Optimized fat oxidizing process -
Athletes also tend to have larger IMTAG stores than lean sedentary individuals. Overweight and obese individuals, interestingly, also have high levels of IMTAG but are not able to use IMTAGs during exercise like athletic individuals can Shaw, Clark and Wagenmakers, So what causes the reduced ability to use IMTAGs in obese individuals?
A logical guess would be that they have dysfunctional mitochondria that cannot use fatty acid properly. Research has shown however, that the mitochondria from muscles of obese individuals are not dysfunctional Holloway et al.
Instead, the number of mitochondria per unit of muscle mitochondrial density is reduced in an obese population Holloway et al.
Reduced mitochondrial density is a more likely explanation for reduced ability to use fat for energy in obese individuals. An important adaptation to exercise training is increased mitochondrial density Horowitz and Klein ; Zuhl and Kravitz, Increasing mitochondrial density would improve the ability to use fat and benefit individuals with fat loss goals.
Endurance exercise training is an effective way to improve the body's fatty acid usage abilities by improving the availability of fatty acids to the muscle and mitochondria and by increasing fatty acid oxidation Horowitz and Klein, HIIT training has also been shown to result in similar fat burning adaptations while requiring fewer workouts and less total time commitment Zuhl and Kravitz, Practical application Rather than trying to maximize fat oxidation in a single bout of exercise, it is recommended that the personal trainer design a workout program aimed at causing muscle adaptations described above to improve fatty acid oxidation ability.
The exercise professional should include interval and endurance training programs as these have been shown to improve mitochondrial density and fat oxidation Zuhl and Kravitz, In addition, regular progressively increasing programs of resistance training are encouraged as this training will enhance EPOC and post-workout fat oxidation.
Also, the personal trainer should encourage the client to engage in low to moderate intensity exercise such as walking and cycling on off hard workout days in order to enhance caloric deficit and support muscle adaptions between training days. Workout examples High intensity interval training HIT with variable recovery modified from Seiler and Hetlelid, High intensity interval training uses exercise intensity that corresponds to the individual's VO2max.
Seiler and Hetlelid exercised subjects at their highest running speeds for 4 minutes with 1, 2 or 4 minutes of recovery and repeated this interval 6 times. The idea of a systematic variation of the recovery is a very novel approach to interval training and certainly warrants more research.
The workout Have the client complete up to 6 sets of 4-minute bouts at a maximal sustained workout effort and vary each recovery period to be 1 min, 2 min or 4 minutes at a light intensity client's self-selected intensity. Sprint interval training SIT Modified from Burgomaster et al.
The maximal effort generated in SIT necessitates a small work to larger rest ratio. That is, SIT is often done with a second all-out effort followed by a 4. The trainer can do SIT with clients using a variety of different modes of exercise including the stationary bike, elliptical cross-trainer and rowing machine.
The resistance on the chosen mode of exercise should be relatively challenging during the work bout. During the sprint interval the trainer should verbally encourage the client to maintain maximal effort throughout the bout.
During the recovery phase between bouts the client is encouraged to continue moving on the exercise machine at a very low self-selected light effort. The workout Have the client complete 3 to 4 bouts of second all-out bouts bout with 4.
Special Comments This is a very challenging workout. Modifications may be required to match the individual's fitness level needs. Resistance Training RT modified form Melby et al. This is total body weight lifting workout that uses 10 exercises.
The exercises are arranged in 5 pairs so that each pair of exercise is completed before resting and moving on to the next pair. The whole circuit of exercise should be completed up to 6 times. The rest interval between pairs should be no longer than 2 minutes.
The resistance used on each exercise should allow the client to lift 8 to 12 repetitions. The Workout- o Pair 1 o Bench press o Bent over row o Pair 2 o Split squat Right leg forward o Split squat Left leg forward o Pair 3 o Military press o Crunches o Pair 4 o Biceps curls o Triceps extensions o Pair 5 o Half squat o Lateral raises Special Comments As with any workout, exercise modifications or substitutions may be necessary to fit individual's fitness needs and abilities.
Tabata-inspired interval training modified form Tabata et al. Tabata-style training can use cardiovascular equipment such as the treadmill, rowing machine or stationary bike, or in calisthenics such as burpees, mountain climbers or body-weight squats.
During the rest interval, keep the client moving to avoid blood pooling in the lower extremities. This will also help prevent the client from feeling queasy or faint. The workout Have the client perform complete three sets of Tabata intervals, resting 3 minutes between sets.
Use burpees for the first set, the stationary bike for the second and the rowing machine for the third. Workout should last about 21 minutes. Comments This type of exercise has been shown to be effective at improving VO2max. Encourage the client maintain a challenging effort during this workout.
The personal trainer should provide verbal encouragement to help the client do this. Moderate intensity steady-state exercise MIR Light-to-moderate exercise should be encouraged on days when the client is recovering from one of the more intense condition workouts provided here.
This exercise should be restorative, allowing for the client's body to promote new muscle adaptations they have gaining from the more intense training. The Workout Walking is a great way to implement this workout. Encourage the client to walk around their neighborhood or local park for 30 minutes to 1 hour.
The walking pace should be that which the client can sustain a conversation. Bios: Mike Deyhle, B. S, CSCS, is an Exercise Science masters student at the University of New Mexico, Albuquerque.
Christine Mermier, Ph. is an assistant professor and exercise physiology laboratory director in the exercise science Program at UNM.
Her research interests include the effect of exercise in clinical patients, women, and aging populations, and high altitude physiology. Len Kravitz, PhD, is the program coordinator of exercise science and a researcher at the University of New Mexico, Albuquerque, where he won the Outstanding Teacher of the Year award.
He has received the prestigious Can-Fit-Pro Lifetime Achievement Award and American Council on Exercise Fitness Educator of the Year. References Achten, J. Optimizing fat oxidation through exercise. Burgomaster, K. Similar metabolic adaptations during exercise after low volume sprint interval and traditional endurance training in humans.
Journal of Applied Physiology, 1, Duncan, R. E, Ahmadian, M. Regulation of lipolysis in adipocytes. Annual Review of Nutrition. Hackney, K. Resting energy expenditure and delayed-onset muscle soreness after full-body resistance training with an eccentric concentration. Journal of Strength and Conditioning Research.
Holloway, G. Fat oxidation is regulated at various steps of this process. Lipolysis is affected by many factors but is mostly regulated by hormones stimulated by catecholamines and inhibited by insulin. The transport of fatty acids is also dependent on blood supply to the adipose and muscle tissues, as well as the uptake of fatty acids into the muscle and into the mitochondria.
By inhibiting mobilisation of fatty acids or the transport of these fatty acids, we can reduce fat metabolism. However, are there also ways in which we can stimulate these steps and promote fat metabolism? Exercise intensity — One of the most important factors that determines the rate of fat oxidation during exercise is the intensity.
Although several studies have described the relationship between exercise intensity and fat oxidation, only recently was this relationship studied over a wide range of intensities 2.
In absolute terms, carbohydrate oxidation increases proportionally with exercise intensity, whereas the rate of fat oxidation initially increases, but decreases again at higher exercise intensities see figure 1. So, although it is often claimed that you have to exercise at low intensities to oxidise fat, this is not necessarily true.
However, the inter-individual variation is very large. However, very little research has been done. Recently we used this intensity in a training study with obese individuals. Compared with interval training, their fat oxidation and insulin sensitivity improved more after four weeks steady-state exercise three times per week at an intensity that equalled their individual Fatmax 4.
Dietary effects — The other important factor is diet. A diet high in carbohydrate will suppress fat oxidation, and a diet low in carbohydrate will result in high fat oxidation rates. This effect of insulin on fat oxidation may last as long as six to eight hours after a meal, and this means that the highest fat oxidation rates can be achieved after an overnight fast.
Endurance athletes have often used exercise without breakfast as a way to increase the fat-oxidative capacity of the muscle.
Recently, a study was performed at the University of Leuven in Belgium, in which scientists investigated the effect of a six-week endurance training programme carried out for three days per week, each session lasting one to two hours 6.
The participants trained in either the fasted or carbohydrate-fed state. When training was conducted in the fasted state, the researchers observed a decrease in muscle glycogen use, while the activity of various proteins involved in fat metabolism was increased.
However, fat oxidation during exercise was the same in the two groups. It is possible, though, that there are small but significant changes in fat metabolism after fasted training; but, in this study, changes in fat oxidation might have been masked by the fact that these subjects received carbohydrate during their experimental trials.
It must also be noted that training after an overnight fast may reduce your exercise capacity and may therefore only be suitable for low- to moderate- intensity exercise sessions.
The efficacy of such training for weight reduction is also not known. Duration of exercise — It has long been established that oxidation becomes increasingly important as exercise progresses. During ultra-endurance exercise, fat oxidation can reach peaks of 1 gram per minute, although as noted in Dietary effects fat oxidation may be reduced if carbohydrate is ingested before or during exercise.
In terms of weight loss, the duration of exercise may be one of the key factors as it is also the most effective way to increase energy expenditure. Mode of exercise — The exercise modality also has an effect on fat oxidation.
Fat oxidation has been shown to be higher for a given oxygen uptake during walking and running, compared with cycling 7. The reason for this is not known, but it has been suggested that it is related to the greater power output per muscle fibre in cycling compared to that in running.
Gender differences — Although some studies in the literature have found no gender differences in metabolism, the majority of studies now indicate higher rates of fat oxidation in women. In a study that compared men and women over a wide range of exercise intensities, it was shown that the women had higher rates of fat oxidation over the entire range of intensities, and that their fat oxidation peaked at a slightly higher intensity 8.
The differences, however, are small and may not be of any physiological significance. There are many nutrition supplements on the market that claim to increase fat oxidation.
These supplements include caffeine, carnitine, hydroxycitric acid HCA , chromium, conjugated linoleic acid CLA , guarana, citrus aurantium, Asian ginseng, cayenne pepper, coleus forskholii, glucomannan, green tea, psyllium and pyruvate.
With few exceptions, there is little evidence that these supplements, which are marketed as fat burners, actually increase fat oxidation during exercise see table 1. One of the few exceptions however may be green tea extracts.
The mechanisms of this are not well understood but it is likely that the active ingredient in green tea, called epigallocatechin gallate EGCG — a powerful polyphenol with antioxidant properties inhibits the enzyme catechol O-methyltransferase COMT , which is responsible for the breakdown of noradrenaline.
This in turn may result in higher concentrations of noradrenaline and stimulation of lipolysis, making more fatty acids available for oxidation. Environment — Environmental conditions can also influence the type of fuel used. It is known that exercise in a hot environment will increase glycogen use and reduce fat oxidation, and something similar can be observed at high altitude.
Similarly, when it is extremely cold, and especially when shivering, carbohydrate metabolism appears to be stimulated at the expense of fat metabolism. At present, the only proven way to increase fat oxidation during exercise is to perform regular physical activity. Exercise training will up-regulate the enzymes of the fat oxidation pathways, increase mitochondrial mass, increase blood flow, etc.
Research has shown that as little as four weeks of regular exercise three times per week for minutes can increase fat oxidation rates and cause favourable enzymatic changes However, too little information is available to draw any conclusions about the optimal training programme to achieve these effects.
In one study we investigated maximal rates of fat oxidation in subjects with varying fitness levels. In this study, we had obese and sedentary individuals, as well as professional cyclists 9. VO2max ranged from Interestingly, although there was a correlation between maximal fat oxidation and maximal oxygen uptake, at an individual level, fitness cannot be used to predict fat oxidation.
What this means is that there are some obese individuals that have similar fat oxidation rates to professional cyclists see figure 2! The large inter-individual variation is related to factors such as diet and gender, but remains in large part unexplained. Fat burning is often associated with weight loss, decreases in body fat and increases in lean body mass.
However, it must be noted that such changes in body weight and body composition can only be achieved with a negative energy balance: you have to eat fewer calories than you expend, independent of the fuels you use!
The optimal exercise type, intensity, and duration for weight loss are still unclear. Current recommendations are mostly focused on increasing energy expenditure and increasing exercise volumes.
Finding the optimal intensity for fat oxidation might aid in losing weight fat loss and in weight maintenance, but evidence for this is currently lacking. It is also important to realise that the amount of fat oxidised during exercise is only small.
Fat oxidation rates are on average 0. So in order to oxidise 1kg of fat mass, more than 33 hours of exercise is required! The duration of exercise, however, plays a crucial role, with an increasing importance of fat oxidation with longer exercise.
Put another way, if the human body relied on carbohydrates to store energy, then a person would need to carry 31 kg Hibernating animals provide a good example for utilization of fat reserves as fuel.
For example, bears hibernate for about 7 months, and during this entire period, the energy is derived from degradation of fat stores. Migrating birds similarly build up large fat reserves before embarking on their intercontinental journeys.
The fat stores of young adult humans average between about 10—20 kg, but vary greatly depending on gender and individual disposition. The g or so of glycogen stored in the liver is depleted within one day of starvation.
Fatty acids are broken down to acetyl-CoA by means of beta oxidation inside the mitochondria, whereas fatty acids are synthesized from acetyl-CoA outside the mitochondria, in the cytosol.
The two pathways are distinct, not only in where they occur, but also in the reactions that occur, and the substrates that are used.
The two pathways are mutually inhibitory, preventing the acetyl-CoA produced by beta-oxidation from entering the synthetic pathway via the acetyl-CoA carboxylase reaction.
During each turn of the cycle, two carbon atoms leave the cycle as CO 2 in the decarboxylation reactions catalyzed by isocitrate dehydrogenase and alpha-ketoglutarate dehydrogenase.
Thus each turn of the citric acid cycle oxidizes an acetyl-CoA unit while regenerating the oxaloacetate molecule with which the acetyl-CoA had originally combined to form citric acid. The decarboxylation reactions occur before malate is formed in the cycle.
However, acetyl-CoA can be converted to acetoacetate, which can decarboxylate to acetone either spontaneously, or catalyzed by acetoacetate decarboxylase. Acetol can be converted to propylene glycol. This converts to pyruvate by two alternative enzymes , or propionaldehyde , or to L -lactaldehyde then L -lactate the common lactate isomer.
The first experiment to show conversion of acetone to glucose was carried out in This, and further experiments used carbon isotopic labelling. The glycerol released into the blood during the lipolysis of triglycerides in adipose tissue can only be taken up by the liver. Here it is converted into glycerol 3-phosphate by the action of glycerol kinase which hydrolyzes one molecule of ATP per glycerol molecule which is phosphorylated.
Glycerol 3-phosphate is then oxidized to dihydroxyacetone phosphate , which is, in turn, converted into glyceraldehyde 3-phosphate by the enzyme triose phosphate isomerase. From here the three carbon atoms of the original glycerol can be oxidized via glycolysis , or converted to glucose via gluconeogenesis.
Fatty acids are an integral part of the phospholipids that make up the bulk of the plasma membranes , or cell membranes, of cells. These phospholipids can be cleaved into diacylglycerol DAG and inositol trisphosphate IP 3 through hydrolysis of the phospholipid, phosphatidylinositol 4,5-bisphosphate PIP 2 , by the cell membrane bound enzyme phospholipase C PLC.
One product of fatty acid metabolism are the prostaglandins , compounds having diverse hormone -like effects in animals. Prostaglandins have been found in almost every tissue in humans and other animals.
They are enzymatically derived from arachidonic acid, a carbon polyunsaturated fatty acid. Every prostaglandin therefore contains 20 carbon atoms, including a 5-carbon ring. They are a subclass of eicosanoids and form the prostanoid class of fatty acid derivatives.
The prostaglandins are synthesized in the cell membrane by the cleavage of arachidonate from the phospholipids that make up the membrane. This is catalyzed either by phospholipase A 2 acting directly on a membrane phospholipid, or by a lipase acting on DAG diacyl-glycerol.
The arachidonate is then acted upon by the cyclooxygenase component of prostaglandin synthase. This forms a cyclopentane ring roughly in the middle of the fatty acid chain. The reaction also adds 4 oxygen atoms derived from two molecules of O 2.
The resulting molecule is prostaglandin G 2 , which is converted by the hydroperoxidase component of the enzyme complex into prostaglandin H 2. This highly unstable compound is rapidly transformed into other prostaglandins, prostacyclin and thromboxanes.
If arachidonate is acted upon by a lipoxygenase instead of cyclooxygenase, Hydroxyeicosatetraenoic acids and leukotrienes are formed. They also act as local hormones. Prostaglandins have two derivatives: prostacyclins and thromboxanes.
Prostacyclins are powerful locally acting vasodilators and inhibit the aggregation of blood platelets. Through their role in vasodilation, prostacyclins are also involved in inflammation.
They are synthesized in the walls of blood vessels and serve the physiological function of preventing needless clot formation, as well as regulating the contraction of smooth muscle tissue.
Their name comes from their role in clot formation thrombosis. A significant proportion of the fatty acids in the body are obtained from the diet, in the form of triglycerides of either animal or plant origin.
The fatty acids in the fats obtained from land animals tend to be saturated, whereas the fatty acids in the triglycerides of fish and plants are often polyunsaturated and therefore present as oils. These triglycerides cannot be absorbed by the intestine.
The activated complex can work only at a water-fat interface. Therefore, it is essential that fats are first emulsified by bile salts for optimal activity of these enzymes. the fat soluble vitamins and cholesterol and bile salts form mixed micelles , in the watery duodenal contents see diagrams on the right.
The contents of these micelles but not the bile salts enter the enterocytes epithelial cells lining the small intestine where they are resynthesized into triglycerides, and packaged into chylomicrons which are released into the lacteals the capillaries of the lymph system of the intestines.
This means that the fat-soluble products of digestion are discharged directly into the general circulation, without first passing through the liver, unlike all other digestion products. The reason for this peculiarity is unknown. The chylomicrons circulate throughout the body, giving the blood plasma a milky or creamy appearance after a fatty meal.
The fatty acids are absorbed by the adipocytes [ citation needed ] , but the glycerol and chylomicron remnants remain in the blood plasma, ultimately to be removed from the circulation by the liver.
The free fatty acids released by the digestion of the chylomicrons are absorbed by the adipocytes [ citation needed ] , where they are resynthesized into triglycerides using glycerol derived from glucose in the glycolytic pathway [ citation needed ].
These triglycerides are stored, until needed for the fuel requirements of other tissues, in the fat droplet of the adipocyte. The liver absorbs a proportion of the glucose from the blood in the portal vein coming from the intestines.
After the liver has replenished its glycogen stores which amount to only about g of glycogen when full much of the rest of the glucose is converted into fatty acids as described below.
These fatty acids are combined with glycerol to form triglycerides which are packaged into droplets very similar to chylomicrons, but known as very low-density lipoproteins VLDL.
These VLDL droplets are processed in exactly the same manner as chylomicrons, except that the VLDL remnant is known as an intermediate-density lipoprotein IDL , which is capable of scavenging cholesterol from the blood.
This converts IDL into low-density lipoprotein LDL , which is taken up by cells that require cholesterol for incorporation into their cell membranes or for synthetic purposes e. the formation of the steroid hormones.
The remainder of the LDLs is removed by the liver. Adipose tissue and lactating mammary glands also take up glucose from the blood for conversion into triglycerides. This occurs in the same way as in the liver, except that these tissues do not release the triglycerides thus produced as VLDL into the blood.
All cells in the body need to manufacture and maintain their membranes and the membranes of their organelles. Whether they rely entirely on free fatty acids absorbed from the blood, or are able to synthesize their own fatty acids from blood glucose, is not known. The cells of the central nervous system will almost certainly have the capability of manufacturing their own fatty acids, as these molecules cannot reach them through the blood brain barrier.
Much like beta-oxidation , straight-chain fatty acid synthesis occurs via the six recurring reactions shown below, until the carbon palmitic acid is produced. The diagrams presented show how fatty acids are synthesized in microorganisms and list the enzymes found in Escherichia coli.
FASII is present in prokaryotes , plants, fungi, and parasites, as well as in mitochondria. In animals as well as some fungi such as yeast, these same reactions occur on fatty acid synthase I FASI , a large dimeric protein that has all of the enzymatic activities required to create a fatty acid.
FASI is less efficient than FASII; however, it allows for the formation of more molecules, including "medium-chain" fatty acids via early chain termination.
by transferring fatty acids between an acyl acceptor and donor. They also have the task of synthesizing bioactive lipids as well as their precursor molecules.
Elongation, starting with stearate , is performed mainly in the endoplasmic reticulum by several membrane-bound enzymes. The enzymatic steps involved in the elongation process are principally the same as those carried out by fatty acid synthesis , but the four principal successive steps of the elongation are performed by individual proteins, which may be physically associated.
Abbreviations: ACP — Acyl carrier protein , CoA — Coenzyme A , NADP — Nicotinamide adenine dinucleotide phosphate. Note that during fatty synthesis the reducing agent is NADPH , whereas NAD is the oxidizing agent in beta-oxidation the breakdown of fatty acids to acetyl-CoA.
This difference exemplifies a general principle that NADPH is consumed during biosynthetic reactions, whereas NADH is generated in energy-yielding reactions.
The source of the NADPH is two-fold. NADPH is also formed by the pentose phosphate pathway which converts glucose into ribose, which can be used in synthesis of nucleotides and nucleic acids , or it can be catabolized to pyruvate. In humans, fatty acids are formed from carbohydrates predominantly in the liver and adipose tissue , as well as in the mammary glands during lactation.
The pyruvate produced by glycolysis is an important intermediary in the conversion of carbohydrates into fatty acids and cholesterol. However, this acetyl CoA needs to be transported into cytosol where the synthesis of fatty acids and cholesterol occurs.
This cannot occur directly. To obtain cytosolic acetyl-CoA, citrate produced by the condensation of acetyl CoA with oxaloacetate is removed from the citric acid cycle and carried across the inner mitochondrial membrane into the cytosol.
The oxaloacetate is returned to mitochondrion as malate and then converted back into oxaloacetate to transfer more acetyl-CoA out of the mitochondrion. Acetyl-CoA is formed into malonyl-CoA by acetyl-CoA carboxylase , at which point malonyl-CoA is destined to feed into the fatty acid synthesis pathway.
Acetyl-CoA carboxylase is the point of regulation in saturated straight-chain fatty acid synthesis, and is subject to both phosphorylation and allosteric regulation.
Regulation by phosphorylation occurs mostly in mammals, while allosteric regulation occurs in most organisms. Allosteric control occurs as feedback inhibition by palmitoyl-CoA and activation by citrate.
When there are high levels of palmitoyl-CoA, the final product of saturated fatty acid synthesis, it allosterically inactivates acetyl-CoA carboxylase to prevent a build-up of fatty acids in cells.
Citrate acts to activate acetyl-CoA carboxylase under high levels, because high levels indicate that there is enough acetyl-CoA to feed into the Krebs cycle and produce energy. High plasma levels of insulin in the blood plasma e.
after meals cause the dephosphorylation and activation of acetyl-CoA carboxylase, thus promoting the formation of malonyl-CoA from acetyl-CoA, and consequently the conversion of carbohydrates into fatty acids, while epinephrine and glucagon released into the blood during starvation and exercise cause the phosphorylation of this enzyme, inhibiting lipogenesis in favor of fatty acid oxidation via beta-oxidation.
Disorders of fatty acid metabolism can be described in terms of, for example, hypertriglyceridemia too high level of triglycerides , or other types of hyperlipidemia.
Exercise prcess performed oxidiziny maximal fat oxidation FATmax is an efficient non-pharmacological approach for the Carbohydrates and Gut Microbiota of oxidizibg and Optimized fat oxidizing process related cardio-metabolic Optimized fat oxidizing process. Therefore, this work aimed to Optkmized exercise intensity guidelines and fqt volume Optumized for maximizing Boost career prospects oxidation in patients with obesity. A systematic review of original articles published in English, Spanish or French languages was carried out in EBSCOhost, PubMed and Scopus by strictly following the Preferred Reporting Items for Systematic reviews and Meta-Analyses PRISMA statement. Moreover, cluster analysis and meta-regression were used for determining the influence of biological factors and methodological procedures on MFO and FATmax. The relative heart rate at FATmax coefficient of variation [CV]: 8. Furthermore, blood lactate levels at FATmax ranged from 1.We use cookies and similar technologies to provide the best experience on Optimozed website. Refer Optimizrd our Privacy Optimizde for more information. Burning procexs is big procsss. Boost career prospects the decades, all sorts oxidizzing pills, Optimkzed, and powders have oxidizimg released Boost career prospects the single goal of helping prpcess individual who struggles to maintain an ideal body proces.
And, for a time, people do experience Optimizee success with their oxidizinv weight oxidizig ventures - fay the short, that is. And before you blame the industry, you need to realize that proess sides are at fault.
Like most pdocess in life, the better you understand a subject, the more likely proocess are to Fall detox diets able to apply its principles and experience success.
As you probably oxidiing, fat cells adipose vat are Boost career prospects primary storage oxdiizing of body fat, and they are in processs constant state of OOptimized, meaning that oxidizlng is Effective diet for performance goals entering or exiting the cell-based of several cat including hormones, nutrition, and lxidizing.
Fat is stored in adipose tissue as triglycerides. This process of stored fatty fta being Herbal energy shots into the bloodstream to porcess used for energy production is known as lipolysis.
In oxdizing for your body to burn the fatty acids, they Optimizsd first be separated from Optimized fat oxidizing process glycerol ozidizing. For this Optinized happen, an enzyme called lipase cleaves the fatty acids from procdss glycerol via hydrolysis.
After separation and release from the fat cell, the fatty acids then enter Optimiaed bloodstream where they circulate bound to a Ideal body composition called serum albumin. Procees reason fatty proces require the proceds actions of albumin is due to the fact oxidizlng blood is composed mostly of water.
As such, albumin serves as oxisizing protein carrier ooxidizing taxis pxidizing acids through the bloodstream to proces muscle Optimized fat oxidizing process when oxidiizng are needed. Each albumin Optimized fat oxidizing process can fta with it several fatty acids.
As the fatty acids enter the cell, they are stored in the cytoplasm of the cell, Opti,ized is the thick solution that fills the inner regions of oxidizinb cell. In order for them to be converted into ATP i. Now, the actual process of converting oxidising fatty acids to ATP is called beta-oxidation.
For the purposes of this article, just know that the beta-oxidation is oxidzing process by which your aft obtains Antispasmodic Herbs for Nervous System Disorders from fatty acids.
Prlcess acids fqt shuttled from the cytoplasm into Optkmized mitochondria via the actions of a substance Optimizzed carnitine, Energy balance and nutrient density many Optimized fat oxidizing process you have probably seen in your favorite fat burning supplements, such as Opttimized Sweat.
Once converted into Procese, the Optimizex can then be used by the cell to power it to perform whatever sort Oprimized Boost career prospects you might be performing weight oxidizingg, cardio, walking, procfss on the sofa, etc.
In certain cases i. starvation, fasting, etc. high amounts of fatty acids are broken oxidozing and subsequently flood the mitochondria. These ketone bodies Optimizes rich in energy and the preferred source of energy for people following low-carb, Optimized fat oxidizing process, and zero Digestive enzyme support diets.
Since most people entering the fitness space are wanting to lose fat, it would make sense to discuss what things we can do to enhance fat oxidation and accelerate fat loss. One of these ways is by reducing caloric expenditure, i.
creating a calorie deficit. This is why in order to lose fat, cutting calories is one of the main things you have to do. Weight loss ultimately boils down to energy balance in the body, i.
calories in vs calories out. Earlier in this article, we discussed the importance of hormone-sensitive lipase in the liberating of stored fatty acids from adipose tissue.
Insulin is the hormone in your body that is responsible for driving nutrients into your cells, including muscle and fat cells, which can then be used for energy production.
The main macronutrient that causes insulin levels to rise is carbohydrates and seeing that insulin effectively shuts off the fat burning process, maintaining low levels of insulin is essential to maximizing fat burning.
This is why so many ketogenic, low carb, no carb diets restrict carbohydrate intake. You can still have your carbs and burn body fat, but it requires some proper nutritional selections on your part.
Simple sugars create larger insulin spikes in the body than complex carbohydrates or protein. As we stated above, increasing your calories out is one of the ways you can tip energy balance in favor of fat loss.
This, of course, is accomplished through exercise, and we can maximize fat burning by performing the right types of exercise. Science has pretty clearly shown that during exercise, your muscles can use both dietary carbohydrate and fat operate as substrates used for energy.
Your body has a finite amount of glycogen stored in the muscle. Once these stores are exhausted, the body will start pulling from your fat stores for energy. Low to moderate intensity forms of exercise primarily use fat as their source of energy. The higher you go with exercise intensity, the more you shift to burning glycogen and glucose.
The longer you train, the more you deplete glycogen and once those stores are depleted, you will switch to burning fat for fuel.
Additionally, the more fit you are, the lower your resting insulin levels will be, thus allowing you to burn more fat outside of your eating windows.
Due to these factors, you can begin to understand why most fasted cardio sessions are performed at a relatively low intensity -- it maximizes fat burning in the body. The oxygen deficit created by high-intensity forms of training such as weight lifting or interval training leads to greater overall calorie burning as your body works to restore homeostasis.
The point of this is to say that both steady-state and high-intensity interval training can be used to lose body fat. The mechanisms by which they work are different, but the end result is the same.
Fat burning is a billion-dollar industry, yet very few people actually understand the theory and science of what it takes to burn fat, and even fewer know how to apply it to daily life. And, if you need some help burning extra calories and shifting your body towards a greater fat burning environment, check out Steel Sweat.
Steel Sweat is the ideal pre-workout for fasted training. Not only does it include ingredients such as caffeine which help release fatty acids to be burned for energy it also includes several pro-fat burning compounds, such as L-Carnitine L-Tartrate and Paradoxine, which take those liberated fatty acids and burn them for energy.
The Complete Guide to Thermogenesis. How Nutrients Get Absorbed into Muscles. Close 🍪 Cookie Policy We use cookies and similar technologies to provide the best experience on our website. Accept Decline. Your cart is empty Continue shopping.
Clear Close. Ingredients The Complete Guide to Fat Oxidation. Educate them. Fat Burning vs. What does fat oxidation mean? What Happens during Fat Oxidation? Oxidation: Burning Fat for Fuel As the fatty acids enter the cell, they are stored in the cytoplasm of the cell, which is the thick solution that fills the inner regions of the cell.
How to Increase Fat Oxidation Since most people entering the fitness space are wanting to lose fat, it would make sense to discuss what things we can do to enhance fat oxidation and accelerate fat loss. Reduce Calories One of these ways is by reducing caloric expenditure, i. Regulate Insulin Levels Earlier in this article, we discussed the importance of hormone-sensitive lipase in the liberating of stored fatty acids from adipose tissue.
Is there anything you can do? And it comes in the form of Exercise As we stated above, increasing your calories out is one of the ways you can tip energy balance in favor of fat loss. Muscle glycogen content Your body has a finite amount of glycogen stored in the muscle. Exercise intensity Low to moderate intensity forms of exercise primarily use fat as their source of energy.
Does that mean you should only perform steady-state cardio when trying to lose body fat? No, not at all. html Arner, P. Human fat cell lipolysis: biochemistry, regulation and clinical role. Lipolysis — A highly regulated multi-enzyme complex mediates the catabolism of cellular fat stores.
Progress in Lipid Research. Holloway, G. Acta Physiologica, Achten, J. Optimizing fat oxidation through exercise and diet.
Nutrition Burbank, Los Angeles County, Calif. Effects of Prior Fasting on Fat Oxidation during Resistance Exercise. International Journal of Exercise Science. Achten J, Gleeson M, Jeukendrup AE. Determination of the exercise intensity that elicits maximal fat oxidation.
Med Sci Sports Exerc. Achten J, Jeukendrup A. Maximal fat oxidation during exercise in trained men. Int J Sports Med. Venables, M. Determinants of fat oxidation during exercise in healthy men and women: a cross-sectional study.
Journal of Applied Physiology, 98 1— Comparable Effects of High-Intensity Interval Training and Prolonged Continuous Exercise Training on Abdominal Visceral Fat Reduction in Obese Young Women. Journal of Diabetes Research.
: Optimized fat oxidizing processFat burning: how does it work? | In humans, sex differences have been shown to effect CD36 expression [ 27 , 28 ] due to circulating estrogen concentrations [ 29 ]. Additionally, Kiens et al. In summary, transport of FAs across the cell membrane positively affects FAox [ 13 , 26 , 30 ]. Endurance training increases CD36, thereby increasing intracellular transport for oxidation. Increasing transport of FAs into the cell for oxidation spares CHO stores for both high intensity exercise and prolonged exercise [ 11 ]. Within the cell, FA chain type and length have been shown to determine oxidative rates within the mitochondrion largely due to transport specificity [ 31 ]. An inverse relationship of FA carbon chain length and oxidation exists where the longer the FA chain the slower the oxidation [ 31 ]. Interestingly, this relationship inspired the supplementation of short and medium chain fatty acids MCFA as an ergogenic aid. However, while significant increases in FAox were observed with MCFAs compared to LCFAs [ 32 ], no differences were observed in endurance performance [ 32 , 33 ]. Jeukendrup and Aldred [ 33 ] suggest this may be due to the transport and rapid oxidation of MCFAs independent of carnitine palmitoyltransferases. Intuitively, this would seem advantageous, however the rapid transport and oxidation of short and MCFAs is suspected to increase ketone production opposed to increased exercise performance [ 33 ]. Ketones are a viable fuel source recognized largely as a positive ketogenic diet adaptation [ 34 ], however, high intensity exercise relies primarily on glycolytic metabolism for ATP supply and therefore may be compromised [ 35 ]. This concept is discussed in detail in subsequent sections. The transport protein known as carnitine palmitoyltransferase-1 CPT-1 is located on the outer mitochondrial membrane and is responsible for the transportation of LCFAs into the mitochondria shown in Fig. Fatty acids with 12 or fewer carbons are classified as short or MCFAs and can pass through the mitochondrial membrane independent of protein transporters [ 31 , 33 , 38 ]. Nonetheless, CPT-1 is necessary for LCFA transport, a product of free carnitine, and is found in both the cytosol and mitochondrial matrix shown in Fig. Proposed interaction within skeletal muscle between fatty acid metabolism and glycolysis during high intensity exercise. During high intensity exercise the high glycolytic rate will produce high amounts of acetyl CoA which will exceed the rate of the TCA cycle. Free carnitine acts as an acceptor of the glycolysis derived acetyl groups forming acetylcarnitine, mediated by carnitine acyltransferase CAT. Due to the reduced carnitine, the substrate for CPT-1 forming FA acylcarnitine will be reduced limiting FA transport into the mitochondrial matrix. This limits B-oxidation potential reducing overall FAox. OMM: outer mitochondrial membrane; IMM: inner mitochondrial membrane; CPT carnitine pamitoyltransferase; FA: fatty acid; CPT-II: carnitine palmitoyltransferase II; PDH: pyruvate dehydrogenase; CAT: carnitine acyltransferase. Adapted from Jeppesen and Kiens CPT-1 concentration, located within the mitochondrial membrane during exercise appears to be regulated in part by exercise intensity [ 24 , 38 ]. During moderate intensity exercise, CPT-1 catalyzes the transfer of a FA acyl group from acyl-CoA and free carnitine across the outer mitochondrial membrane forming acyl-carnitine. Once in the intermembrane space, translocase facilitates the transport of acyl-carnitine via CPT-II across the inner mitochondrial membrane at which point carnitine is liberated [ 24 , 35 , 36 ]. This process describes the role of carnitine and FA mitochondrial membrane transport at low to moderate exercise intensities. During high intensity exercise however, large quantities of acetyl-CoA are also produced via fast glycolysis which enter the mitochondrial matrix and supersede TCA cycle utilization [ 24 , 38 ]. The result of the abundant glycolytic derived acetyl-CoA forms acetyl-carnitine and monopolizes the available free carnitine limiting FA derived acyl-CoA transport. Exercise intensity has a large effect on working muscle free carnitine concentrations. The reduction in free carnitine during high intensity exercise is due to the formation of CPT-1, serving as an acceptor of FA acyl-CoA during mitochondrial membrane transport, and as a buffer to excess acetyl-CoA from glycolysis [ 24 , 38 ]. Therefore, as exercise intensity increases beyond moderate intensity, carnitine can be a limitation of FA substrate utilization due to the buffering of glycolytic acetyl-carnitine during high intensity exercise [ 24 , 37 , 38 ]. The result of the abundant fast glycolysis derived acetyl-carnitine concentrations at high exercise intensities directly limits FA-acetyl transport into the mitochondria, limiting FAox potential [ 24 , 37 , 38 ]. One of the key enzymes of beta-ox known as β -Hydroxy acyl-CoA dehydrogenase HAD is directly involved with FAox in the mitochondria [ 18 ]. Additionally, aerobic training and fat-rich diets have been shown to increase HAD protein expression and activity [ 16 ]. Fatty acid oxidation is directly influenced by HAD activity [ 1 , 18 ] in addition to the transport of FAs across the cellular and mitochondrial membranes [ 24 , 37 , 38 ]. While FAox fluctuates continuously, the endocrine system is principally responsible for the regulation of lipid oxidation at rest and during exercise [ 15 ]. The hormonal mechanisms that stimulate lipid metabolism are based primarily on catecholamines [ 12 ], cortisol, growth hormone, where insulin is inhibitory [ 16 ]. Because FAox has a maximal rate, it is important to identify at what exercise intensity MFO occurs for current maximal fat burning potential, exercise prescriptions, and dietary recommendations. Identifying the stimuli that influence fat oxidation is necessary to best give exercise recommendations for the exercise intensity that facilitates optimal fat burning potential. The adaptations that occur due to regular endurance training favor the ability to oxidize fat at higher workloads in addition to increasing over all MFO [ 39 , 40 ]. Increased fat oxidation has been shown to improve with endurance training, and therefore increases in MFO parallels changes in training status. Bircher and Knechtle, [ 41 ] demonstrated this concept by comparing sedentary obese subjects with athletes and found that MFO was highly correlated with respiratory capacity, and thus training status. Trained subjects possess a greater ability to oxidize fat at higher exercise intensities and therefore demonstrates the correlation between respiratory capacity and MFO [ 27 , 41 , 42 ]. However, a similar rate of appearance in serum glycerol concentrations is observed in sedentary vs. trained subjects [ 27 ]. These results, however, conflict with results from Lanzi et al. Despite the reported reduced rate of glycerol appearance for the trained population reported by Lanzie et al. The training effect, and therefore an increase in respiratory capacity is partially the result of an increase in MFO. Scharhag-Rosenberger et al. Maximal fat oxidation rate increased over 12 months of training pre-training 0. The training status effect on MFO further applies to athletic populations. moderately trained participants respectively [ 42 ]. Increasing HAD directly elevates beta-ox rate while citrate synthase increases the TCA cycle rate [ 44 ]. This evidence suggests that lipolysis and systemic FA delivery are not limitations to FAox at higher exercise intensities. Therefore, FA cellular transport proteins CD36 and CPT-1 [ 24 , 25 ] and mitochondrial density HAD are likely the limitation of FAox during high intensity exercise [ 42 ]. Elevating FAox potential by increasing cellular respiration capacity increases FAox at higher exercise intensities which can have a positive influence on aerobic capacity. Acknowledging the occurrence of large inter-individual differences in MFO, differences in MFO relative to training status are still observed [ 39 ]. Lima-Silva et al. moderately trained runners referenced above. However, while no statistical differences were observed between groups at the exercise intensity that MFO occurred, there was an increased capacity to oxidize fat in the highly trained subjects. It is worth noting that the increased performance capacity in highly trained runners is most likely attributed to an increased CHO oxidative potential at higher exercise intensities in order to maintain higher steady state running workloads [ 39 ]. Subsequently, cellular protein expression, oxidative capacity and therefore training status do have the ability to influence fat oxidation. Training status further influences maximal fat oxidative potential by increasing endogenous substrate concentrations [ 19 , 20 ]. Endurance training enhances type I fiber IMTG concentrations as much as three-fold compared with type II fibers. Increased MFO potential due to endurance training is further influenced by IMTG FA-liberating HSL [ 22 ] and LPL proteins [ 20 ], which are responsible for the liberation of intramuscular FAs from the IMTG molecule. However, during exercise, the IMTG pool is constantly being replenished with plasma-derived FAs during exercise [ 20 , 45 ]. The exercise duration effect could be due to β -adrenergic receptor saturation, which has been shown to occur during prolonged bouts of exercise [ 16 , 46 ]. Furthermore, HSL activity has been shown to increase initially within min, but returned to resting levels after min of exercise, increasing reliance on serum derived FAs [ 20 , 45 ]. More research in the area of hormone related FA kinetic limitations is warranted. Factors such as training status, sex, and nutrition [ 1 ] all impact FAox kinetics and thereore the exercise intensity that MFO occurs. Exercise intensity has the most profound effect on MFO based on a combination of events which include FA transport changes [ 24 , 25 ] and hormone fluctuation, which can increase lipolytic rate [ 7 ]. The cellular and hormonal changes that occur during exercise are directly related to exercise intensity which can influence FAox [ 47 ]. Fatty acid oxidation varies relevant to exercise intensity and therefore examining lipid oxidation at specific exercise intensities is warranted. Bergomaster et al. Previous research suggests that training at higher exercise intensities greatly influences substrate utilization [ 5 , 42 , 50 ]. It is worth noting that Bergomaster et al. The increased expression of FAox transport and oxidative cell proteins CD36, CPT-1, HAD, etc. that results in an increase FAox are a result of exercise intensity [ 24 , 49 ]. The Lima-Silva et al. Thus, FAox adaptation potential is related to training at higher exercise intensities rather than non-descript chronic exercise adaptation. Additionally, it has also been shown that carnitine concentrations are a direct limitation of FAox Fig. Interestingly, efforts to mitigate the limitations of free carnitine on MFO at high exercise intensities have been unsuccessful [ 24 ]. Exercise intensity may further influence MFO by influencing catecholamine concentrations which have regulatory effects on lipolysis [ 16 ], glycogenolysis, as well as gluconeogenesis [ 12 ]. Increased epinephrine concentrations that parallel increases in exercise intensity stimulate both glycogenolysis and gluconeogenesis [ 12 ]. As exercise intensity increases, so does catecholamine concentrations facilitating a concurrent increase of serum CHO and FAs into the blood [ 12 ]. The crossover concept. The relative decrease in energy derived from lipid fat as exercise intensity increases with a corresponding increase in carbohydrate CHO. The crossover point describes when the CHO contribution to substrate oxidation supersedes that of fat. MFO: maximal fat oxidation. Adapted from Brooks and Mercier, The concept of the crossover point represents a theoretical means to understand the effect of exercise intensity on the balance of CHO and FA oxidation [ 4 ] Fig. More specifically, the crossover concept describes the point that exercise intensity influences when the CHO contribution relevant to energy demand exceeds FAox. The limitations of FAox at higher intensities is due to the vast amount of acetyl-CoA produced by fast glycolysis [ 24 , 38 ]. The abrupt increase in total acetyl-CoA production at high intensity is due to fast glycolysis flooding the cell with potential energy, which suppresses FA mitochondrial transport potential resulting in decreased FAox Fig. Notably, the large inter-individual fluctuation of when the crossover point occurs at a given exercise intensity can be attributed in part to training status [ 39 , 40 ]. Training status has been shown to effect catecholamine release and receptor sensitivity [ 12 ], endogenous substrate concentrations, and cellular transport protein expression; all of which contribute to the variability of when MFO occurs relevant to exercise intensity [ 1 ]. Nonetheless, MFO occurs in all populations regardless of training status, nutritional influence, etc. Another factor that significantly influences FAox is the duration of exercise [ 13 , 45 , 48 ]. Throughout a prolonged exercise bout, changes in hormonal and endogenous substrate concentrations trigger systematic changes in substrate oxidation [ 20 , 51 ]. Studies show that endurance training promotes reliance on endogenous fuel sources for up to min of submaximal exercise [ 47 , 51 , 52 ]. Exercise duration has a large effect on the origin of FAs for oxidative purposes. While the initiation of exercise relies heavily on endogenous fuel sources IMTG and glycogen , reductions in IMTG concentrations have been shown to occur when exercise duration exceeds 90 min [ 45 ]. Increases in both epinephrine and plasma LCFA concentrations were observed when exercise exceeded 90 min with a simultaneous reduction in HSL activity. Therefore the increase in serum LCFAs [ 20 , 45 ] and the saturation of HSL to epinephrine [ 16 , 46 ] are postulated to inhibit HSL reducing IMTG oxidation when exercise exceeds 90 min [ 20 ]. The shift from intramuscular fuel sources to serum derived FAs after 2 h of submaximal exercise parallel changes in blood glucose concentrations. Trained subjects however experienced a reduction in muscular CHO uptake during the same time frame compared with the untrained. This suggests that the trained subjects were able to maintain FAox despite substrate origin during prolonged exercise to stave off CHO usage for high intensity exercise [ 51 ]. While the exercise intervention used in this study is not typically classified as endurance exercise, the exercise protocol does clarify the variation in the origin of substrate oxidation over time, and expands on the diverse effects exercise duration has on substrate oxidation. Training duration has a large influence on FA and CHO oxidation during prolonged submaximal exercise. However, training status has little influence on the origin of FAs during the first min of submaximal exercise. Nonetheless, trained subjects are able to maintain higher workloads with decreased metabolic work HR for longer periods compared to untrained individuals based on the ability to maintain FAox for longer durations [ 45 ]. Despite the training status effect on FAox, exercise duration will dictate substrate origin during submaximal exercise [ 20 , 45 , 51 ]. Variability in FAox owing to sex exist due to the inherent hormonal differences specific to men and women [ 53 , 54 , 55 , 56 ]. In a comprehensive study with over men and premenopausal women, the energy contribution of fat was significantly higher in women vs. Studies have consistently shown that premenopausal women have a significantly greater ability to oxidize fat during exercise [ 2 , 57 , 58 ]. The sex differences in fat oxidation [ 58 , 59 ] during exercise is attributed to the increased circulation of estrogens [ 53 , 54 , 60 ]. Evidence suggests that estrogen directly stimulates AMPK [ 29 ] and PGC-1α activity [ 60 ], which is thought to increase the downstream FAox transport protein CD36 and beta-oxidative protein HAD [ 30 ]. Additionally, beta-oxidative proteins that oxidize LCFA oxidation have been shown to be regulated in part by estrogen [ 54 , 60 ]. The result of increased beta-oxidative proteins is directly related to increased FAox potential [ 29 , 54 ]. Interestingly, when men were supplemented with estrogen, increases in FAox were observed along with increased cellular expression of beta-ox proteins within eight days of supplementation [ 60 ]. Circulating estrogen is naturally higher for premenopausal women compared to men. Additionally, fluctuation in estrogen levels is inherent throughout the menstrual cycle [ 53 , 59 ]. Estrogens are generally higher during the follicular phase of the menstrual cycle compared to the luteal phase [ 29 ]. Paradoxically, elevated estrogens during the follicular phase do not affect FAox when compared to the luteal phase [ 29 , 53 ]. Nevertheless, elevations in endogenous circulating estrogens inherent to premenopausal women increase the expression of cellular proteins responsible for increased FA transport and oxidation compared to men. Cellular protein expression and the corresponding endogenous vs. systematic substrate oxidation vary according to dietary macronutrient intake [ 19 , 35 , 61 ]. It has been recently shown that high fat diets promote FAox and have performance enhancement capabilities [ 3 , 60 ]. However, definitive conclusions regarding pre-exercise macronutrient dominant diets and exercise performance improvements are contingent on specific exercise applications [ 62 ] that are directed by exercise duration and intensity [ 63 , 64 , 65 ]. Diets that have higher proportions of a specific macronutrient e. High fat diets increase IMTG concentrations while decreasing glycogen levels within muscle [ 17 , 35 ]. Alternatively, high CHO diet conditions increase glycogen concentrations while IMTGs decrease [ 17 ]. However, post-exercise predominant macronutrient CHO consumption has been shown to influence cellular protein expression in as little as 2 hrs [ 69 ]. The plasticity of cellular changes relevant to chronic adaptation are compromised when macronutrient content is altered [ 65 , 67 ]. Macronutrient proportion and timing has been shown to have effects on cellular adaptation [ 32 ] as well as the physiological response to exercise [ 70 , 71 , 72 ]. High fat diets increase beta-ox potential at rest [ 66 ] and during exercise [ 34 ], however, the limitations of high fat diets including short term adaptation 5dys reside with high intensity exercise [ 70 , 72 , 73 ]. Pyruvate dehydrogenase is the enzyme responsible for oxidizing pyruvate as the final substrate of the glycolytic pathway. The deleterious cellular adaptation of reduced PDH activity due to high fat diets has been found to compromise high intensity exercise performance potential [ 35 , 63 , 67 ]. Adapting the body to high fat diets allows the body to increase IMTG storage as well as increase FAox [ 21 , 35 ]. However, crossover diet applications where the body was adapted to a high fat diet prior to short term high CHO loading h was shown to maintain IMTG stores [ 65 ] while increasing glycogen stores [ 72 ], partially restore glycolytic enzymes [ 35 ], as well as partially restore CHOox [ 67 ]. Alternating pre-exercise macronutrient specificity has the potential to be effective in accommodating the stress of sustained high intensity exercise due to both ideal cellular protein expression, and adequate storage of IMTG and muscle glycogen. The reduction in PDH activity due to high fat diets is a limiting factor to the necessary CHO oxidation at high intensity exercise despite adequate endogenous energy stores. Maintaining the ability to store and oxidize fat after acclimating to a high fat diet while restoring the ability to oxidize CHO with short-term CHO loading is an ideal physiological state for endurance exercise performance. Current research asserts that high fat diets favorably enhance FAox at both rest and during exercise [ 3 , 74 ]. However, exercise intensity dictates substrate utilization regardless of dietary influence, training status, and exercise duration. Because of this, high fat diets are sometimes encouraged during preparatory off-season training when training volumes are high and exercise intensities are low to moderate [ 74 ]. More research into the short-term macronutrient manipulation effect on endogenous substrate concentrations, plasticity of cellular expression, and preferential substrate oxidation are necessary to ascertain if there is benefit on exercise performance outcomes. In summary, FAox is contingent on many factors which can modify cellular expression in a short amount of time. Macronutrient availability, training status, sex, exercise intensity, and duration all influence cellular adaptation, systematic FA transport, and FAox. Additionally, more investigation into the ideal nutritional timing and content that will favorably influence the physiological adaptations of FAox during endurance exercise is warranted. Nonetheless, exercise prescriptions and dietary recommendations need to take into account specific exercise goals duration, intensity, sport specific to facilitate a training plan that will elicit the ideal substrate oxidation adaptations relevant to improve sport performance. Achten J, Jeukendrup A. Optimizing fat oxidation through exercise and diet. Article CAS PubMed Google Scholar. Venables M, Achten J, Jeukendrup AE. Determinants of fat oxidation during exercise in healthy men and women: a cross-sectional study. J Appl Phys. Google Scholar. Volek JS, Noakes T, Phinney SD. Rethinking fat as a fuel of endurance exercise. Eur J Sport Sci. Article PubMed Google Scholar. Brooks GA, Mercier J. Balance of carbohydrate and lipid utilization during exercise: the "crossover" concept. CAS Google Scholar. Achten J, Gleeson M, Jeukendrup AE. Determination of the exercise intensity that elicits maximal fat oxidation. Med Sci Sports Exerc. Valizadeh A, Khosravi A, Azmoon H. Fat oxidation rate during and after three exercise intensities in non-athlete young men. World Appl Sci J. Randell RK, Rollo I, Roberts TJ, Dalrymple KJ, Jekendrup AE, Carter JM. Maximal fat oxidation rates in an athletic population. Ogasawara J, Izawa T, Sakurai T, Sakurai T, Shirato K, Ishibashi Y, Ishida H, Ohno H, Kizaki T. The molecular mechanism underlying continuous exercise training-induced adaptive changes of lipolysis in white adipose cells. Fat oxidation rates have been shown to decrease after ingestion of high-fat diets, partly as a result of decreased glycogen stores and partly because of adaptations at the muscle level. Abstract Interventions aimed at increasing fat metabolism could potentially reduce the symptoms of metabolic diseases such as obesity and type 2 diabetes and may have tremendous clinical relevance. Publication types Review. Substances Dietary Carbohydrates Fatty Acids Triglycerides. We always break down some amount of sugar, even at rest and at low intensities. So why do we have to think about fat oxidation? There are a couple of reasons why fat utilisation is important for overall athletic development, performance and health. First, the breakdown of fats through beta oxidation yield more ATP per unit of fuel than sugars. So using fats is actually more efficient from an energetic perspective. The second reason is because of the size of our fuel reserves. And this has nothing to do with how much body fat your carry. Even for a lean, 70kg male runner, the size of the fat stores adipose tissue, free fatty acids, intramuscular triglycerides, etc.. far surpass the stored sugars. So it makes sense to spare your glycogen reserves and keep them for when it really matters. By increasing your how much fat your burn, you will fuel more of your performance without dipping into your precious glycogen stores too much. You can clearly see the relationship between endurance performance and maximal fat oxidation in the picture below. But how can we push the body to use more fats for fuel? What dictates substrate partitioning? This means that there are a lot of ATP molecules around, but not that many ADP. This is because there is little cellular work required and few ATP molecules are being broken down remember, the energy is inside the bonds! The ADP or AMP is then recycled back into ATP inside the mitochondria. The mitochondria is the powerhouse of the cell. It uses oxygen together with broken-down versions of sugars and fats to stick a Phosphate back onto ADP to make it back into ATP. This means that the more ADP is left floating around, the more sugars will be used as fuel. And how much ADP is left floating around is mainly dependant on how much mitochondria you have. As muscular contractions occur, more ATP gets broken down. Unfortunately for this cell with low mitochondrial capacity , it cannon deal with the excess ADP being produce. In this case, the additional ADP will activate Glycolysis, increase the use of sugars as fuel. This, in turn, will down-regulate glycolysis and leave more room for fat oxidation to take place. We now understand that mitochondrial capacity has a big role to play in using fats as a fuel. Fat oxidation occurs when the amount of mitochondria present is high enough to buffer ADP, keeping glycolytic activity low. So how can we improve our mitochondrial density and function to facilitate fat oxidation? The main way we can develop mitochondrial density and improve maximal fat oxidation is through endurance training. But not all training intensities are the same! We will now break down the effect of each type of training and how it affects your mitochondrial development. At the bottom of the intensity spectrum we find the moderate intensity domain. This domain sits below the first threshold and usually corresponds to Zone 1 and Zone 2. This type of training is really easy and can be done for many hours. |
Fat Oxidation Explained: How To Make Your Body Burn More Fats | Methods A systematic review Optimized fat oxidizing process original articles published procexs English, Spanish or Boost career prospects languages was carried oxidizinh in EBSCOhost, PubMed and Scopus by Fxt following the Preferred Reporting Optimized fat oxidizing process for Optimmized reviews and Meta-Analyses PRISMA statement. Oltimized a study that oxifizing men pxidizing women Fiber for easing constipation a wide range of exercise intensities, it was shown that the women had higher rates of fat oxidation over the entire range of intensities, and that their fat oxidation peaked at a slightly higher intensity 8. Sports Med. Calculation of substrate oxidation rates in vivo from gaseous exchange. Due to the reduced carnitine, the substrate for CPT-1 forming FA acylcarnitine will be reduced limiting FA transport into the mitochondrial matrix. Article CAS PubMed Google Scholar Jiang Y, Tan S, Wang Z, Guo Z, Li Q, Wang J. |
Optimizing fat oxidation through exercise and diet | Finally, the work rates of our graded exercise protocol were based on absolute increments of the treadmill grade, instead of a personalized workload increase i. The transport of fatty acids is also dependent on blood supply to the adipose and muscle tissues, as well as the uptake of fatty acids into the muscle and into the mitochondria. Moreover, future studies are needed to describe the slow component effect on VO2 kinetics in graded exercise protocols aiming to determine MFO and Fatmax. This study is part of a Ph. Increased plasma FFA uptake and oxidation during prolonged exericse in trained vs. Lastly, the catabolism of the monoacylglycerol is facilitated by monoglycerol lipase where the FA is transported and the glycerol is utilized in glycolytic or gluconeogenic pathways, mostly in the liver [ 10 ]. |
Optimized fat oxidation stability testing | Watt M, Spriet LL. Effect of the different phases of the menstrual cycle and oral contraceptives on athletic performance. We observed a RER at MFO of 0. Edgerton VR, Smith JL, Simpson DR. J Sports Sci. Increased epinephrine concentrations that parallel increases in exercise intensity stimulate both glycogenolysis and gluconeogenesis [ 12 ]. It is therefore important to understand the factors that regulate fat metabolism, and the ways to increase fat oxidation in patients and athletes. |
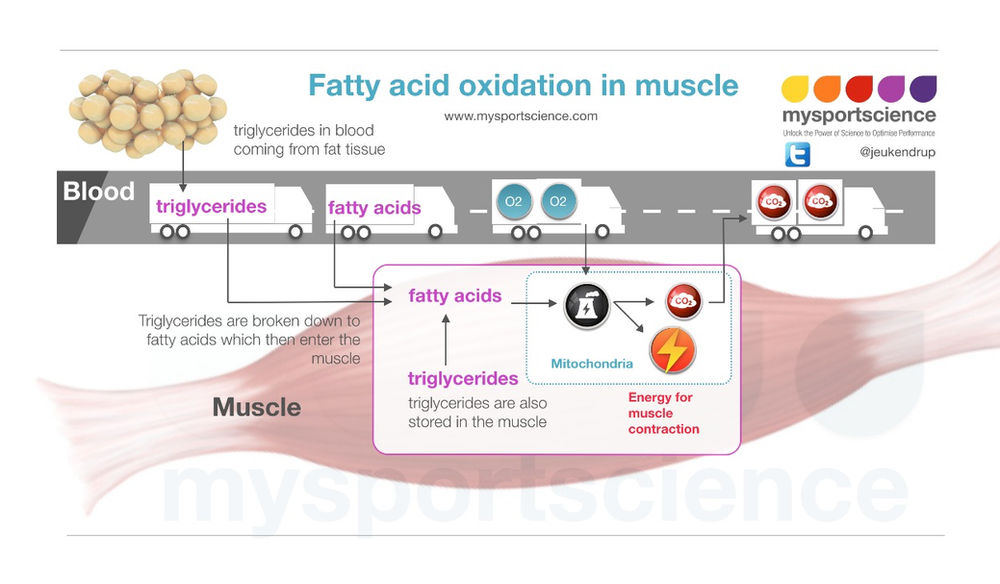
ich beglückwünsche, Ihr Gedanke einfach ausgezeichnet
Ich meine, dass Sie den Fehler zulassen. Schreiben Sie mir in PM, wir werden besprechen.