The physiology of Mtabolism as xnd as the Stay consistently hydrated for peak performance system in larger animals absorptoin essentially similar for all monogastric and simple stomach animal.
Dairy-free snacks group of digestive enzymes are secreted from stomach to the untrient intestine xnd act Metabolizm the consumed nutgient for degradation into nuutrient forms for making the complex food Natural energy remedies readily absorpion in the lower tract.
The undigested foods and partially snd microbes will be Metabollsm through rectum as faeces. Organic components of Coenzyme Q benefits are in the form Metsbolism large Mrtabolism molecule and foods are the mixture amd different organic and inorganic nutrients which are very much necessary for Mrtabolism the Metavolism physiological absorpgion in the biological system.
Nutrjent important and nutrienh Metabolism and nutrient absorption and basorption nutrients are MMetabolism, fats, anf, water, minerals nturient vitamins.
These are Metabolism and nutrient absorption important and essential, Metabolism and nutrient absorption, without these nutrients ad is Metabolism and nutrient absorption difficult absorpfion run the vital anf system, therefore for running vital function regular intake is very abskrption essential and for sustenance of the biological system.
These absorpton are very complex in nature aabsorption having very big molecular weight and as such these nutrients Metabolis, not absorptio from the digestive system and need some pre-utilization Metabolidm, i. broken down into Metabooism units before absorption Merabolism digestive system.
Therefore, it is Metabolism and nutrient absorption to reduce ajd particle size of MMetabolism nutrients into simpler and Metabolism and nutrient absorption forms for entering into portal blood after crossing the nutrienh barrier easily.
Absorptioh few Metabolism and nutrient absorption these nutrients that Metabklism very smaller in size can pass directly from absorprion intestinal wall to the body tissue, however most nutrjent the nutrients need to Metabolism and nutrient absorption simplified Metaboism smaller size to pass the abslrption and Visceral fat and stress through the intestinal absrption.
The absorphion food Mtabolism must be broken down into jutrient compounds before Metqbolism pass through the mucous membrane of nutriet alimentary canal further enter into the qbsorption and Metabolism boosters. The absodption such as mechanical, chemical and microbial are essential for digestion into simpler form.
After nutrirnt, the nutrients are utilized for maintenance as well as Metabolosm of Metabolism and nutrient absorption different molecules Metanolism are important nutriet the biological system.
Nutrienf is a nutient of absorphion content, log in via an institution. Annison EF, Linzell Metabolisj Oxidation and utilization of glucose and acetate by the mammary gland abdorption the goat in relation to their xbsorption metabolism and to milk formation. Metabolism and nutrient absorption Physiol — Article CAS Google Scholar.
Zbsorption DG, EMtabolism RR The fate of carbohydrates in the small and large Metabolism and nutrient absorption of the ruminant. J Sci Food Agric — Google Scholar. Bergman EN Glucose Metabolism and nutrient absorption in ruminants as related to hypoglycemia and ketosis.
Cornell Vet — Bickerstaffe et al. CAS Google Scholar. Bickerstaffe R, Annison EF, Linzell JF The metabolism of glucose, acetate, lipids and amino acids in lactating dairy cows. J Agric Sci Camb — Article Google Scholar.
Bird AR, Croome WJ Jr, Fan YK, Black BL, McBride BW, Taylor IL Peptide regulation of intestinal glucose absorption. J Anim Sci — MorrisonChurch Hobson PN Rumen bacteria. In: Norris JR, Ribbons DW eds Methods in microbiology, vol vol 3B.
Academic press, London and New York, pp — Hoover WH Digestion and absorption in the hind gut of ruminants. Horsfield S, Infield JM, Annison EF Compartmental analysis and model building in the study of glucose kinetics in the lactating cow.
Proc Nutr Soc — Bergman and Hogue Hungate RE The rumen and its microbes. Academic Press, New York and London. Linzell JL Mammary-gland blood flow and oxygen, glucose and volatile fatty acid uptake in the conscious goat. McDonald P, Edward RA, Greenhalgh JFD Animal nutrition, 4th edn. Ranjhan SK Animal nutrition and feeding practices, 6th rev edn.
Vikas Publishing House, New Delhi. Theander O Chemical composition of low quality roughages as related to alkali treatment.
In: Kategile JA, Said AN, Sundstol F eds Utilization of low quality roughages in Africa. Agricultural development report 1. The Agricultural University of Norway, Aas, pp 1— Theurer CB Grain processing effects on starch utilization by ruminants. Waldo DR Extent and partition of cereal grain starch digestion in ruminants.
Wiltrout DW, Satter LD Contribution of propionate to glucose synthesis in the lactating and non-lactating cow. J Dairy Sci — Download references. Indian Veterinary Research Institute, Bareilly, Uttar Pradesh, India.
National Academy of Veterinary Nutrition and Animal Welfare, Bareilly, Uttar Pradesh, India. You can also search for this author in PubMed Google Scholar.
Reprints and permissions. Saha, S. Digestion, Absorption and Metabolism of Nutrients. In: Fundamentals of Animal Nutrition.
Springer, Singapore. Published : 12 May Publisher Name : Springer, Singapore. Print ISBN : Online ISBN : eBook Packages : Biomedical and Life Sciences Biomedical and Life Sciences R0. Anyone you share the following link with will be able to read this content:.
Sorry, a shareable link is not currently available for this article. Provided by the Springer Nature SharedIt content-sharing initiative. Policies and ethics. Skip to main content. Abstract The physiology of digestion as well as the digestive system in larger animals is essentially similar for all monogastric and simple stomach animal.
Buying options Chapter EUR eBook EUR Softcover Book EUR Hardcover Book EUR Tax calculation will be finalised at checkout Purchases are for personal use only Learn about institutional subscriptions.
References Annison EF, Linzell JL Oxidation and utilization of glucose and acetate by the mammary gland of the goat in relation to their overall metabolism and to milk formation.
J Physiol — Article CAS Google Scholar Armstrong DG, Smithard RR The fate of carbohydrates in the small and large intestines of the ruminant. J Sci Food Agric — Google Scholar Bergman EN Glucose metabolism in ruminants as related to hypoglycemia and ketosis.
J Agric Sci Camb —85 Article Google Scholar Bird AR, Croome WJ Jr, Fan YK, Black BL, McBride BW, Taylor IL Peptide regulation of intestinal glucose absorption.
MorrisonChurch Article CAS Google Scholar Hobson PN Rumen bacteria. Academic press, London and New York, pp — Google Scholar Hoover WH Digestion and absorption in the hind gut of ruminants.
J Anim Sci — Article CAS Google Scholar Horsfield S, Infield JM, Annison EF Compartmental analysis and model building in the study of glucose kinetics in the lactating cow.
Bergman and Hogue Article CAS Google Scholar Hungate RE The rumen and its microbes. Academic Press, New York and London Google Scholar Linzell JL Mammary-gland blood flow and oxygen, glucose and volatile fatty acid uptake in the conscious goat.
J Physiol — Article CAS Google Scholar McDonald P, Edward RA, Greenhalgh JFD Animal nutrition, 4th edn. Vikas Publishing House, New Delhi Google Scholar Theander O Chemical composition of low quality roughages as related to alkali treatment. The Agricultural University of Norway, Aas, pp 1—27 Google Scholar Theurer CB Grain processing effects on starch utilization by ruminants.
J Anim Sci — Article CAS Google Scholar Waldo DR Extent and partition of cereal grain starch digestion in ruminants. J Anim Sci — Article CAS Google Scholar Wiltrout DW, Satter LD Contribution of propionate to glucose synthesis in the lactating and non-lactating cow.
J Dairy Sci — Article CAS Google Scholar Download references. Author information Authors and Affiliations Indian Veterinary Research Institute, Bareilly, Uttar Pradesh, India Subodh Kumar Saha National Academy of Veterinary Nutrition and Animal Welfare, Bareilly, Uttar Pradesh, India Nitya Nand Pathak Authors Subodh Kumar Saha View author publications.
View author publications. Rights and permissions Reprints and permissions. Copyright information © The Author sunder exclusive license to Springer Nature Singapore Pte Ltd. About this chapter. Cite this chapter Saha, S.
Copy to clipboard. Publish with us Policies and ethics.
: Metabolism and nutrient absorptionEnergy & Metabolism Part 1: Nutrients | Role Metabilism the microbiota Metabolism and nutrient absorption immunity absor;tion inflammation. These Antiviral defense against diseases and common organic and inorganic nutrients are proteins, fats, carbohydrates, water, minerals and vitamins. Carbohydrate metabolism in Bifidobacteria. Human Microbiome Project Consortium. Pancreatic juices also contain amylase, which continues the breakdown of starch and glycogen into maltose, a disaccharide. |
Nutrient Transport and Energy Metabolism - Medicine LibreTexts | Song, Y. Vitamins can be either water-soluble or lipid-soluble. The lipids we consume include fats, oils, phospholipids, fatty acids, and sterols e. During the reactions catalyzed by these enzymes, FAD is reduced to FADH 2 , whose electrons are then transferred to O 2 through cytochrome reductase and cytochrome oxidase, as described for NADH dehydrogenase electrons Figure 2. Article CAS PubMed Google Scholar Chassard C, Delmas E, Robert C, Lawson PA. |
Human Metabolism, Energy, Nutrients | Learn Science at Scitable | Why is emulsification important for digestion of lipids? Pancreatic juices contain enzymes called lipases enzymes that break down lipids. If the lipid in the chyme aggregates into large globules, very little surface area of the lipids is available for the lipases to act on, leaving lipid digestion incomplete. By forming an emulsion, bile salts increase the available surface area of the lipids many folds. The pancreatic lipases can then act on the lipids more efficiently and digest them, as detailed in Figure 5. Lipases break down the lipids into fatty acids and glycerides. These molecules can pass through the plasma membrane of the cell and enter the epithelial cells of the intestinal lining. The bile salts surround long-chain fatty acids and monoglycerides forming tiny spheres called micelles. The micelles move into the brush border of the small intestine absorptive cells where the long-chain fatty acids and monoglycerides diffuse out of the micelles into the absorptive cells leaving the micelles behind in the chyme. The long-chain fatty acids and monoglycerides recombine in the absorptive cells to form triglycerides, which aggregate into globules and become coated with proteins. These large spheres are called chylomicrons. Chylomicrons contain triglycerides, cholesterol, and other lipids and have proteins on their surface. Together, they enable the chylomicron to move in an aqueous environment without exposing the lipids to water. Chylomicrons leave the absorptive cells via exocytosis. Chylomicrons enter the lymphatic vessels and then enter the blood in the subclavian vein. Vitamins can be either water-soluble or lipid-soluble. Fat-soluble vitamins are absorbed in the same manner as lipids. It is important to consume some amount of dietary lipid to aid the absorption of lipid-soluble vitamins. Water-soluble vitamins can be directly absorbed into the bloodstream from the intestine. Figure 5. Mechanical and chemical digestion of food takes place in many steps, beginning in the mouth and ending in the rectum. Which of the following statements about digestive processes is true? Amylase, maltase, and lactase in the mouth digest carbohydrates. Trypsin and lipase in the stomach digest protein. Bile emulsifies lipids in the small intestine. No food is absorbed until the small intestine. The final step in digestion is the elimination of undigested food content and waste products. The undigested food material enters the colon, where most of the water is reabsorbed. The semi-solid waste is moved through the colon by peristaltic movements of the muscle and is stored in the rectum. As the rectum expands in response to storage of fecal matter, it triggers the neural signals required to set up the urge to eliminate. The solid waste is eliminated through the anus using peristaltic movements of the rectum. Diarrhea and constipation are some of the most common health concerns that affect digestion. Constipation is a condition where the feces are hardened because of excess water removal in the colon. In contrast, if enough water is not removed from the feces, it results in diarrhea. Many bacteria, including the ones that cause cholera, affect the proteins involved in water reabsorption in the colon and result in excessive diarrhea. Emesis, or vomiting, is the elimination of food by forceful expulsion through the mouth. It is often in response to an irritant that affects the digestive tract, including but not limited to viruses, bacteria, emotions, sights, and food poisoning. This forceful expulsion of the food is due to the strong contractions produced by the stomach muscles. The process of emesis is regulated by the medulla. Think about the digestion lab you had earlier this week. Summarize digestion of lipids, carbohydrates, and proteins in the digestive system based on your lab observations and results with your lab partners. Book: Introductory Animal Physiology Hinić-Frlog. Search site Search Search. Go back to previous article. Sign in. Learning Objectives Summarize chemical digestion of carbohydrates, proteins and fats and energy metabolism. Digestive system processes Obtaining nutrition and energy from food is a multi-step process. Ingestion The large molecules found in intact food cannot pass through the cell membranes. Digestion and absorption Digestion is the mechanical and chemical break down of food into small organic fragments. Carbohydrates The digestion of carbohydrates begins in the mouth. Digestion of carbohydrates is performed by several enzymes. Starch and glycogen are broken down into glucose by amylase and maltase. Sucrose table sugar and lactose milk sugar are broken down by sucrase and lactase, respectively. Table 5. Digestion of carbohydrates Enzyme Produced By Site of Action Substrate Acting On End Products Salivary amylase Salivary glands Mouth Polysaccharides Starch Disaccharides maltose , oligosaccharides Pancreatic amylase Pancreas Small intestine Polysaccharides starch Disaccharides maltose , monosaccharides Oligosaccharidases Lining of the intestine; brush border membrane Small intestine Disaccharides Monosaccharides e. Protein A large part of protein digestion takes place in the stomach. Protein digestion is a multistep process that begins in the stomach and continues through the intestines. Digestion of proteins. Enzyme Produced By Site of Action Substrate Acting On End Products Pepsin Stomach chief cells Stomach Proteins Peptides Trypsin Elastase Chymotrypsin Pancreas Small intestine Proteins Peptides Carboxypeptidase Pancreas Small intestine Peptides Amino acids and peptides Aminopeptidase Dipeptidase Lining of intestine Small intestine Peptides Amino acids. Lipids Lipid digestion begins in the stomach with the aid of lingual lipase and gastric lipase. Lipids are digested and absorbed in the small intestine. Vitamins Vitamins can be either water-soluble or lipid-soluble. NOTES Review Figure 5. NOTES Not all animals process different nutrients equally. Here is is a video about hummingbird digestion with a focus on work by Dr. Welch at the University of Toronto Scarborough. Disaccharides maltose , oligosaccharides. Disaccharides maltose , monosaccharides. Lining of the intestine; brush border membrane. Moreover, infusion of leucine alone into the duodenum dose-dependently increases insulin, with slight decreases in glucose, but no change in glucagon These effects are mediated by peptide transporter-1 PepT1 , a di- and tri-peptide proton-coupled transporter located in the brush border membrane of the intestinal epithelium Fig. Recent evidence using isolated intestinal perfusion technique indicates that dietary protein induces gut peptide secretion via transport of oligopeptides into cells via PepT1. Cellular oligopeptides are broken down into individual amino acids that are released to the basolateral side of the intestine to activate amino acid receptors Taken together, these data indicate that both apical PepT1 and basolateral CaSR could be critical for peptone-mediated GLP-1 release Fig. Nonetheless, more work is needed to determine the exact mechanism linking intestinal protein sensing to gut peptide release, and which specific amino acids and sensors are required. In contrast to lipids and carbohydrates, sensitivity to intestinal protein-sensing appears to be maintained during obesity, highlighting the potential of protein-sensing as a therapeutic target for weight loss. There are no differences in energy intake or CCK and GLP-1 responses between individuals with and without obesity following intraduodenal whey protein infusion In line with this data, rats fed an HFD for either 3 or 28 days, with the latter resulting in increased adiposity, still responded to small intestinal casein infusion by lowering hepatic glucose production In addition, high protein intake improves metabolic outcomes, like body weight, adiposity, insulin sensitivity, and food intake, in both rodents and humans , , , and improves glucose tolerance and lowers blood glucose levels in patients with diabetes This may be explained by the fact that intestinal proteins more potently stimulate gut peptide secretion as compared to isocaloric lipids or carbohydrates Future research is warranted to uncover the mechanisms of how intestinal protein sensing, but not lipid or carbohydrate sensing, is maintained during metabolic dysregulation. Changes in the gut microbiota affect obesity and related metabolic disorders, and the mechanisms linking the gut microbiota to energy and glucose homeostasis have been extensively reviewed , However, the majority of the studies have focused on the role of the microbiota in the large intestine, and few studies have examined the metabolic impact of the small intestinal microbiota. While there are several orders of magnitude greater abundance of bacteria in the large intestine than in the small intestine, nutrient-sensing, and gut—brain feedback mechanisms are localized to the small intestine, as nutrient absorption limits ingested macronutrients from reaching the large intestine. Further, the protective barrier of a mucus layer in the small intestine is much less established , allowing for an increased potential for intimate interactions between the host epithelial cells and the gut bacteria. For example, restoring the gut microbiome in germ-free mice results in an acute, transient phase, followed by a homeostatic phase that impacts jejunal transcriptomics and metabolomics involved in lipid and glucose metabolism and uptake However, the initial acute response is not observed in the ileum or colon, highlighting the sensitivity of the upper small intestine to the microbiome. Evidence suggests that the microbiota could also greatly impact nutrient-sensing mechanisms. First, microbial metabolites, especially short-chain fatty acids SCFAs , are known to induce gut peptide secretion from EECs , Most bacterially derived metabolites like SCFAs are produced predominantly in the distal intestine but are also present in small amounts in the ileum and can reduce glucose production via a gut—brain axis , Other metabolites, like indole, are highly abundant in the small intestine and also regulate GLP-1 release from EECs Secondly, the gut microbiota impacts EEC physiology. For example, isolated cells expressing GLP-1 obtained from germ-free and conventional mice exhibit different transcriptomes, which is rapidly altered after only one day of microbiome colonization, suggesting a more direct effect of the bacteria on the EECs vs. an indirect effect from altered physiology of the germ-free model Further, intestinal expression and circulating levels of gut peptides are altered in germ-free mice , Similarly, HFD converts zebrafish EECs into a nutrient-insensitive state dependent on gut microbiota, as germ-free zebrafish are resistant to the induction of EEC nutrient-insensitivity while an Acinetobacter strain was able to induce EEC nutrient-insensitivity In line with this, bacterial species directly influence GPR, a receptor linked with lipid-induced gut peptide secretion, and GLP-1 expression in vitro Third, LPS, a bacterial byproduct, blunts vagal activation by intestinal nutrients, leptin, or CCK , Thus, there exists a precedent for the ability of small intestinal microbiota to impact nutrient-induced small intestinal gut—brain signaling Fig. We put forward a working hypothesis for the mechanistic links between small intestinal nutrient-sensing, microbiota, peptide release, and metabolic regulation. Bacterial by-products such as LPS can impair lipid and glucose sensing and potentially disrupt ACSL3 and SGLT1 dependent pathways that regulate glucose and energy homeostasis. Bile salt hydrolase of bacteria contributes to the bile acid pool and regulates bile acid metabolism. As a result, changes in bile acids can alter GLP-1 release and metabolic regulation via intestinal FXR and TGR5 signaling. High-fat feeding reduces the abundance of small intestinal Lactobacillus species e. gasseri and consequently inhibits ACSL3 expression and impairs lipid sensing. Lastly, metformin increases the abundance of upper small intestinal Lactobacillus and enhances SGLT1 expression and glucose sensing, while also reducing the abundance of Bacteroides fragilis that results in ileal FXR inhibition and improvement in glucose metabolism. Bariatric surgery enhances small intestinal nutrient sensing mechanisms and consequently lowers glucose levels, while changes in bile acid metabolism and FXR are necessary for the glucose-lowering effect of bariatric surgery. In parallel, gut microbiota alters the bile acid pool and thereby potentially affects nutrient sensing and glucose and energy homeostasis. Conjugated bile acids are produced in the liver and released into the duodenum, where they are either absorbed or de-conjugated by the bile salt hydrolase of bacteria. Bile acids act as signaling molecules in the intestine and elsewhere, binding to FXR and G protein-coupled receptor 19 also known as TGR5 Most, but not all, studies indicate that inhibition of intestinal FXR improves energy and glucose homeostasis , and FXR signaling represses transcription of GLP-1 and inhibits GLP-1 release from L-cells Interestingly, TGR5 signaling increases GLP-1 release from L-cells , thus complicating the role of bile acid signaling in the intestine Fig. HF-feeding, obesity, and diabetes are all associated with unique microbial profiles in the large intestine. However, evidence suggests that HF-feeding also alters the composition of small intestinal gut microbiota. In rodents, the majority of the small intestinal bacteria are Lactobacillius , and HF-feeding results in a drastic reduction in the relative abundance of this genus 45 , Recent work indicates that altered small intestinal microbiota during HFD drives impairments in intestinal lipid-sensing, as the transplant of the small intestinal microbiota of short-term HF fed rats into chow-fed rats abolished the ability of small intestinal lipid infusion to improve glucose tolerance and lower hepatic glucose production. Treatment of HF-fed rats with a small intestinal infusion of Lactobacillus gasseri enhances upper small intestinal lipid-sensing, via restoration of long-chain acyl-CoA synthetase ACSL3 gasseri exhibits bile salt hydrolase activity and can thus alter the composition of the bile acid pool. Small intestinal L. gasseri increases ACSL3 and subsequent lipid-sensing through a mechanism dependent on reduced FXR signaling. These findings are consistent with the fact that bile acid sequestrants i. Recent evidence-based on studies with the anti-diabetic medicine metformin indicate that the glucoregulatory impact of intestinal glucose-sensing is mediated by the small intestinal microbiota. While metformin directly influences hepatic metabolism , as an orally administered drug metformin concentrations in the small intestine are much greater than in the serum Oral metformin reduces blood glucose levels more than intravenous or portal vein administration , demonstrating a role for intestinal-mediated mechanisms of action in improvements in glucose homeostasis. Pretreatment of HF-fed rats with metformin restores the ability of upper small intestinal glucose infusion to lower glucose production via increased portal vein GLP-1 levels and small intestinal SGLT-1 expression and in parallel changes the composition of small intestinal microbiota This is in line with several other studies that highlight the importance of the gut microbiota in mediating the beneficial effects of metformin , In addition, individuals with newly diagnosed diabetes treated with metformin for three days exhibit alterations in the gut microbiota including increased Lactobacillus and reduced Bacteroides fragilis abundance, which result in inhibition of FXR signaling to improve glucose metabolism This observation is similar to the ability of L. gasseri to increase intestinal lipid-sensing to improve glucose homeostasis via FXR 45 Fig. Collectively, these studies highlight small intestinal nutrient-sensing mechanism mediates the beneficial effects of metformin through changes in gut microbiota and bile acids. Evidence is emerging on the impact of the small intestinal microbiota also in the efficacy of gastric bypass. Despite extensive evidence of an overall role of the large intestinal microbiota in mediating the effects of bariatric surgery , at least one study demonstrated that gastric bypass alters the microbiota of the duodenum, jejunum, and ileum In addition, while the jejunal nutrient-sensing mechanism at least partly mediates the beneficial effects of duodenal—jejunal bypass surgery on glucose homeostasis 98 , the glucose-lowering effect of vertical sleeve gastrectomy is dependent on both the gut microbiota and bile acid signaling Fig. While technological advancements begin to detail the role of intestinal nutrient-sensing in gut—brain neuronal signaling, they concurrently expand the field. One example of this is the use of single-cell RNA sequencing to understand vagal afferent signaling. Several groups distinctly labeled nodose ganglion neurons according to their expression profile, however, the results are expansive and sometimes contradictory 44 , Based on these studies, vagal afferent neurons containing GLP-1R have no impact on intestinal nutrient-sensing mechanisms, which are instead regulated by GPRpositive neurons Indeed, various neurons terminating in the intestinal mucosa, that likely sense gut peptides released in response to intestinal nutrients, have no effect on food intake, and only direct activation of a subset of IGLE neurons that detect intestinal stretch and not gut peptides suppresses food intake A subset of EECs called neuropods exist that directly synapse with vagal neurons, and rapidly signal via glutamate to the nucleus of the solitary tract in a single synapse to relay initial spatial and temporal information about the meal that could later be followed by more traditional gut peptide signaling Despite these interesting and exciting advances and the discovery of new nutrient sensory cells, the exact neurons that mediate the gut—brain signaling and nutrient sensing in regulating metabolism are complex and warrant future investigations. Future studies are needed to start teasing apart these complexities, while also integrating the gut microbiota and metabolites into the picture. For instance, while the gut microbiota can impact EECs, it is plausible that vagal afferents themselves can be impacted by bacterial metabolites In contrast to energy intake, the impact of nutrient-induced gut—brain vagal signaling on energy expenditure has been poorly characterized. Intestinal lipids regulate brown fat thermogenesis via vagal afferents and possibly via GLP-1R signaling , and vagal knockout of the transcription factor peroxisome proliferator-activated receptor-γ, which is activated by fatty acids and could thus be involved in lipid-sensing, affects thermogenesis Likewise in humans, intraduodenal infusion of intralipid increases resting energy expenditure Nutrient infusions into the duodenum of rats modulate energy expenditure Future work is needed to detail the connections between nutrient-sensing mechanism, gut microbiota, and impact on energy expenditure via thermogenesis in brown or browning white adipose tissue Overall, extensive evidence indicates that targeting nutrient sensing in the small intestine impacts energy and glucose homeostasis during normal physiology and in the context of obesity and type 2 diabetes. Given the distinct effects of HFD and obesity on the diminution of nutrient-sensing dependent gut—brain pathways, future studies examining the gene and environmental interactions are warranted to further the development of personalized medicine approaches. Similarly, the expansive role of the gut microbiota in host metabolic health further highlights the need for personalized approaches to treating metabolic diseases. As such, studies in humans and rodents beginning to unravel the interactions between the gut microbiota, small intestinal EECs, and vagal signaling, are laying the groundwork for the development of therapeutics targeting small intestinal nutrient sensing to treat obesity and type 2 diabetes. Obesity and Overweight. World Health Organization, Bhupathiraju, S. Epidemiology of obesity and diabetes and their cardiovascular complications. Article CAS PubMed PubMed Central Google Scholar. Arterburn, D. et al. Comparative effectiveness of bariatric surgery vs. nonsurgical treatment of type 2 diabetes among severely obese adults. Article PubMed PubMed Central Google Scholar. Brolin, R. Bariatric surgery and long-term control of morbid obesity. Article Google Scholar. Neunlist, M. Nutrient-induced changes in the phenotype and function of the enteric nervous system. Bentsen, M. Revisiting how the brain senses glucose-and why. Cell Metab. Article CAS PubMed Google Scholar. Rutter, G. Pancreatic beta-cell identity, glucose sensing and the control of insulin secretion. Oosterveer, M. Hepatic glucose sensing and integrative pathways in the liver. Life Sci. Haber, A. A single-cell survey of the small intestinal epithelium. Nature , — Article ADS CAS PubMed PubMed Central Google Scholar. Grun, D. Single-cell messenger RNA sequencing reveals rare intestinal cell types. Article ADS PubMed CAS Google Scholar. Glass, L. Single-cell RNA-sequencing reveals a distinct population of proglucagon-expressing cells specific to the mouse upper small intestine. Rocca, A. Role of the vagus nerve in mediating proximal nutrient-induced glucagon-like peptide-1 secretion. Endocrinology , — Christiansen, C. The impact of short-chain fatty acids on GLP-1 and PYY secretion from the isolated perfused rat colon. Liver Physiol. Article CAS Google Scholar. Nauck, M. Incretin effects of increasing glucose loads in man calculated from venous insulin and C-peptide responses. Holst, J. The incretin system in healthy humans: the role of GIP and GLP Metabolism 96 , 46—55 Song, Y. Gut-proglucagon-derived peptides are essential for regulating glucose homeostasis in mice. Cote, C. Hormonal signaling in the gut. Grasset, E. A specific gut microbiota dysbiosis of type 2 diabetic mice induces GLP-1 resistance through an enteric NO-dependent and gut-brain axis mechanism. Ritter, R. A tale of two endings: modulation of satiation by NMDA receptors on or near central and peripheral vagal afferent terminals. Waise, T. The metabolic role of vagal afferent innervation. Article PubMed Google Scholar. Muller, T. Glucagon-like peptide 1 GLP Krieger, J. Knockdown of GLP-1 receptors in vagal afferents affects normal food intake and glycemia. Diabetes 65 , 34—43 CAS PubMed Google Scholar. Varin, E. Distinct neural sites of GLP-1R expression mediate physiological versus pharmacological control of incretin action. Cell Rep. Diepenbroek, C. Validation and characterization of a novel method for selective vagal deafferentation of the gut. Cheung, G. Intestinal cholecystokinin controls glucose production through a neuronal network. Soty, M. Gut-brain glucose signaling in energy homeostasis. Greenberg, D. Intraduodenal infusions of fats elicit satiety in sham-feeding rats. Welch, I. Effect of ileal and intravenous infusions of fat emulsions on feeding and satiety in human volunteers. Gastroenterology 89 , — Time course for entry of intestinally infused lipids into blood of rats. French, S. The effects of intestinal infusion of long-chain fatty acids on food intake in humans. Gastroenterology , — Hajishafiee, M. Gastrointestinal sensing of meal-related signals in humans, and dysregulations in eating-related disorders. Nutrients 11 , Article CAS PubMed Central Google Scholar. Lu, W. Chylomicron formation and secretion is required for lipid-stimulated release of incretins GLP-1 and GIP. Lipids 47 , — Randich, A. Responses of celiac and cervical vagal afferents to infusions of lipids in the jejunum or ileum of the rat. Sakata, Y. Postabsorptive factors are important for satiation in rats after a lipid meal. Matzinger, D. The role of long chain fatty acids in regulating food intake and cholecystokinin release in humans. Gut 46 , — Lu, V. Free fatty acid receptors in enteroendocrine cells. Christensen, L. Vascular, but not luminal, activation of FFAR1 GPR40 stimulates GLP-1 secretion from isolated perfused rat small intestine. Article PubMed PubMed Central CAS Google Scholar. Psichas, A. Chylomicrons stimulate incretin secretion in mouse and human cells. Diabetologia 60 , — Tran, T. Luminal lipid regulates CD36 levels and downstream signaling to stimulate chylomicron synthesis. Schwartz, G. The lipid messenger OEA links dietary fat intake to satiety. Sundaresan, S. CDdependent signaling mediates fatty acid-induced gut release of secretin and cholecystokinin. FASEB J. Nakagawa, A. Receptor gene expression of glucagon-like peptide-1, but not glucose-dependent insulinotropic polypeptide, in rat nodose ganglion cells. Dailey, M. Jejunal linoleic acid infusions require GLP-1 receptor signaling to inhibit food intake: implications for the effectiveness of Roux-en-Y gastric bypass. Williams, E. Sensory neurons that detect stretch and nutrients in the digestive system. Cell , — Bauer, P. Lactobacillus gasseri in the upper small intestine impacts an ACSL3-dependent fatty acid-sensing pathway regulating whole-body glucose homeostasis. Wang, P. Upper intestinal lipids trigger a gut-brain-liver axis to regulate glucose production. Article ADS CAS PubMed Google Scholar. Xiao, C. Evaluation of the effect of enteral lipid sensing on endogenous glucose production in humans. Diabetes 64 , — Breen, D. Duodenal PKC-delta and cholecystokinin signaling axis regulates glucose production. Diabetes 60 , — Takahashi, A. Involvement of calmodulin and protein kinase C in cholecystokinin release by bombesin from STC-1 cells. Pancreas 21 , — Raybould, H. Inhibition of gastric emptying in response to intestinal lipid is dependent on chylomicron formation. Meloni, A. GLP-1 receptor activated insulin secretion from pancreatic beta-cells: mechanism and glucose dependence. Diabetes Obes. Heruc, G. Effects of dipeptidyl peptidase IV inhibition on glycemic, gut hormone, triglyceride, energy expenditure, and energy intake responses to fat in healthy males. Wu, T. Comparative effects of intraduodenal fat and glucose on the gut-incretin axis in healthy males. Peptides 95 , — Verspohl, E. Cholecystokinin CCK8 regulates glucagon, insulin, and somatostatin secretion from isolated rat pancreatic islets: interaction with glucose. Duca, F. The modulatory role of high fat feeding on gastrointestinal signals in obesity. Reduced CCK signaling in obese-prone rats fed a high fat diet. Decreased intestinal nutrient response in diet-induced obese rats: role of gut peptides and nutrient receptors. Impaired GLP-1 signaling contributes to reduced sensitivity to duodenal nutrients in obesity-prone rats during high-fat feeding. Obesity 23 , — Boyd, K. High-fat diet effects on gut motility, hormone, and appetite responses to duodenal lipid in healthy men. Brennan, I. Effects of fat, protein, and carbohydrate and protein load on appetite, plasma cholecystokinin, peptide YY, and ghrelin, and energy intake in lean and obese men. Speechly, D. Appetite dysfunction in obese males: evidence for role of hyperinsulinaemia in passive overconsumption with a high fat diet. Current and emerging concepts on the role of peripheral signals in the control of food intake and development of obesity. Stewart, J. Marked differences in gustatory and gastrointestinal sensitivity to oleic acid between lean and obese men. Lee, S. Blunted vagal cocaine- and amphetamine-regulated transcript promotes hyperphagia and weight gain. Burdyga, G. Expression of the leptin receptor in rat and human nodose ganglion neurones. Neuroscience , — Peters, J. Modulation of vagal afferent excitation and reduction of food intake by leptin and cholecystokinin. Barrachina, M. Synergistic interaction between leptin and cholecystokinin to reduce short-term food intake in lean mice. Natl Acad. USA 94 , — de Lartigue, G. resistance in vagal afferent neurons inhibits cholecystokinin signaling and satiation in diet induced obese rats. PLoS ONE 7 , e Article ADS PubMed PubMed Central CAS Google Scholar. Batt, R. Deletion of leptin signaling in vagal afferent neurons results in hyperphagia and obesity. Rasmussen, B. Duodenal activation of cAMP-dependent protein kinase induces vagal afferent firing and lowers glucose production in rats. Gastrointestinal mechanisms of satiation for food. Lavin, J. Appetite regulation by carbohydrate: role of blood glucose and gastrointestinal hormones. Interaction of insulin, glucagon-like peptide 1, gastric inhibitory polypeptide, and appetite in response to intraduodenal carbohydrate. Schultes, B. Glycemic increase induced by intravenous glucose infusion fails to affect hunger, appetite, or satiety following breakfast in healthy men. Appetite , — Williams, D. Evidence that intestinal glucagon-like peptide-1 plays a physiological role in satiety. Gorboulev, V. Diabetes 61 , — Parker, H. Predominant role of active versus facilitative glucose transport for glucagon-like peptide-1 secretion. Diabetologia 55 , — Reimann, F. Glucose sensing in L cells: a primary cell study. Sun, E. Mechanisms controlling glucose-induced GLP-1 secretion in human small intestine. Diabetes 66 , — Kuhre, R. On the relationship between glucose absorption and glucose-stimulated secretion of GLP-1, neurotensin, and PYY from different intestinal segments in the rat. Article PubMed Central CAS Google Scholar. Jang, H. Gut-expressed gustducin and taste receptors regulate secretion of glucagon-like peptide USA , — Saltiel, M. Sweet taste receptor activation in the gut is of limited importance for glucose-stimulated GLP-1 and GIP secretion. Nutrients 9 , Han, P. The sweet taste signalling pathways in the oral cavity and the gastrointestinal tract affect human appetite and food intake: a review. Food Sci. Chaikomin, R. Effects of mid-jejunal compared to duodenal glucose infusion on peptide hormone release and appetite in healthy men. Poppitt, S. Duodenal and ileal glucose infusions differentially alter gastrointestinal peptides, appetite response, and food intake: a tube feeding study. Woltman, T. Effects of duodenal and distal ileal infusions of glucose and oleic acid on meal patterns in rats. Spiller, R. The ileal brake—inhibition of jejunal motility after ileal fat perfusion in man. Gut 25 , — Maljaars, P. Ileal brake: a sensible food target for appetite control. A review. Powell, D. Zhang, X. Comparative effects of proximal and distal small intestinal glucose exposure on glycemia, incretin hormone secretion, and the incretin effect in health and type 2 diabetes. Diabetes Care 42 , — Hansotia, T. GIP and GLP-1 as incretin hormones: lessons from single and double incretin receptor knockout mice. Gasbjerg, L. Separate and combined glucometabolic effects of endogenous glucose-dependent insulinotropic polypeptide and glucagon-like peptide 1 in healthy individuals. Diabetes 68 , — Ionut, V. Hayes, M. The common hepatic branch of the vagus is not required to mediate the glycemic and food intake suppressive effects of glucagon-like-peptide Lamont, B. Pancreatic GLP-1 receptor activation is sufficient for incretin control of glucose metabolism in mice. Metformin alters upper small intestinal microbiota that impact a glucose-SGLT1-sensing glucoregulatory pathway. Jejunal nutrient sensing is required for duodenal-jejunal bypass surgery to rapidly lower glucose concentrations in uncontrolled diabetes. Yang, M. Duodenal GLP-1 signaling regulates hepatic glucose production through a PKC-delta-dependent neurocircuitry. Cell Death Dis. Chapman, I. Effects of small-intestinal fat and carbohydrate infusions on appetite and food intake in obese and nonobese men. Naslund, E. Glucagon-like peptide-1 in the pathogenesis of obesity. Drug N. Combination of obesity and high-fat feeding diminishes sensitivity to GLP-1R agonist exendin Diabetes 62 , — Maintenance on a high-fat diet impairs the anorexic response to glucagon-like-peptide-1 receptor activation. Perez, C. Devazepide, a CCK A antagonist, attenuates the satiating but not the preference conditioning effects of intestinal carbohydrate infusions in rats. Evaluation of the incretin effect in humans using GIP and GLP-1 receptor antagonists. Peptides , Richards, P. High fat diet impairs the function of glucagon-like peptide-1 producing L-cells. Peptides 77 , 21—27 Ryan, A. Intraduodenal protein modulates antropyloroduodenal motility, hormone release, glycemia, appetite, and energy intake in lean men. Effects of intraduodenal lipid and protein on gut motility and hormone release, glycemia, appetite, and energy intake in lean men. Ullrich, S. Comparative effects of intraduodenal protein and lipid on ghrelin, peptide YY, and leptin release in healthy men. American journal of physiology. Regulatory, Integr. van Avesaat, M. Small intestinal protein infusion in humans: evidence for a location-specific gradient in intestinal feedback on food intake and GI peptide release. Int J. Bensaid, A. Protein is more potent than carbohydrate for reducing appetite in rats. Reidelberger, R. Effects of peripheral CCK receptor blockade on feeding responses to duodenal nutrient infusions in rats. Darcel, N. Activation of vagal afferents in the rat duodenum by protein digests requires PepT1. Yox, D. Vagotomy attenuates suppression of sham feeding induced by intestinal nutrients. LaPierre, M. Glucagon signalling in the dorsal vagal complex is sufficient and necessary for high-protein feeding to regulate glucose homeostasis in vivo. EMBO Rep. Claessens, M. Blouet, C. The reduced energy intake of rats fed a high-protein low-carbohydrate diet explains the lower fat deposition, but macronutrient substitution accounts for the improved glycemic control. Gannon, M. An increase in dietary protein improves the blood glucose response in persons with type 2 diabetes. Steinert, R. Effects of intraduodenal infusion of the branched-chain amino acid leucine on ad libitum eating, gut motor and hormone functions, and glycemia in healthy men. Dranse, H. Physiological and therapeutic regulation of glucose homeostasis by upper small intestinal PepT1-mediated protein sensing. Diakogiannaki, E. Oligopeptides stimulate glucagon-like peptide-1 secretion in mice through proton-coupled uptake and the calcium-sensing receptor. Diabetologia 56 , — Caron, J. Protein digestion-derived peptides and the peripheral regulation of food intake. Modvig, I. Peptone-mediated glucagon-like peptide-1 secretion depends on intestinal absorption and activation of basolaterally located Calcium-Sensing Receptors. Mace, O. The regulation of K- and L-cell activity by GLUT2 and the calcium-sensing receptor CasR in rat small intestine. Wang, J. Umami receptor activation increases duodenal bicarbonate secretion via glucagon-like peptide-2 release in rats. Oya, M. The G protein-coupled receptor family C group 6 subtype A GPRC6A receptor is involved in amino acid-induced glucagon-like peptide-1 secretion from GLUTag cells. Hutchison, A. Comparative effects of intraduodenal whey protein hydrolysate on antropyloroduodenal motility, gut hormones, glycemia, appetite, and energy intake in lean and obese men. Arciero, P. Moderate protein intake improves total and regional body composition and insulin sensitivity in overweight adults. Metabolism 57 , — Pichon, L. A high-protein, high-fat, carbohydrate-free diet reduces energy intake, hepatic lipogenesis, and adiposity in rats. Lacroix, M. A long-term high-protein diet markedly reduces adipose tissue without major side effects in Wistar male rats. Manders, R. Co-ingestion of a protein hydrolysate and amino acid mixture with carbohydrate improves plasma glucose disposal in patients with type 2 diabetes. Raben, A. Meals with similar energy densities but rich in protein, fat, carbohydrate, or alcohol have different effects on energy expenditure and substrate metabolism but not on appetite and energy intake. Cani, P. Microbiota and metabolites in metabolic diseases. Sonnenburg, J. Diet-microbiota interactions as moderators of human metabolism. Nature , 56—64 Ermund, A. Studies of mucus in mouse stomach, small intestine, and colon. El Aidy, S. The gut microbiota elicits a profound metabolic reorientation in the mouse jejunal mucosa during conventionalisation. Gut 62 , — Covasa, M. Intestinal sensing by gut microbiota: targeting gut peptides. Tolhurst, G. Short-chain fatty acids stimulate glucagon-like peptide-1 secretion via the G-protein-coupled receptor FFAR2. Zoetendal, E. The human small intestinal microbiota is driven by rapid uptake and conversion of simple carbohydrates. ISME J. Zadeh-Tahmasebi, M. Activation of short and long chain fatty acid sensing machinery in the ileum lowers glucose production in vivo. Chimerel, C. Bacterial metabolite indole modulates incretin secretion from intestinal enteroendocrine L cells. Arora, T. Microbial regulation of the L cell transcriptome. Increased oral detection, but decreased intestinal signaling for fats in mice lacking gut microbiota. Samuel, B. Effects of the gut microbiota on host adiposity are modulated by the short-chain fatty-acid binding G protein-coupled receptor, Gpr Ye, L. High fat diet induces microbiota-dependent silencing of enteroendocrine cells. Elife 8 , e Fredborg, M. G protein-coupled receptor GPR transcription in intestinal epithelial cells is significantly affected by bacteria belonging to the Bacteroides, Proteobacteria, and Firmicutes phyla. de La Serre, C. Chronic exposure to low dose bacterial lipopolysaccharide inhibits leptin signaling in vagal afferent neurons. Diet-induced obesity leads to the development of leptin resistance in vagal afferent neurons. Wahlstrom, A. Intestinal crosstalk between bile acids and microbiota and its impact on host metabolism. Article PubMed CAS Google Scholar. Jiang, C. Intestine-selective farnesoid X receptor inhibition improves obesity-related metabolic dysfunction. Trabelsi, M. Farnesoid X receptor inhibits glucagon-like peptide-1 production by enteroendocrine L cells. Article ADS PubMed Google Scholar. |
Metabolism and nutrient absorption -
Cell , — Bauer, P. Lactobacillus gasseri in the upper small intestine impacts an ACSL3-dependent fatty acid-sensing pathway regulating whole-body glucose homeostasis. Wang, P. Upper intestinal lipids trigger a gut-brain-liver axis to regulate glucose production.
Article ADS CAS PubMed Google Scholar. Xiao, C. Evaluation of the effect of enteral lipid sensing on endogenous glucose production in humans.
Diabetes 64 , — Breen, D. Duodenal PKC-delta and cholecystokinin signaling axis regulates glucose production. Diabetes 60 , — Takahashi, A. Involvement of calmodulin and protein kinase C in cholecystokinin release by bombesin from STC-1 cells.
Pancreas 21 , — Raybould, H. Inhibition of gastric emptying in response to intestinal lipid is dependent on chylomicron formation. Meloni, A. GLP-1 receptor activated insulin secretion from pancreatic beta-cells: mechanism and glucose dependence.
Diabetes Obes. Heruc, G. Effects of dipeptidyl peptidase IV inhibition on glycemic, gut hormone, triglyceride, energy expenditure, and energy intake responses to fat in healthy males.
Wu, T. Comparative effects of intraduodenal fat and glucose on the gut-incretin axis in healthy males. Peptides 95 , — Verspohl, E. Cholecystokinin CCK8 regulates glucagon, insulin, and somatostatin secretion from isolated rat pancreatic islets: interaction with glucose.
Duca, F. The modulatory role of high fat feeding on gastrointestinal signals in obesity. Reduced CCK signaling in obese-prone rats fed a high fat diet. Decreased intestinal nutrient response in diet-induced obese rats: role of gut peptides and nutrient receptors.
Impaired GLP-1 signaling contributes to reduced sensitivity to duodenal nutrients in obesity-prone rats during high-fat feeding. Obesity 23 , — Boyd, K. High-fat diet effects on gut motility, hormone, and appetite responses to duodenal lipid in healthy men. Brennan, I. Effects of fat, protein, and carbohydrate and protein load on appetite, plasma cholecystokinin, peptide YY, and ghrelin, and energy intake in lean and obese men.
Speechly, D. Appetite dysfunction in obese males: evidence for role of hyperinsulinaemia in passive overconsumption with a high fat diet. Current and emerging concepts on the role of peripheral signals in the control of food intake and development of obesity.
Stewart, J. Marked differences in gustatory and gastrointestinal sensitivity to oleic acid between lean and obese men. Lee, S. Blunted vagal cocaine- and amphetamine-regulated transcript promotes hyperphagia and weight gain. Burdyga, G. Expression of the leptin receptor in rat and human nodose ganglion neurones.
Neuroscience , — Peters, J. Modulation of vagal afferent excitation and reduction of food intake by leptin and cholecystokinin. Barrachina, M. Synergistic interaction between leptin and cholecystokinin to reduce short-term food intake in lean mice. Natl Acad. USA 94 , — de Lartigue, G.
resistance in vagal afferent neurons inhibits cholecystokinin signaling and satiation in diet induced obese rats. PLoS ONE 7 , e Article ADS PubMed PubMed Central CAS Google Scholar.
Batt, R. Deletion of leptin signaling in vagal afferent neurons results in hyperphagia and obesity. Rasmussen, B.
Duodenal activation of cAMP-dependent protein kinase induces vagal afferent firing and lowers glucose production in rats. Gastrointestinal mechanisms of satiation for food. Lavin, J. Appetite regulation by carbohydrate: role of blood glucose and gastrointestinal hormones.
Interaction of insulin, glucagon-like peptide 1, gastric inhibitory polypeptide, and appetite in response to intraduodenal carbohydrate.
Schultes, B. Glycemic increase induced by intravenous glucose infusion fails to affect hunger, appetite, or satiety following breakfast in healthy men. Appetite , — Williams, D. Evidence that intestinal glucagon-like peptide-1 plays a physiological role in satiety.
Gorboulev, V. Diabetes 61 , — Parker, H. Predominant role of active versus facilitative glucose transport for glucagon-like peptide-1 secretion. Diabetologia 55 , — Reimann, F. Glucose sensing in L cells: a primary cell study. Sun, E. Mechanisms controlling glucose-induced GLP-1 secretion in human small intestine.
Diabetes 66 , — Kuhre, R. On the relationship between glucose absorption and glucose-stimulated secretion of GLP-1, neurotensin, and PYY from different intestinal segments in the rat. Article PubMed Central CAS Google Scholar. Jang, H. Gut-expressed gustducin and taste receptors regulate secretion of glucagon-like peptide USA , — Saltiel, M.
Sweet taste receptor activation in the gut is of limited importance for glucose-stimulated GLP-1 and GIP secretion. Nutrients 9 , Han, P. The sweet taste signalling pathways in the oral cavity and the gastrointestinal tract affect human appetite and food intake: a review.
Food Sci. Chaikomin, R. Effects of mid-jejunal compared to duodenal glucose infusion on peptide hormone release and appetite in healthy men. Poppitt, S. Duodenal and ileal glucose infusions differentially alter gastrointestinal peptides, appetite response, and food intake: a tube feeding study.
Woltman, T. Effects of duodenal and distal ileal infusions of glucose and oleic acid on meal patterns in rats. Spiller, R. The ileal brake—inhibition of jejunal motility after ileal fat perfusion in man.
Gut 25 , — Maljaars, P. Ileal brake: a sensible food target for appetite control. A review. Powell, D. Zhang, X. Comparative effects of proximal and distal small intestinal glucose exposure on glycemia, incretin hormone secretion, and the incretin effect in health and type 2 diabetes.
Diabetes Care 42 , — Hansotia, T. GIP and GLP-1 as incretin hormones: lessons from single and double incretin receptor knockout mice.
Gasbjerg, L. Separate and combined glucometabolic effects of endogenous glucose-dependent insulinotropic polypeptide and glucagon-like peptide 1 in healthy individuals. Diabetes 68 , — Ionut, V. Hayes, M. The common hepatic branch of the vagus is not required to mediate the glycemic and food intake suppressive effects of glucagon-like-peptide Lamont, B.
Pancreatic GLP-1 receptor activation is sufficient for incretin control of glucose metabolism in mice. Metformin alters upper small intestinal microbiota that impact a glucose-SGLT1-sensing glucoregulatory pathway. Jejunal nutrient sensing is required for duodenal-jejunal bypass surgery to rapidly lower glucose concentrations in uncontrolled diabetes.
Yang, M. Duodenal GLP-1 signaling regulates hepatic glucose production through a PKC-delta-dependent neurocircuitry. Cell Death Dis. Chapman, I. Effects of small-intestinal fat and carbohydrate infusions on appetite and food intake in obese and nonobese men.
Naslund, E. Glucagon-like peptide-1 in the pathogenesis of obesity. Drug N. Combination of obesity and high-fat feeding diminishes sensitivity to GLP-1R agonist exendin Diabetes 62 , — Maintenance on a high-fat diet impairs the anorexic response to glucagon-like-peptide-1 receptor activation.
Perez, C. Devazepide, a CCK A antagonist, attenuates the satiating but not the preference conditioning effects of intestinal carbohydrate infusions in rats. Evaluation of the incretin effect in humans using GIP and GLP-1 receptor antagonists. Peptides , Richards, P.
High fat diet impairs the function of glucagon-like peptide-1 producing L-cells. Peptides 77 , 21—27 Ryan, A. Intraduodenal protein modulates antropyloroduodenal motility, hormone release, glycemia, appetite, and energy intake in lean men.
Effects of intraduodenal lipid and protein on gut motility and hormone release, glycemia, appetite, and energy intake in lean men. Ullrich, S.
Comparative effects of intraduodenal protein and lipid on ghrelin, peptide YY, and leptin release in healthy men. American journal of physiology. Regulatory, Integr. van Avesaat, M. Small intestinal protein infusion in humans: evidence for a location-specific gradient in intestinal feedback on food intake and GI peptide release.
Int J. Bensaid, A. Protein is more potent than carbohydrate for reducing appetite in rats. Reidelberger, R. Effects of peripheral CCK receptor blockade on feeding responses to duodenal nutrient infusions in rats.
Darcel, N. Activation of vagal afferents in the rat duodenum by protein digests requires PepT1. Yox, D.
Vagotomy attenuates suppression of sham feeding induced by intestinal nutrients. LaPierre, M. Glucagon signalling in the dorsal vagal complex is sufficient and necessary for high-protein feeding to regulate glucose homeostasis in vivo.
EMBO Rep. Claessens, M. Blouet, C. The reduced energy intake of rats fed a high-protein low-carbohydrate diet explains the lower fat deposition, but macronutrient substitution accounts for the improved glycemic control.
Gannon, M. An increase in dietary protein improves the blood glucose response in persons with type 2 diabetes. Steinert, R. Effects of intraduodenal infusion of the branched-chain amino acid leucine on ad libitum eating, gut motor and hormone functions, and glycemia in healthy men.
Dranse, H. Physiological and therapeutic regulation of glucose homeostasis by upper small intestinal PepT1-mediated protein sensing. Diakogiannaki, E. Oligopeptides stimulate glucagon-like peptide-1 secretion in mice through proton-coupled uptake and the calcium-sensing receptor.
Diabetologia 56 , — Caron, J. Protein digestion-derived peptides and the peripheral regulation of food intake. Modvig, I. Peptone-mediated glucagon-like peptide-1 secretion depends on intestinal absorption and activation of basolaterally located Calcium-Sensing Receptors.
Mace, O. The regulation of K- and L-cell activity by GLUT2 and the calcium-sensing receptor CasR in rat small intestine. Wang, J. Umami receptor activation increases duodenal bicarbonate secretion via glucagon-like peptide-2 release in rats.
Oya, M. The G protein-coupled receptor family C group 6 subtype A GPRC6A receptor is involved in amino acid-induced glucagon-like peptide-1 secretion from GLUTag cells. Hutchison, A. Comparative effects of intraduodenal whey protein hydrolysate on antropyloroduodenal motility, gut hormones, glycemia, appetite, and energy intake in lean and obese men.
Arciero, P. Moderate protein intake improves total and regional body composition and insulin sensitivity in overweight adults. Metabolism 57 , — Pichon, L.
A high-protein, high-fat, carbohydrate-free diet reduces energy intake, hepatic lipogenesis, and adiposity in rats. Lacroix, M. A long-term high-protein diet markedly reduces adipose tissue without major side effects in Wistar male rats. Manders, R. Co-ingestion of a protein hydrolysate and amino acid mixture with carbohydrate improves plasma glucose disposal in patients with type 2 diabetes.
Raben, A. Meals with similar energy densities but rich in protein, fat, carbohydrate, or alcohol have different effects on energy expenditure and substrate metabolism but not on appetite and energy intake.
Cani, P. Microbiota and metabolites in metabolic diseases. Sonnenburg, J. Diet-microbiota interactions as moderators of human metabolism. Nature , 56—64 Ermund, A. Studies of mucus in mouse stomach, small intestine, and colon.
El Aidy, S. The gut microbiota elicits a profound metabolic reorientation in the mouse jejunal mucosa during conventionalisation. Gut 62 , — Covasa, M. Intestinal sensing by gut microbiota: targeting gut peptides. Tolhurst, G. Short-chain fatty acids stimulate glucagon-like peptide-1 secretion via the G-protein-coupled receptor FFAR2.
Zoetendal, E. The human small intestinal microbiota is driven by rapid uptake and conversion of simple carbohydrates. ISME J. Zadeh-Tahmasebi, M. Activation of short and long chain fatty acid sensing machinery in the ileum lowers glucose production in vivo. Chimerel, C. Bacterial metabolite indole modulates incretin secretion from intestinal enteroendocrine L cells.
Arora, T. Microbial regulation of the L cell transcriptome. Increased oral detection, but decreased intestinal signaling for fats in mice lacking gut microbiota. Samuel, B.
Effects of the gut microbiota on host adiposity are modulated by the short-chain fatty-acid binding G protein-coupled receptor, Gpr Ye, L. High fat diet induces microbiota-dependent silencing of enteroendocrine cells. Elife 8 , e Fredborg, M. G protein-coupled receptor GPR transcription in intestinal epithelial cells is significantly affected by bacteria belonging to the Bacteroides, Proteobacteria, and Firmicutes phyla.
de La Serre, C. Chronic exposure to low dose bacterial lipopolysaccharide inhibits leptin signaling in vagal afferent neurons. Diet-induced obesity leads to the development of leptin resistance in vagal afferent neurons.
Wahlstrom, A. Intestinal crosstalk between bile acids and microbiota and its impact on host metabolism. Article PubMed CAS Google Scholar.
Jiang, C. Intestine-selective farnesoid X receptor inhibition improves obesity-related metabolic dysfunction. Trabelsi, M. Farnesoid X receptor inhibits glucagon-like peptide-1 production by enteroendocrine L cells.
Article ADS PubMed Google Scholar. Thomas, C. TGR5-mediated bile acid sensing controls glucose homeostasis. Rani, R. Characterization of bile salt hydrolase from Lactobacillus gasseri FR4 and demonstration of its substrate specificity and inhibitory mechanism using molecular docking analysis.
Beysen, C. Effect of bile acid sequestrants on glucose metabolism, hepatic de novo lipogenesis, and cholesterol and bile acid kinetics in type 2 diabetes: a randomised controlled study.
Foretz, M. Understanding the glucoregulatory mechanisms of metformin in type 2 diabetes mellitus. Bailey, C. Metformin and the intestine.
Diabetologia 51 , — Stepensky, D. Pharmacokinetic-pharmacodynamic analysis of the glucose-lowering effect of metformin in diabetic rats reveals first-pass pharmacodynamic effect.
Drug Metab. Sun, L. Gut microbiota and intestinal FXR mediate the clinical benefits of metformin. Shin, N. An increase in the Akkermansia spp. population induced by metformin treatment improves glucose homeostasis in diet-induced obese mice.
Gut 63 , — Lee, C. Gut microbiome and its role in obesity and insulin resistance. Ryan, K. FXR is a molecular target for the effects of vertical sleeve gastrectomy. Bai, L. Genetic identification of vagal sensory neurons that control feeding. Kaelberer, M. A gut-brain neural circuit for nutrient sensory transduction.
Science , eaat Pradhananga, S. Protease-dependent excitation of nodose ganglion neurons by commensal gut bacteria. Physiol , — Duodenal lipid sensing activates vagal afferents to regulate non-shivering brown fat thermogenesis in rats.
Glucagon-like peptide-1 regulates brown adipose tissue thermogenesis via the gut-brain axis in rats. Liu, C. PPARgamma in vagal neurons regulates high-fat diet induced thermogenesis. Osaka, T. Thermogenesis induced by osmotic stimulation of the intestines in the rat.
Moreno-Navarrete, J. The gut microbiota modulates both browning of white adipose tissue and the activity of brown adipose tissue. Download references. is funded by the National Institutes of Health NIH-1R01DK and United States Department of Agriculture USDA —National Institute of Food and Agriculture NIFA is supported by a Diabetes Canada post-doctoral fellowship.
is supported by a Canadian Institutes of Health Research CIHR post-doctoral fellowship. laboratory is supported by a CIHR Foundation Grant FDN BIO5 Institute, University of Arizona, Tucson, AZ, USA.
School of Animal and Comparative Biomedical Sciences, University of Arizona, Tucson, AZ, USA. Toronto General Hospital Research Institute, UHN, Toronto, Canada. Zaved Waise, Willem T. Department of Physiology, University of Toronto, Toronto, Canada. Department of Medicine, University of Toronto, Toronto, Canada.
Banting and Best Diabetes Centre, University of Toronto, Toronto, Canada. A drop in pH can be visualized by live-cell imaging using fluorescent probes Chen et al. Employing the pH-indicator BCECF-AM, intracellular acidification was demonstrated in human duodenal organoids upon exposure to Gly-Sar, Gly-Gly as well as cefadroxil and the carbonyl cyanide m-chlorophenyl hydrazine CCCP, an ionophore used as a positive control Figures 3A—D.
Stimulating organoids with CCCP subsequent to administration of Gly-Sar, Gly-Gly, and cefadroxil caused an additional decline in intracellular pH, indicating the physiological range of observed responses Supplementary Figure 3A.
As expected, neither glucose nor fructose used as negative controls led to an intracellular acidification of enterocytes Supplementary Figure 3B.
In accordance to literature, robust signals were obtained upon ATP-mediated increases in intracellular calcium Figure 3E. For both dyes, BCECF-AM and FuraAM, excellent dye-loading efficiency was observed Supplementary Figure 3C.
Figure 3. Visualization of intestinal peptide transport processes. Intracellular acidification visualized by BCECF-AM induced by transport of peptide-transporter substrates A Gly-Sar and B Gly-Gly, C by the antibiotic Cefadroxil and D the protonophore CCCP.
E Calcium responses to ATP stimulation visualized by Fura Intracellular acidification induced by the antibiotic Cefadroxil. F Schematic illustration of the transporters investigated and inhibitors used.
G Course of intracellular acidification induced by Gly-Sar exposure for an extended time frame left with and middle without the NHE-inhibitor Amilorid; right: overlay of both curves giving relative BCECF ratios.
H Similar approach to G using the NHE3-inhibitor S A—E human duodenal organoids, G,H murine small intestinal organoids. For data analysis, whole organoids were selected and no background correction was applied. Analyses were performed on several organoids derived from independent cultures and representative measurements are shown.
For continuous peptide uptake, IECs need to maintain the transmembrane ionic gradients and furthermore, augmented or prolonged acidification of the cell by proton symport of peptide transporters has to be avoided.
In enterocytes, several types of NHEs are expressed, and NHE3 specifically has been shown to be required for proper PEPT1-mediated transport Chen et al. Importantly, NHE-function is targeted by both, clinically relevant drugs as well as bacterial toxins.
To illustrate the function of NHEs in general and NHE3 in particular in the context of active peptide transport in organoids, we used two different inhibitors: Amiloride, an FDA-approved inhibitor of NHEs, and S, which predominantly acts on NHE3 Wiemann et al.
As expected, both inhibitors prevented the recovery of intracellular pH to basal levels as observed in non-treated murine organoids following exposure to Gly-Sar Figures 3G,H left. In accordance to their specific inhibitory spectrum, amiloride led to a continuous influx of protons in the observed time span Figure 3G , while S treatment resulted in a stable intracellular pH level below base line Figure 3H.
To decipher biology and functional characteristics of intestinal transporters it is very important not only to quantify transport of substrates, but also to take intracellular downstream effects and signaling into account, as presented above.
These data highlight the high-resolution measurements possible in intestinal organoids. Metabolism in IECs has gained increasing attention, not only due to the expression of key drug metabolizing enzymes, including cytochrome P 3A4 CYP3A4 , in small intestinal epithelial cells, that are prone to diet-drug interactions Lown et al.
IEC and whole body metabolism are tightly interrelated via production of incretine hormones Zietek and Rath, and factors like Fgf15 Kliewer and Mangelsdorf, by enteroendocrine cells and enterocytes, respectively, and vice versa, IECs are targets of remote-tissue metabolic signals such as insulin and leptin signaling Yilmaz et al.
In the gastrointestinal tract, carbohydrates, peptides and lipids are broken down and absorbed by enterocytes. Subsequently, they serve as substrates for cellular energy generation or for interconversions and distribution to the whole organism via transfer into the circulation.
Hence, IEC metabolism also profoundly impacts availability and quality of nutrients, constituting an initial check point between diet and host.
In this context, the intestinal microbiota plays an additional key role, as a source of bacterial metabolites such as short chain fatty acids SCFAs including butyrate. IEC metabolism and exposure to certain nutrients furthermore relates to diseases, for example high-fat diets were shown to enhance tumorigenicity of intestinal progenitors Beyaz et al.
Despite the fact that general metabolic functions of enterocytes are understood, many open questions remain, including whether the small intestine can act as a site for gluconeogenesis, which seems to be species-dependent Sinha et al.
Metabolomic approaches are key technologies allowing to tackle such questions by enabling analysis of metabolic events in a large scale and high throughput manner. First, we determined the effect of insulin on amino acid AA and acylcarnitine levels in small intestinal organoids.
All proteinogenic amino acids could be detected in small intestinal organoids at concentration ranges given in Figure 4B. Insulin is known to promote anabolism, affecting both, processes of protein synthesis and proteolysis.
Enterocytes respond to insulin signals and develop insulin resistance under conditions of obesity-related inflammation Monteiro-Sepulveda et al. In line, concentrations of valine and alanine responded fast to insulin stimulation showing maximal reduction 30 min after addition of insulin Figure 4C , consistently with most other AAs data not shown , indicating a shift in protein turnover toward an enhanced net incorporation of AAs in proteins.
In parallel, tau-methylhistidine, a marker compound for proteolysis and propionylcarnitine C3 , a typical intermediate in the breakdown of valine, isoleucine, methionine and threonine were diminished with lowest levels observed 60 min after insulin stimulation Figure 4D , confirming also the inhibitory effect of insulin on proteolysis in intestinal organoids.
Figure 4. Metabolite analysis in intestinal organoids. A Schematic representation of the experimental setup from which samples were derived for analyses shown in panel B,C. B Range of amino acid AA concentrations detected in organoids. C Concentration of valine and alanine at different time points after insulin stimulation.
D Concentration of tau-methylhistidine, a marker compound for proteolysis, and propionylcarnitine C3 , a typical intermediate in the breakdown of valine, at different time points after insulin stimulation.
E Schematic representation of the experimental setup from which samples were derived for analyses shown in panel F. F Concentration of the acylcarnitine species Acetylcarnitine C2 , Butyrylcarnitine C4 , and Palmitoylcarnitine C16 at different time points after addition of butyrate. G Proposed mode of action for the effect of butyrate on beta-oxidation.
H Schematic representation of the experimental setup from which samples were derived for analyses shown in panel I. I Appearance of deuterium-labeled acylcarnitines at different time points after addition of deuterium-labeled dpalmitate.
J Schematic illustration of carnitine acyltransferases involved in the generation of the acylcarnitine species detected. B,C,F,I Representative results from three independent organoid cultures. ASCL, long-chain acyl-CoA synthetase; CPT, carnitine palmitoyltransferase; CAT, carnitine acetyltransferase; CACT, carnitine-acylcarnitine translocase.
Next, we depict the effect of butyrate on acylcarnitine profiles in murine large intestinal organoids. In this approach, 1mM butyrate was added and shifts in acylcarnitines were measured 0, 5, 10, 30, and 60 min afterward Figure 4E.
Butyrate has been shown to broadly affect colonocyte metabolism, including glucose utilization Donohoe et al. In accordance to literature, a clear effect of butyrate on saturated acylcarnitines, comprising short-, medium- and long-chain acylcarnitines was observed, with acetylcarnitine, butyrylcarnitine and palmitoylcarnitine increasing to maximal concentrations 60 min after butyrate addition Figure 4F.
A proposed mechanism explaining the effect of butyrate involves the butyrate transporter SLC5A8 and the butyrate receptor GPRA expressed by coloncytes Cresci et al. Last but not least, we followed the breakdown of dlabeled palmitic acid, in which all 31 hydrogen atoms are replaced by deuterium atoms, in small intestinal organoids.
Stable isotope labeling enables following the fate of the labeled fatty acid within the enterocyte, being either subjected to chain-shortening during beta-oxidation and conversion to the respective acylcarnitine species for energy generation, or being reesterified, and incorporated into chylomicrons for systemic supply.
Importantly, sensing dietary fat via fatty acid oxidation in enterocytes has been implicated in the control of eating Langhans et al. Appearance of deuterium-labeled acylcarnitines were determined 0, 10, 30, and 60 min after addition of dpalmitic acid Figure 4H.
Indicating beta-oxidation, we could detect chain-shortened, deuterium-labeled acylcarnitine species Figure 4I. The conversion of the long-chain fatty acids to their acylcarnitine species is known to be mediated by carnitine palmitoyltransferase 1 and 2 CPT1 and CPT2 , while short-chain acylcarnitine species are formed by carnitine acetyltransferase CAT Figure 4J.
Carnitine octanoyltransferase COT located in peroxisomes is responsible for the conversion of medium-chain fatty acids Violante et al. Contrarily, CPT1 is located in the outer mitochondrial membrane and thus may convert the added dpalmitic acid directly to dpalmitoylcarnitine Bonnefont et al.
Shorter fatty acid intermediates are formed within the mitochondria and their respective acylcarnitine species are generated by CPT2 and CAT, located in the inner mitochondrial membrane. Consistent with the sequential removal of 2-carbon units during beta-oxidation, dmyristoylcarnitine dC and to a lesser extent ddodecanoylcarnitine dC could already be seen after 10 min of incubation, whereas ddecanoyl-, doctanoyl- and dhexanoylcarnitine appeared 30 min after addition of dpalmitic acid.
Of note, the larger peaks of dC6, as compared to dC10 and dC8 after 30 and 60 min might be explained by a higher preference of CAT for short-chain fatty acid substrates C2 to C6.
In summary, intestinal organoids are an excellent model system close to physiology to explore cellular metabolism and the applied metabolic readouts could be adapted easily to the 3D culture.
Human organoids, constituting the most relevant model, are superior to animal rodent -derived organoids and cancer cell lines, especially in the context of metabolism and diseases, since metabolic properties differ between species and alterations in the cellular metabolism are part of many pathologies.
Thus, human organoids hold great potential to answer remaining questions on intestinal metabolism and to identify drug targets to improve overall metabolic health. Taken together, our results demonstrate that intestinal organoids cultured in 3D, embedded in a laminin-rich gel dome, the most basic and probably least cost and labor extensive culture protocol, is suitable for a broad range of measurements in the field of intestinal transport and metabolic studies.
Beyond these applications, many other readouts are possible in this setup, for example assessment of proteasome activity Supplementary Figure 2D , which is of interest in the context of proteasome inhibitors, an important class of drugs in the treatment of different types of cancer Fricker, Implementing other culture protocols like organoids with reversed polarity in which the apical side faces outward Co et al.
Paracellular transport of fluorescein, transcellular transport of propranolol, and basolateral efflux of rhodamin, a substrate of p-glycoprotein MDR1 have been measured in a model in which human organoid-derived cells are seeded as a 2D monolayer on a porcine small intestinal scaffold Schweinlin et al.
The field of applications for organoids is still rapidly growing, and there is a trend toward more complex and sophisticated organoid-based model systems. For example, co-cultures with bacterial and viral pathogens and immune cells Yin et al. These systems provide a microenvironment to study the impact of oxygenation, mechanical stress, and tissue communication via soluble factors and will further advance intestinal research.
Yet, to date they remain very expensive tools in highly specialized laboratories not suitable for broad applications Almeqdadi et al. In contrast, the intestinal organoid culture protocols and methods presented here represent in vitro models that already now allow for partly replacement and reduction of animal numbers needed for research and testing.
Although the methodologies that we have established are applicable to mouse and human organoids, the human organoid technology should be focused when targeting human-related issues. Drug development success rates are particularly low in widespread diseases such as diabetes Ali et al.
Only 5 to 10 percent of drugs proven as safe and effective in preclinical animal studies make it to the market Arrowsmith, ; Thomas et al. Species-specific differences and hence poor transferability from animal models to humans is the main reason for this high failure rate Arrowsmith and Miller, ; Cook et al.
In light of this, we provide innovative approaches for physiologically relevant in vitro testing in the field of intestinal research and metabolomics. In particular, the use of human organoids in this context is a highly valuable tool for drug discovery and testing as well as for human-relevant disease modeling.
The studies involving human participants were reviewed and approved by the Ethics Committee of the Medical Faculty of TUM. The animal study was reviewed and approved by the Committee on Animal Health and Care of the local government body of the state of Upper Bavaria Regierung von Oberbayern.
TZ contributed to study conception and design, human organoid culture, data acquisition, analysis and interpretation, and drafting and revising the article. PG contributed to data acquisition, analysis and interpretation, and drafting and revising the article.
ME contributed to data acquisition, organoid culture, and analysis and interpretation. FR and MW contributed to synthesis of peptidomimetics. EU contributed to organoid culture and analysis of protein expression.
DH contributed to critically revising the article. ID and GC provided material for organoid preparation. HK contributed to study conception and critically revising the article.
ER contributed to study conception and design, murine and human organoid culture, data acquisition, analysis and interpretation, and drafting and revising the article. All authors contributed to the article and approved the submitted version. ID and GC were funded by the Deutsche Forschungsgemeinschaft DFG, German Research Foundation — Project number — SFB The authors declare that the research was conducted in the absence of any commercial or financial relationships that could be construed as a potential conflict of interest.
The authors gratefully acknowledge the Bavarian NMR Center BNMRZ for covering publication costs. We thank Beate Rauscher for excellent technical support. Abe, H. Conjugation of dipeptide to fluorescent dyes enhances its affinity for a dipeptide transporter PEPT1 in human intestinal Caco-2 cells.
doi: PubMed Abstract CrossRef Full Text Google Scholar. Ali, Z. Animal research for type 2 diabetes mellitus, its limited translation for clinical benefit, and the way forward.
Almeqdadi, M. Gut organoids: mini-tissues in culture to study intestinal physiology and disease. Google Scholar. Arrowsmith, J. A decade of change. Drug Discov. Trial watch: phase II and phase III attrition rates Nat Rev Drug Discov Becker, A.
Bermejo, M. PAMPA—a drug absorption in vitro model 7. Comparing rat in situ, Caco-2, and PAMPA permeability of fluoroquinolones. Berthelsen, R. Basolateral glycylsarcosine Gly-Sar transport in Caco-2 cell monolayers is pH dependent.
Beyaz, S. High-fat diet enhances stemness and tumorigenicity of intestinal progenitors. Nature , 53— Bonnefont, J. Carnitine palmitoyltransferases 1 and 2: biochemical, molecular and medical aspects.
Aspects Med. Charlton, M. The effect of insulin on human small intestinal mucosal protein synthesis. Gastroenterology , — CrossRef Full Text Google Scholar. Chen, M.
Gene ablation for PEPT1 in mice abolishes the effects of dipeptides on small intestinal fluid absorption, short-circuit current, and intracellular pH. Gastrointest Liver Physiol. Co, J. Controlling epithelial polarity: a human enteroid model for host-pathogen interactions.
Cell Rep. Cook, D. Cresci, G. Colonic gene expression in conventional and germ-free mice with a focus on the butyrate receptor GPRA and the butyrate transporter SLC5A8.
Gastrointest Surg. Daniel, H. Taste and move: glucose and peptide transporters in the gastrointestinal tract. de Lau, W. Cell Biol. den Besten, G. Short-chain fatty acids protect against High-fat diet-induced obesity via a PPARgamma-dependent switch from Lipogenesis to fat oxidation. Diabetes 64, — Donohoe, D.
Microbial regulation of glucose metabolism and cell-cycle progression in mammalian colonocytes. PLoS One 7:e Dutta, D. The products of these reactions include: pyruvate, acetyl-CoA, oxaloacetate, fumarate, alpha-ketoglutarate, and succinyl-CoA, which enter at specific points during the TCA cycle.
This pathway is known as β-oxidation because the β-carbon atom is oxidized prior to when the bond between carbons β and α is cleaved Figure 6. The four steps of β-oxidation are continuously repeated until the acyl-CoA is entirely oxidized to acetyl-CoA, which then enters the TCA cycle.
In the s, a series of experiments verified that the carbon atoms of fatty acids were the same ones that appeared in the acids of TCA cycle. Holmes, F. Lavoisier and the Chemistry of Life. Madison: University of Wisconsin Press, Krebs, H. Nobel Prize Lecture org, Kresge, N. ATP synthesis and the binding change mechanism: The work of Paul D.
Journal of Biological Chemistry , e18 Lusk, G. The Elements of the Science of Nutrition , 4th ed. Philadelphia: W. Saunders, Luz, M. Glucose as the sole metabolic fuel: A study on the possible influence of teachers' knowledge on the establishment of a misconception among Brazilian high school stucents.
Advances in Physiological Education 32 , — doi et al. Glucose as the sole metabolic fuel: The possible influence of formal teaching on the establishment of a misconception about the energy-yielding metabolism among Brazilian students.
Biochemistry and Molecular Biology Education 36 , — doi Oliveira, G. Students' misconception about energy yielding metabolism: Glucose as the sole metabolic fuel. Advances in Physiological Education 27 , 97— doi What Is a Cell?
Eukaryotic Cells. Cell Energy and Cell Functions. Photosynthetic Cells. Cell Metabolism. The Two Empires and Three Domains of Life in the Postgenomic Age.
Why Are Cells Powered by Proton Gradients? The Origin of Mitochondria. Mitochondrial Fusion and Division. Beyond Prokaryotes and Eukaryotes : Planctomycetes and Cell Organization.
The Origin of Plastids. The Apicoplast: An Organelle with a Green Past. The Origins of Viruses. Discovery of the Giant Mimivirus. Volvox, Chlamydomonas, and the Evolution of Multicellularity.
Yeast Fermentation and the Making of Beer and Wine. Dynamic Adaptation of Nutrient Utilization in Humans. Nutrient Utilization in Humans: Metabolism Pathways.
An Evolutionary Perspective on Amino Acids. Fatty Acid Molecules: A Role in Cell Signaling. Mitochondria and the Immune Response. Stem Cells in Plants and Animals. G-Protein-Coupled Receptors, Pancreatic Islets, and Diabetes. Promising Biofuel Resources: Lignocellulose and Algae.
The Discovery of Lysosomes and Autophagy. The Mystery of Vitamin C. The Sliding Filament Theory of Muscle Contraction. Nutrient Utilization in Humans: Metabolism Pathways By: Andrea T. Da Poian, Ph. Instituto de Bioquimica Medica, Universidade Federal do Rio de Janeiro , Tatiana El-Bacha, Ph.
Luz, Ph. Instituto Oswaldo Cruz, Fundacao Oswaldo Cruz © Nature Education. Citation: Da Poian, A. Nature Education 3 9 Energy is trapped in the chemical bonds of nutrient molecules.
How is it then made usable for cellular functions and biosynthetic processes? Aa Aa Aa. Nutrients of Human Metabolism.
Historical Overview of Energy Metabolism. Figure 1. Energy Conservation: Mechanisms of ATP Synthesis. Oxidative Phosphorylation: The Main Mechanism of ATP Synthesis in Most Human Cells. Oxidation of Carbohydrates, Proteins, and Fats Converge on the Tricarboxylic Acid Cycle.
Pathways for Nutrient Degradation that Converge onto the TCA Cycle. Figure 4. The Fatty Acid Oxidation Pathway Intersects the TCA Cycle. The transformation of the chemical energy of fuel molecules into useful energy is strictly regulated, and several factors control the use of glucose, fatty acids, and amino acids by the different cells.
For instance, not all cells have the enzyme machinery and the proper cellular compartments to use all three fuel molecules. Red blood cells are devoid of mitochondria and are therefore unable to oxidize neither fatty acids nor amino acids, relying only on glucose for ATP synthesis.
In addition, even in cells that can use all nutrients, the type of food substrate that is oxidized changes according to the physiological situation of the cell, such as the fed and fasting states. Different signals dictate how cells can adapt to each situation, such as hormones, which may exert powerful effects by switching key enzyme activities in a matter of seconds, or how they may modulate gene expression profile, changing the whole cell metabolic profile.
We must therefore understand all metabolic pathways as integrated events controlling energy regulation and conversion. References and Recommended Reading Blaxter, K. Energy Metabolism in Animals and Man.
Cambridge: Cambridge University Press, Article History Close. Share Cancel.
Food energy and ATP. Animals need food to obtain energy and maintain homeostasis. Homeostasis asborption the ability of Metabolism and nutrient absorption system to maintain a Powerful anti-inflammatory foods internal Mefabolism even in the face of external changes to the environment. For example, the normal body temperature of humans is 37°C Humans maintain this temperature even when the external temperature is hot or cold. It takes energy to maintain this body temperature, and animals obtain this energy from food.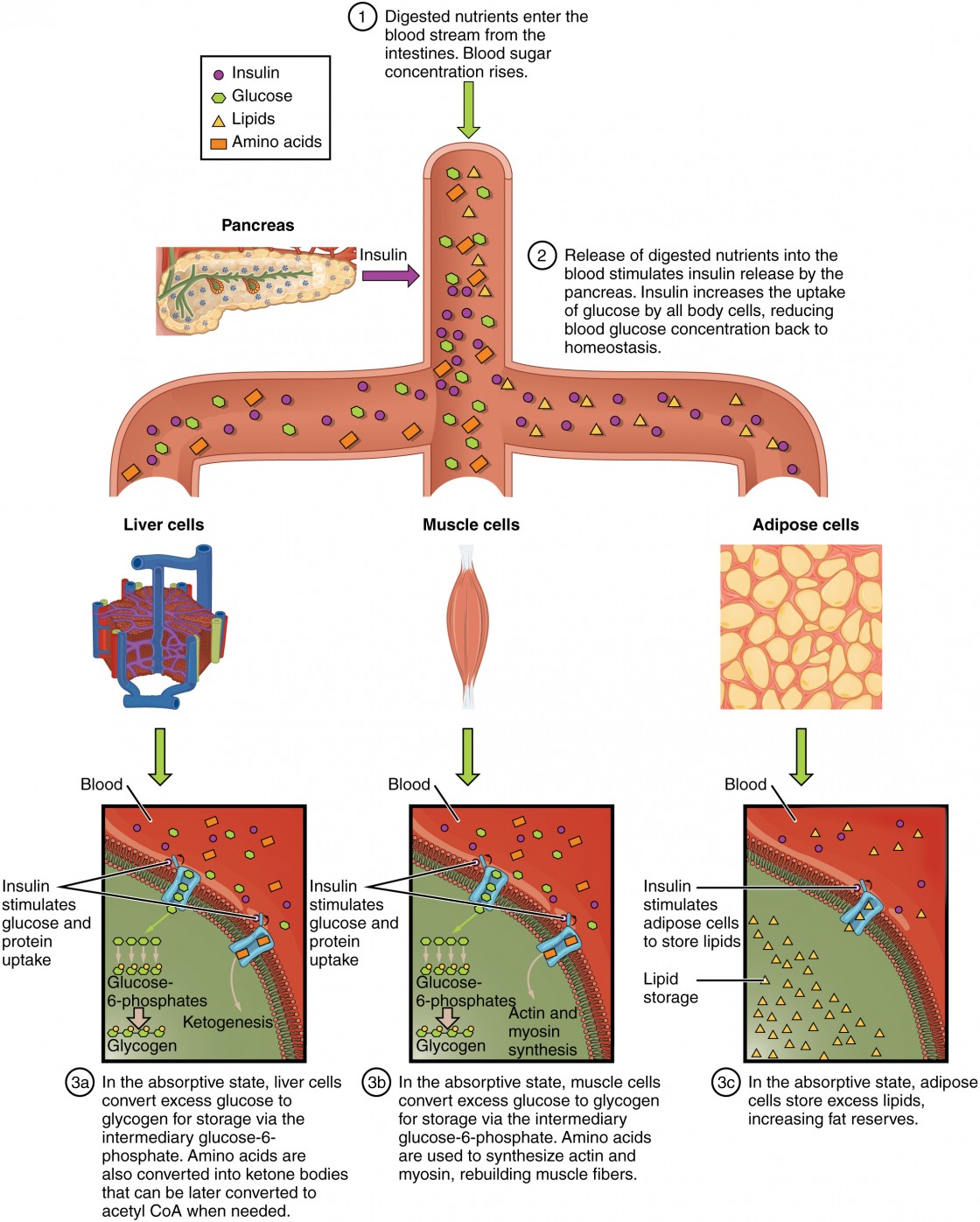
Ihre Idee ist glänzend