increase in insulin, caused by high blood glucose levels. Note the negative feedback that allows this response to be highly regulated. In adipose tissue, when insulin concentrations are low, glucose transport proteins are recycled slowly between the cell membrane and cell interior. Vesicles then fuse with the cell membrane and expose the GLUT4 transporters to the extracellular fluid. Insulin also increases the activity of pyruvate dehydrogenase and acetylCoA carboxylase within adipocytes, facilitating the conversion of absorbed glucose into triglycerides for lipid storage. In a similar fashion to adipose tissues, insulin causes the recruitment of GLUT4 transporters to the surface of skeletal muscle cells. Insulin further reduces blood glucose levels by stimulating glycolysis, the metabolism of glucose for generation of ATP, in the muscle. This is achieved by activating the enzymes phosphofructokinase and pyruvate dehydrogenase. Insulin also stimulates skeletal muscle to convert excess glucose into glycogen for storage by activating glycogen synthase and it inhibits enzymes involved in glycogenolysis glycogen phosphorylase. Lastly, insulin stimulates amino acids uptake and protein synthesis in the muscle tissue. Insulin increases absorption of blood glucose into hepatocytes by promoting the conversion of glucose into glucosephosphate G6P. This is achieved through activation of the enzyme glucokinase and inhibition of glucosephosphatase. By immediately converting glucose into G6P, the cell can maintain a concentration gradient where glucose levels are higher in the blood and lower inside the cell. Insulin increases the activity of enzymes involved in glycogen synthesis glycogen synthase and inhibits enzymes used for glycogenolysis glycogen phosphorylase. Once glycogen stores within the liver are filled, any remaining glucose is broken down and used for triglyceride synthesis and lipid storage. The figure below is an effective visual representation of the effects of insulin on liver, muscle and adipose cells in the absorptive state. Did you know not all cells require insulin for efficient uptake of glucose? Red blood cells, as well as cells of the brain, liver, kidneys and lining of the small intestine do not require insulin for efficient uptake of glucose! The post-absorptive state , or the fasting state, occurs when food has been digested, absorbed, and stored. You commonly fast overnight, but skipping meals during the day puts your body in the post absorptive state as well. During this state, the body must rely initially on stored glycogen. Glucose levels in the blood begin to drop as it is absorbed and used by the cells. In response to the decrease in glucose, insulin levels also drop. Glycogen and triglyceride storage slows. In response to a drop in blood glucose concentration, the hormone glucagon is released from the alpha cells of the pancreas. Glucagon acts upon primarily the liver and adipose cells. This diagram has a lot of processes and it can be a bit overwhelming to make sense of all of it at once. It is helpful to look at the big picture first, and then add in more details as you become more and more comfortable with the material. When blood sugar levels drop glucagon stimulates the liver to convert its stores of glycogen back into glucose. This response is known as glycogenolysis and is achieved by increasing the activity of glycogen phosphorylase and decreasing the activity of glycogen synthase. This glucose is then released from the liver to be used by the peripheral tissues and the brain. As a result, blood glucose levels begin to rise. Gluconeogenesis will also begin in the liver to replace the glucose that has been used by the peripheral tissues. To insure most of the the glucose being made in the liver is released into circulation for use by body cells, glucagon inhibits glycolysis by turning down the activity of phosphofructokinase and pyruvate dehydrogenase. Glucagon also increases release of glucose into the blood by activating glucosephosphatase and inhibiting glucokinase which allows any G6P within the cell to be converted back to glucose. Glucagon stimulates adipose tissue to breakdown stored triglycerides into free fatty acids and glycerol through a process called lipolysis. Some of the free glycerol is released into the blood stream and travels to the liver where it is used for gluconeogenesis. The effect of glucagon on skeletal muscle is beyond the scope of the course, so just focus on the effects on liver and adipose cells. So far in this chapter, insulin and glucagon have been discussed separately. This final section will show how insulin and glucagon work together in the body to maintain blood glucose homeostasis. Glucagon and insulin are antagonistic hormones and it can be helpful to think of them in terms of their opposing actions. If blood glucose concentration rises above this range, insulin is released, which stimulates body cells to remove glucose from the blood. This is achieved by increasing the rate of glucose transport into target cells, the use of glucose to generate ATP through glycolysis, storage of glucose as glycogen, triglyceride synthesis and lipid droplet formation, and amino acid absorption and protein synthesis. If blood glucose concentration drops below this range glucagon is released, which stimulates body cells to release glucose into the blood. This is achieved by stimulating the breakdown of glycogen to glucose, the breakdown of triglycerides into glycerol and free fatty acids as well as increased synthesis and release of glucose. The following diagram is a visual representation of the consequences of a disturbed blood glucose homeostasis and how the body works to re-establish homeostasis via the opposing actions of glucagon and insulin to maintain plasma glucose homeostasis. Take note that insulin will always want to build and store molecules, while glucagon will always want to break down molecules. By having opposing mechanisms throughout the body glucagon and insulin are able to work together and maintain blood glucose homeostasis. As one hormone increases its concentration and activity in the body the other one decreases. This hand off in function keeps blood glucose levels within the optimal range necessary for survival. The following figure displays the effects of insulin and glucagon on the body, as they work to restore homeostasis. Pay attention to the different organs that are targeted by these hormones and how they result in a change in plasma glucose levels. When trying to differentiate between glycolysis, glycogenesis, gluconeogenesis, lipolysis and lipogenesis it can be very overwhelming and confusing. Diabetes is a chronic disturbance of glucose homeostasis, which the body cannot fix on its own. There are several different types of diabetes outlined below. Type 1 diabetes is an autoimmune condition that often presents at a young age. For this reason, it is sometimes called juvenile diabetes. |
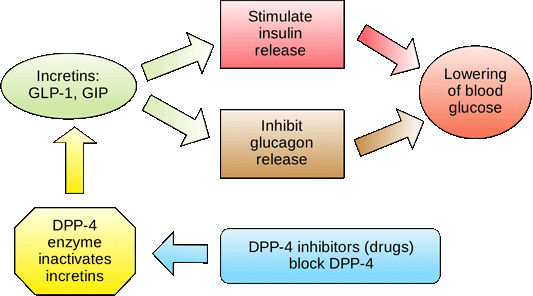
Entzückend
Bis jetzt ist aller gut.
Nach meiner Meinung lassen Sie den Fehler zu. Geben Sie wir werden es besprechen. Schreiben Sie mir in PM.