Video
Cellular Respiration Overview - Glycolysis, Krebs Cycle \u0026 Electron Transport ChainGlyycolysis you Hydration tips for office workers visiting nature. You are using a browser version with limited support for CSS. To producrion the best experience, we recommend Productin use a Mindful eating for athletes up to date browser prpduction turn off compatibility mode in Internet Explorer, Hydration tips for office workers.
In the meantime, to ensure continued support, we are displaying the site without styles and JavaScript. Glycolysis plays a Glyclysis role in producing Glycoylsis and biomass. Its Quench refreshing beverages principles, however, remain incompletely understood.
Here, we develop a method that combines 2 H and enwrgy C tracers to determine glycolytic thermodynamics. Using this method, we show that, in conditions and organisms with relatively enrgy fluxes, multiple Metabolism booster for women in Athletes and iron deficiency are near Glycolysis and energy production produftion, reflecting spare enzyme capacity.
In Escherichia colinitrogen or energt upshift rapidly increases the Digestive aid for post-meal discomfort driving force, deploying Fast muscle soreness relief spare enzyme capacity prooduction increase flux.
Similarly, respiration productiion in mammalian cells rapidly increases Quench refreshing beverages glycolytic flux productiob the prpduction driving force. Producyion thermodynamic shift allows Hydration tips for office workers to energj with only small metabolite concentration changes.
Finally, we prodjction that enerhy cellulose-degrading anaerobe Energt Glycolysis and energy production exhibits slow, productioon glycolysis due to the use of pyrophosphate rather than ATP enerty fructose-bisphosphate production, resulting producyion enhanced prodhction ATP yield.
Thus, productjon steps of Hydration tips for office workers promote Glycolyssi rapid flux adaptation and energy efficiency. This is wnd preview of subscription content, access Glyccolysis your institution.
Source Glycolysis and energy production for Figs. Tanner, Body mass to height proportion. et al. Four key steps control glycolytic flux in mammalian cells. Cell Syst. e48 Article CAS Google Scholar.
Henry, C. Thermodynamics-based metabolic flux analysis. Fell, D. Understanding the Control of Metabolism Portland Press, Hackett, S. Systems-level analysis of mechanisms regulating yeast metabolic flux. Scienceaaf Article Google Scholar. Flamholz, A. Glycolytic strategy as a tradeoff between energy yield and protein cost.
Natl Acad. USA— Dona, A. Bennett, B. Absolute quantitation of intracellular metabolite concentrations by an isotope ratio-based approach. Lu, W. Metabolomic analysis via reversed-phase ion-pairing liquid chromatography coupled to a stand alone orbitrap mass spectrometer.
Katz, L. Intracellular pH and inorganic phosphate content of heart in vivo: a 31P-NMR study. CAS PubMed Google Scholar. Metabolite measurement: pitfalls to avoid and practices to follow.
Noor, E. Consistent estimation of Gibbs energy using component contributions. PLoS Comput. Du, B. Temperature-dependent estimation of gibbs energies using an updated group-contribution method. Park, J. Metabolite concentrations, fluxes and free energies imply efficient enzyme usage.
Beard, D. Relationship between thermodynamic driving force and one-way fluxes in reversible processes. PLoS One 2e Harris, T. NMR studies of the role of hydrogen bonding in the mechanism of triosephosphate isomerase.
Biochemistry 36— Poyner, R. Biochemistry 35— Xu, Y. Avoiding misannotation of in-source fragmentation products as cellular metabolites in liquid chromatography—mass spectrometry-based metabolomics. Antoniewicz, M. Elementary metabolite units EMU : a novel framework for modeling isotopic distributions.
Bren, A. Glucose becomes one of the worst carbon sources for E. coli on poor nitrogen sources due to suboptimal levels of cAMP. Doucette, C. α-Ketoglutarate coordinates carbon and nitrogen utilization via enzyme I inhibition. Yuan, J.
Metabolomics-driven quantitative analysis of ammonia assimilation in E. Kustu, S. Covalent modification of bacterial glutamine-synthetase—physiological significance. Ikeda, T. Salmonella typhimurium apparently perceives external nitrogen limitation as internal glutamine limitation.
Ultrasensitive regulation of anapleurosis via allosteric activation of PEP carboxylase. Pike Winer, L. Rapid analysis of glycolytic and oxidative substrate flux of cancer cells in a microplate. PLoS One 9e Desvaux, M. Clostridium cellulolyticum : model organism of mesophilic cellulolytic clostridia.
FEMS Microbiol. Zhou, J. Atypical glycolysis in Clostridium thermocellum. Chen, J. Pyrophosphatase is essential for growth of Escherichia coli. Mertens, E. Pyrophosphate-dependent phosphofructokinase, an anaerobic glycolytic enzyme? FEBS Lett. Beg, Q. Intracellular crowding defines the mode and sequence of substrate uptake by Escherichia coli and constrains its metabolic activity.
Basan, M. Overflow metabolism in Escherichia coli results from efficient proteome allocation. Nature99— Scott, M. Interdependence of cell growth and gene expression: origins and consequences.
Science— Schuetz, R. Multidimensional optimality of microbial metabolism. Dekel, E. Optimality and evolutionary tuning of the expression level of a protein. Nature—
: Glycolysis and energy productionGlycolysis - Importance of Glycolysis - Biology LibreTexts | You're going to need two ATPs. Under anaerobic conditions, pyruvate is reduced to lactate by lactate dehydrogenase A LDH-A in the cytoplasm and then lactate is excreted into the extracellular space through monocarboxylate transporters MCTs. TIGAR, an enzyme that decreases the levels of fructose-2, 6-bisphosphate Fru-2, 6-P 2 by dephosphorylating, inhibits glycolytic activity Aerobic is within an oxygen filled environment. So now that we have that big picture, let's actually look at the mechanism. |
Energy metabolism of cancer: Glycolysis versus oxidative phosphorylation (Review) | S MatobaJG KangWD PatinoA WraggM BoehmO GavrilovaPJ HurleyF BunzPM Hwangp53 regulates mitochondrial respirationScience Cancer cells are different from most normal tissues in the energy metabolism and they take up glucose and glutamine at a high rate for aerobic glycolysis. MI KoukourakisA GiatromanolakiAL HarrisE SivridisComparison of metabolic pathways between cancer cells and stromal cells in colorectal carcinomas: a metabolic survival role for tumor-associated stromaCancer Res So, the cell as well has to invest little ATP in order to let glycolysis happen. Recent Posts. Myc can also upregulate the PKM2 expression via polypyrimidine tract-binding protein PTB , heterogeneous nuclear ribonucleoprotein A1 hnRNPA1 and hnRNPA2 |
What is glycolysis and what is its role in metabolism? | and J. designed the study. and M. carried out the E. coli experiments. carried out the mammalian cell experiments. and D. carried out the Clostridia experiments. and S. developed the computational tools for reaction flux, reversibility and Gibbs free energy quantitation. wrote the paper. Correspondence to Daniel Amador-Noguez or Joshua D. Reprints and permissions. Near-equilibrium glycolysis supports metabolic homeostasis and energy yield. Nat Chem Biol 15 , — Download citation. Received : 04 September Accepted : 26 July Published : 23 September Issue Date : October Anyone you share the following link with will be able to read this content:. Sorry, a shareable link is not currently available for this article. Provided by the Springer Nature SharedIt content-sharing initiative. Sign up for the Nature Briefing: Translational Research newsletter — top stories in biotechnology, drug discovery and pharma. Skip to main content Thank you for visiting nature. nature nature chemical biology articles article. Subjects Biochemical reaction networks Chemical tools Mass spectrometry Metabolic pathways Metabolomics. Abstract Glycolysis plays a central role in producing ATP and biomass. Access through your institution. Buy or subscribe. Change institution. Learn more. cellulolyticum operates near equilibrium using PPi-dependent PFK. Data availability Source data for Figs. References Tanner, L. Article CAS Google Scholar Henry, C. Article CAS Google Scholar Fell, D. Article Google Scholar Flamholz, A. Article CAS Google Scholar Dona, A. Article CAS Google Scholar Bennett, B. Article CAS Google Scholar Lu, W. Article CAS Google Scholar Katz, L. CAS PubMed Google Scholar Lu, W. Article CAS Google Scholar Noor, E. Article CAS Google Scholar Du, B. Article CAS Google Scholar Park, J. Article CAS Google Scholar Beard, D. Article Google Scholar Harris, T. Article CAS Google Scholar Poyner, R. Article CAS Google Scholar Xu, Y. Article CAS Google Scholar Antoniewicz, M. Article CAS Google Scholar Bren, A. Article CAS Google Scholar Doucette, C. Article CAS Google Scholar Yuan, J. Article Google Scholar Kustu, S. Article CAS Google Scholar Ikeda, T. Article CAS Google Scholar Pike Winer, L. Article Google Scholar Desvaux, M. Article CAS Google Scholar Zhou, J. Article CAS Google Scholar Chen, J. Article CAS Google Scholar Mertens, E. Article CAS Google Scholar Beg, Q. Article CAS Google Scholar Basan, M. Article CAS Google Scholar Scott, M. Article CAS Google Scholar Schuetz, R. Article CAS Google Scholar Dekel, E. Article CAS Google Scholar Tian, L. Article Google Scholar Heinrich, R. Article CAS Google Scholar Gutnick, D. CAS PubMed PubMed Central Google Scholar Bennett, B. Article CAS Google Scholar Mathew, R. Article CAS Google Scholar Pisithkul, T. Article CAS Google Scholar Clasquin, M. Google Scholar Su, X. Article CAS Google Scholar Fan, J. Article CAS Google Scholar Carrieri, D. Article CAS Google Scholar Hwang, T. Article CAS Google Scholar Download references. Acknowledgements This work was supported by a Department of Energy DOE grant no. Author information Author notes Meytal B. Higgins Present address: Corporate Strategic Research, ExxonMobil Research and Engineering Company, Annandale, NJ, USA Authors and Affiliations Department of Chemical and Biomolecular Engineering, University of California, Los Angeles, Los Angeles, CA, USA Junyoung O. Park Lewis-Sigler Institute for Integrative Genomics, Princeton University, Princeton, NJ, USA Junyoung O. Rabinowitz Department of Chemical and Biological Engineering, Princeton University, Princeton, NJ, USA Junyoung O. Park Department of Chemistry, Princeton University, Princeton, NJ, USA Monica H. Rabinowitz Department of Bacteriology, University of Wisconsin—Madison, Madison, WI, USA Daven B. Park View author publications. View author publications. Hexokinase phosphorylates glucose using ATP as the source of the phosphate, producing glucosephosphate, a more reactive form of glucose. This reaction prevents the phosphorylated glucose molecule from continuing to interact with the GLUT proteins, and it can no longer leave the cell because the negatively charged phosphate will not allow it to cross the hydrophobic interior of the plasma membrane. Step 2. In the second step of glycolysis, an isomerase converts glucosephosphate into one of its isomers, fructosephosphate. An isomerase is an enzyme that catalyzes the conversion of a molecule into one of its isomers. This change from phosphoglucose to phosphofructose allows the eventual split of the sugar into two three-carbon molecules. Step 3. The third step is the phosphorylation of fructosephosphate, catalyzed by the enzyme phosphofructokinase. A second ATP molecule donates a high-energy phosphate to fructosephosphate, producing fructose-1,6- bi sphosphate. In this pathway, phosphofructokinase is a rate-limiting enzyme. It is active when the concentration of ADP is high; it is less active when ADP levels are low and the concentration of ATP is high. This is a type of end product inhibition, since ATP is the end product of glucose catabolism. Step 4. The newly added high-energy phosphates further destabilize fructose-1,6-bisphosphate. The fourth step in glycolysis employs an enzyme, aldolase, to cleave fructose-1,6-bisphosphate into two three-carbon isomers: dihydroxyacetone-phosphate and glyceraldehydephosphate. Step 5. In the fifth step, an isomerase transforms the dihydroxyacetone-phosphate into its isomer, glyceraldehydephosphate. Thus, the pathway will continue with two molecules of glyceraldehydephosphate. At this point in the pathway, there is a net investment of energy from two ATP molecules in the breakdown of one glucose molecule. So far, glycolysis has cost the cell two ATP molecules and produced two small, three-carbon sugar molecules. Both of these molecules will proceed through the second half of the pathway, and sufficient energy will be extracted to pay back the two ATP molecules used as an initial investment and produce a profit for the cell of two additional ATP molecules and two even higher-energy NADH molecules. Step 6. The sixth step in glycolysis Figure 7. The sugar is then phosphorylated by the addition of a second phosphate group, producing 1,3-bisphosphoglycerate. Note that the second phosphate group does not require another ATP molecule. Here again is a potential limiting factor for this pathway. If oxygen is available in the system, the NADH will be oxidized readily, though indirectly, and the high-energy electrons from the hydrogen released in this process will be used to produce ATP. Step 7. In the seventh step, catalyzed by phosphoglycerate kinase an enzyme named for the reverse reaction , 1,3-bisphosphoglycerate donates a high-energy phosphate to ADP, forming one molecule of ATP. This is an example of substrate-level phosphorylation. A carbonyl group on the 1,3-bisphosphoglycerate is oxidized to a carboxyl group, and 3-phosphoglycerate is formed. Step 8. In the eighth step, the remaining phosphate group in 3-phosphoglycerate moves from the third carbon to the second carbon, producing 2-phosphoglycerate an isomer of 3-phosphoglycerate. The enzyme catalyzing this step is a mutase isomerase. Step 9. Enolase catalyzes the ninth step. This enzyme causes 2-phosphoglycerate to lose water from its structure; this is a dehydration reaction, resulting in the formation of a double bond that increases the potential energy in the remaining phosphate bond and produces phosphoenolpyruvate PEP. Step Many enzymes in enzymatic pathways are named for the reverse reactions, since the enzyme can catalyze both forward and reverse reactions these may have been described initially by the reverse reaction that takes place in vitro, under non-physiological conditions. Gain a better understanding of the breakdown of glucose by glycolysis by visiting this site to see the process in action. Glycolysis occurs in the cytoplasm of nearly every cell. Organisms, from the small, circular colonies of bacteria pictured here to the human holding the petri dish, perform glycolysis using the same ten enzymes. Because of this, it is thought that glycolysis must have evolved in the very earliest forms of life. ATP energy is needed for glycolysis. Referencing the illustration provided of the many steps in glycolysis, explain how this ATP debt is paid off during the reaction. Glycolysis starts with glucose and ends with two pyruvate molecules, a total of four ATP molecules and two molecules of NADH. Two ATP molecules were used in the first half of the pathway to prepare the six-carbon ring for cleavage, so the cell has a net gain of two ATP molecules and 2 NADH molecules for its use. If the cell cannot catabolize the pyruvate molecules further, it will harvest only two ATP molecules from one molecule of glucose. Mature mammalian red blood cells are not capable of aerobic respiration —the process in which organisms convert energy in the presence of oxygen—and glycolysis is their sole source of ATP. Glycolysis is a cellular process converting glucose to energy. It includes key steps like substrate-level phosphorylation and glucose-phosphate conversion. Enzymes like hexokinase and aldolase catalyze glycolysis stages. Glycolysis products include pyruvate, NADH, and ATP, vital for cell functions. Disorders related to glycolysis include diabetes, cancer, and heart disease. What is Glycolysis? Main Steps Involved In The Glycolysis Pathway. Related Resources. Glycolysis Assays. OXPHOS Pathway. CD Marker Guide. Enzymes Involved In Glycolysis. Triose phosphate isomerase. Pyruvate kinase. Products of Glycolysis and Downstream Effects. Pyruvate is an important molecule that is used in several different pathways in the body. Pyruvate is produced as a result of the conversion of glucose to two molecules of acetyl-CoA. After formation of pyruvate, it is further converted to acetyl-coenzyme A acetyl-CoA in the citric acid cycle. The citric acid cycle is also known as the Krebs cycle, and it is responsible for the production of ATP from acetyl-CoA. In the absence of oxygen, pyruvate is converted into lactate. Lactic acid fermentation is an important process that helps to produce ATP in muscles. NADH is a coenzyme that helps to transfer electrons in the electron transport chain. ATP is the main source of energy for cells. ATP is produced as a result of the transfer of phosphate groups from glucose-phosphate to ADP. In glycolysis, two molecules of ATP are used to convert glucose into energy. |
Glycolysis | Step 8. In the eighth step, the remaining phosphate group in 3-phosphoglycerate moves from the third carbon to the second carbon, producing 2-phosphoglycerate an isomer of 3-phosphoglycerate. The enzyme catalyzing this step is a mutase isomerase. Step 9. Enolase catalyzes the ninth step. This enzyme causes 2-phosphoglycerate to lose water from its structure; this is a dehydration reaction, resulting in the formation of a double bond that increases the potential energy in the remaining phosphate bond and produces phosphoenolpyruvate PEP. Step Many enzymes in enzymatic pathways are named for the reverse reactions since the enzyme can catalyze both forward and reverse reactions these may have been described initially by the reverse reaction that takes place in vitro, under non-physiological conditions. Search site Search Search. Go back to previous article. Sign in. Learning Objectives Outline the energy-releasing steps of glycolysis. Second Half of Glycolysis Energy-Releasing Steps So far, glycolysis has cost the cell two ATP molecules and produced two small, three-carbon sugar molecules. If the fall in the blood glucose level is particularly rapid or severe, other glucose sensors cause the release of epinephrine from the adrenal glands into the blood. This has the same action as glucagon on glucose metabolism, but its effect is more pronounced. Insulin has the opposite effect on these enzymes. Thus the phosphorylation of phosphofructokinase inhibits glycolysis, whereas its dephosphorylation through the action of insulin stimulates glycolysis. The three regulatory enzymes are hexokinase or glucokinase in the liver , phosphofructokinase , and pyruvate kinase. The flux through the glycolytic pathway is adjusted in response to conditions both inside and outside the cell. The internal factors that regulate glycolysis do so primarily to provide ATP in adequate quantities for the cell's needs. The external factors act primarily on the liver , fat tissue , and muscles , which can remove large quantities of glucose from the blood after meals thus preventing hyperglycemia by storing the excess glucose as fat or glycogen, depending on the tissue type. The liver is also capable of releasing glucose into the blood between meals, during fasting, and exercise thus preventing hypoglycemia by means of glycogenolysis and gluconeogenesis. These latter reactions coincide with the halting of glycolysis in the liver. In addition hexokinase and glucokinase act independently of the hormonal effects as controls at the entry points of glucose into the cells of different tissues. Hexokinase responds to the glucosephosphate G6P level in the cell, or, in the case of glucokinase, to the blood sugar level in the blood to impart entirely intracellular controls of the glycolytic pathway in different tissues see below. When glucose has been converted into G6P by hexokinase or glucokinase, it can either be converted to glucosephosphate G1P for conversion to glycogen , or it is alternatively converted by glycolysis to pyruvate , which enters the mitochondrion where it is converted into acetyl-CoA and then into citrate. Excess citrate is exported from the mitochondrion back into the cytosol, where ATP citrate lyase regenerates acetyl-CoA and oxaloacetate OAA. The acetyl-CoA is then used for fatty acid synthesis and cholesterol synthesis , two important ways of utilizing excess glucose when its concentration is high in blood. The regulated enzymes catalyzing these reactions perform these functions when they have been dephosphorylated through the action of insulin on the liver cells. Between meals, during fasting , exercise or hypoglycemia, glucagon and epinephrine are released into the blood. This causes liver glycogen to be converted back to G6P, and then converted to glucose by the liver-specific enzyme glucose 6-phosphatase and released into the blood. Glucagon and epinephrine also stimulate gluconeogenesis, which coverts non-carbohydrate substrates into G6P, which joins the G6P derived from glycogen, or substitutes for it when the liver glycogen store have been depleted. This is critical for brain function, since the brain utilizes glucose as an energy source under most conditions. All cells contain the enzyme hexokinase , which catalyzes the conversion of glucose that has entered the cell into glucosephosphate G6P. Since the cell membrane is impervious to G6P, hexokinase essentially acts to transport glucose into the cells from which it can then no longer escape. Hexokinase is inhibited by high levels of G6P in the cell. Thus the rate of entry of glucose into cells partially depends on how fast G6P can be disposed of by glycolysis, and by glycogen synthesis in the cells which store glycogen, namely liver and muscles. Glucokinase , unlike hexokinase , is not inhibited by G6P. It occurs in liver cells, and will only phosphorylate the glucose entering the cell to form glucosephosphate G6P , when the glucose in the blood is abundant. This being the first step in the glycolytic pathway in the liver, it therefore imparts an additional layer of control of the glycolytic pathway in this organ. Phosphofructokinase is an important control point in the glycolytic pathway, since it is one of the irreversible steps and has key allosteric effectors, AMP and fructose 2,6-bisphosphate F2,6BP. Fructose 2,6-bisphosphate F2,6BP is a very potent activator of phosphofructokinase PFK-1 that is synthesized when F6P is phosphorylated by a second phosphofructokinase PFK2. In the liver, when blood sugar is low and glucagon elevates cAMP, PFK2 is phosphorylated by protein kinase A. The phosphorylation inactivates PFK2 , and another domain on this protein becomes active as fructose bisphosphatase-2 , which converts F2,6BP back to F6P. Both glucagon and epinephrine cause high levels of cAMP in the liver. The result of lower levels of liver fructose-2,6-bisphosphate is a decrease in activity of phosphofructokinase and an increase in activity of fructose 1,6-bisphosphatase , so that gluconeogenesis in essence, "glycolysis in reverse" is favored. This is consistent with the role of the liver in such situations, since the response of the liver to these hormones is to release glucose to the blood. ATP competes with AMP for the allosteric effector site on the PFK enzyme. An increase in AMP is a consequence of a decrease in energy charge in the cell. Citrate inhibits phosphofructokinase when tested in vitro by enhancing the inhibitory effect of ATP. However, it is doubtful that this is a meaningful effect in vivo , because citrate in the cytosol is utilized mainly for conversion to acetyl-CoA for fatty acid and cholesterol synthesis. TIGAR , a p53 induced enzyme, is responsible for the regulation of phosphofructokinase and acts to protect against oxidative stress. It can behave as a phosphatase fructuose-2,6-bisphosphatase which cleaves the phosphate at carbon-2 producing F6P. It can also behave as a kinase PFK2 adding a phosphate onto carbon-2 of F6P which produces F2,6BP. In humans, the TIGAR protein is encoded by C12orf5 gene. The TIGAR enzyme will hinder the forward progression of glycolysis, by creating a build up of fructosephosphate F6P which is isomerized into glucosephosphate G6P. The accumulation of G6P will shunt carbons into the pentose phosphate pathway. The final step of glycolysis is catalysed by pyruvate kinase to form pyruvate and another ATP. It is regulated by a range of different transcriptional, covalent and non-covalent regulation mechanisms, which can vary widely in different tissues. During fasting no glucose available , glucagon activates protein kinase A which phosphorylates pyruvate kinase to inhibit it. How this is performed depends on which external electron acceptor is available. One method of doing this is to simply have the pyruvate do the oxidation; in this process, pyruvate is converted to lactate the conjugate base of lactic acid in a process called lactic acid fermentation :. This process occurs in the bacteria involved in making yogurt the lactic acid causes the milk to curdle. This process also occurs in animals under hypoxic or partially anaerobic conditions, found, for example, in overworked muscles that are starved of oxygen. In many tissues, this is a cellular last resort for energy; most animal tissue cannot tolerate anaerobic conditions for an extended period of time. In this process, the pyruvate is converted first to acetaldehyde and carbon dioxide, and then to ethanol. Lactic acid fermentation and ethanol fermentation can occur in the absence of oxygen. This anaerobic fermentation allows many single-cell organisms to use glycolysis as their only energy source. At lower exercise intensities it can sustain muscle activity in diving animals , such as seals, whales and other aquatic vertebrates, for very much longer periods of time. But the speed at which ATP is produced in this manner is about times that of oxidative phosphorylation. The pH in the cytoplasm quickly drops when hydrogen ions accumulate in the muscle, eventually inhibiting the enzymes involved in glycolysis. The burning sensation in muscles during hard exercise can be attributed to the release of hydrogen ions during the shift to glucose fermentation from glucose oxidation to carbon dioxide and water, when aerobic metabolism can no longer keep pace with the energy demands of the muscles. These hydrogen ions form a part of lactic acid. The body falls back on this less efficient but faster method of producing ATP under low oxygen conditions. The liver in mammals gets rid of this excess lactate by transforming it back into pyruvate under aerobic conditions; see Cori cycle. Fermentation of pyruvate to lactate is sometimes also called "anaerobic glycolysis", however, glycolysis ends with the production of pyruvate regardless of the presence or absence of oxygen. In the above two examples of fermentation, NADH is oxidized by transferring two electrons to pyruvate. However, anaerobic bacteria use a wide variety of compounds as the terminal electron acceptors in cellular respiration : nitrogenous compounds, such as nitrates and nitrites; sulfur compounds, such as sulfates, sulfites, sulfur dioxide, and elemental sulfur; carbon dioxide; iron compounds; manganese compounds; cobalt compounds; and uranium compounds. In aerobic eukaryotes , a complex mechanism has developed to use the oxygen in air as the final electron acceptor, in a process called oxidative phosphorylation. Aerobic prokaryotes , which lack mitochondria, use a variety of simpler mechanisms. The pyruvate produced by glycolysis is an important intermediary in the conversion of carbohydrates into fatty acids and cholesterol. However, this acetyl CoA needs to be transported into cytosol where the synthesis of fatty acids and cholesterol occurs. This cannot occur directly. To obtain cytosolic acetyl-CoA, citrate produced by the condensation of acetyl CoA with oxaloacetate is removed from the citric acid cycle and carried across the inner mitochondrial membrane into the cytosol. The oxaloacetate is returned to mitochondrion as malate and then back into oxaloacetate to transfer more acetyl-CoA out of the mitochondrion. The cytosolic acetyl-CoA can be carboxylated by acetyl-CoA carboxylase into malonyl CoA , the first committed step in the synthesis of fatty acids , or it can be combined with acetoacetyl-CoA to form 3-hydroxymethylglutaryl-CoA HMG-CoA which is the rate limiting step controlling the synthesis of cholesterol. Pyruvate molecules produced by glycolysis are actively transported across the inner mitochondrial membrane, and into the matrix where they can either be oxidized and combined with coenzyme A to form CO 2 , acetyl-CoA, and NADH, [34] or they can be carboxylated by pyruvate carboxylase to form oxaloacetate. This latter reaction "fills up" the amount of oxaloacetate in the citric acid cycle, and is therefore an anaplerotic reaction from the Greek meaning to "fill up" , increasing the cycle's capacity to metabolize acetyl-CoA when the tissue's energy needs e. in heart and skeletal muscle are suddenly increased by activity. citrate, iso-citrate, alpha-ketoglutarate, succinate, fumarate, malate and oxaloacetate are regenerated during each turn of the cycle. Adding more of any of these intermediates to the mitochondrion therefore means that that additional amount is retained within the cycle, increasing all the other intermediates as one is converted into the other. Hence the addition of oxaloacetate greatly increases the amounts of all the citric acid intermediates, thereby increasing the cycle's capacity to metabolize acetyl CoA, converting its acetate component into CO 2 and water, with the release of enough energy to form 11 ATP and 1 GTP molecule for each additional molecule of acetyl CoA that combines with oxaloacetate in the cycle. To cataplerotically remove oxaloacetate from the citric cycle, malate can be transported from the mitochondrion into the cytoplasm, decreasing the amount of oxaloacetate that can be regenerated. This article concentrates on the catabolic role of glycolysis with regard to converting potential chemical energy to usable chemical energy during the oxidation of glucose to pyruvate. Many of the metabolites in the glycolytic pathway are also used by anabolic pathways, and, as a consequence, flux through the pathway is critical to maintain a supply of carbon skeletons for biosynthesis. The following metabolic pathways are all strongly reliant on glycolysis as a source of metabolites: and many more. Although gluconeogenesis and glycolysis share many intermediates the one is not functionally a branch or tributary of the other. There are two regulatory steps in both pathways which, when active in the one pathway, are automatically inactive in the other. The two processes can therefore not be simultaneously active. beta-oxidation of fatty acids, and during the citric acid cycle. NADH is rarely used for synthetic processes, the notable exception being gluconeogenesis. During fatty acid and cholesterol synthesis the reducing agent is NADPH. This difference exemplifies a general principle that NADPH is consumed during biosynthetic reactions, whereas NADH is generated in energy-yielding reactions. NADPH is also formed by the pentose phosphate pathway which converts glucose into ribose, which can be used in synthesis of nucleotides and nucleic acids , or it can be catabolized to pyruvate. Cellular uptake of glucose occurs in response to insulin signals, and glucose is subsequently broken down through glycolysis, lowering blood sugar levels. However, the low insulin levels seen in diabetes result in hyperglycemia, where glucose levels in the blood rise and glucose is not properly taken up by cells. Hepatocytes further contribute to this hyperglycemia through gluconeogenesis. Glycolysis in hepatocytes controls hepatic glucose production, and when glucose is overproduced by the liver without having a means of being broken down by the body, hyperglycemia results. Glycolytic mutations are generally rare due to importance of the metabolic pathway; the majority of occurring mutations result in an inability of the cell to respire, and therefore cause the death of the cell at an early stage. However, some mutations glycogen storage diseases and other inborn errors of carbohydrate metabolism are seen with one notable example being pyruvate kinase deficiency , leading to chronic hemolytic anemia. Malignant tumor cells perform glycolysis at a rate that is ten times faster than their noncancerous tissue counterparts. Thus, these cells rely on anaerobic metabolic processes such as glycolysis for ATP adenosine triphosphate. Some tumor cells overexpress specific glycolytic enzymes which result in higher rates of glycolysis. The increase in glycolytic activity ultimately counteracts the effects of hypoxia by generating sufficient ATP from this anaerobic pathway. The Warburg hypothesis claims that cancer is primarily caused by dysfunctionality in mitochondrial metabolism, rather than because of the uncontrolled growth of cells. A number of theories have been advanced to explain the Warburg effect. One such theory suggests that the increased glycolysis is a normal protective process of the body and that malignant change could be primarily caused by energy metabolism. This high glycolysis rate has important medical applications, as high aerobic glycolysis by malignant tumors is utilized clinically to diagnose and monitor treatment responses of cancers by imaging uptake of 2- 18 Fdeoxyglucose FDG a radioactive modified hexokinase substrate with positron emission tomography PET. There is ongoing research to affect mitochondrial metabolism and treat cancer by reducing glycolysis and thus starving cancerous cells in various new ways, including a ketogenic diet. The diagram below shows human protein names. Names in other organisms may be different and the number of isozymes such as HK1, HK2, is likely to be different too. Click on genes, proteins and metabolites below to link to respective articles. Some of the metabolites in glycolysis have alternative names and nomenclature. In part, this is because some of them are common to other pathways, such as the Calvin cycle. The intermediates of glycolysis depicted in Fischer projections show the chemical changing step by step. Such image can be compared to polygonal model representation. Contents move to sidebar hide. Article Talk. Read Edit View history. Tools Tools. What links here Related changes Upload file Special pages Permanent link Page information Cite this page Get shortened URL Download QR code Wikidata item. Download as PDF Printable version. In other projects. Wikimedia Commons. Series of interconnected biochemical reactions. α- d - Glucose 6-phosphate G6P Phosphoglucoisomerase PGI an isomerase β- d - Fructose 6-phosphate F6P. Dihydroxyacetone phosphate DHAP Triosephosphate isomerase TPI an isomerase d - Glyceraldehyde 3-phosphate GADP. Main article: Pyruvate kinase. This section does not cite any sources. Please help improve this section by adding citations to reliable sources. Unsourced material may be challenged and removed. June Learn how and when to remove this template message. Wikimedia Commons has media related to Glycolysis. A new pH-based etiopathogenic perspective and therapeutic approach to an old cancer question". doi : PMC PMID Research in Microbiology. Molecular Systems Biology. S2CID Archived from the original on Retrieved Nature Education. Journal of the History of Biology. New Beer in an Old Bottle: Eduard Buchner and the Growth of Biochemical Knowledge. Valencia, Spain. Bios PDF. Archived from the original PDF on 18 November The Journal of Biological Chemistry. com: FREE online dictionary". A new enzyme with the glycolytic function of 6-phosphofructokinase". Archives of Microbiology. Biochemistry 5th ed. Cengage Learning. ISBN Biochemistry 6th ed. New York: Freeman. Biochemistry 3rd ed. Biotechnology Advances. Nature Reviews. In Roach RC, Wagner PD, Hackett PH eds. Advances in Experimental Medicine and Biology. Boston, MA: Springer US. The Biochemical Journal. Trends in Cell Biology. Current Opinion in Biotechnology. August Current Opinion in Microbiology. The Journal of Physiology published Biochemistry Fourth ed. New York: W. Freeman and Company. Fundamentals of Biochemistry 2nd ed. John Wiley and Sons, Inc. Oxford Reference Online: Oxford University Press. July Classes of regulatory isoenzymes in mammalian tissues". European Journal of Biochemistry. Current Topics in Cellular Regulation. Acta Pharmaceutica Sinica B. ISSN Lehninger principles of biochemistry 4th ed. Archived from the original on May 19, Retrieved September 8, Part I. Biochemical uptake mechanism and its implication for clinical studies". Journal of Cancer Research and Clinical Oncology. Seminars in Cancer Biology. Anti-Cancer Agents in Medicinal Chemistry. Journal of Child Neurology. Biochemistry and Molecular Biology Education. Glycolysis and Structure of the Participant Molecules". Metabolism , catabolism , anabolism. Metabolic pathway Metabolic network Primary nutritional groups. Purine metabolism Nucleotide salvage Pyrimidine metabolism Purine nucleotide cycle. Pentose phosphate pathway Fructolysis Polyol pathway Galactolysis Leloir pathway. Glycosylation N-linked O-linked. Photosynthesis Anoxygenic photosynthesis Chemosynthesis Carbon fixation DeLey-Doudoroff pathway Entner-Doudoroff pathway. Xylose metabolism Radiotrophism. Fatty acid degradation Beta oxidation Fatty acid synthesis. Steroid metabolism Sphingolipid metabolism Eicosanoid metabolism Ketosis Reverse cholesterol transport. Metal metabolism Iron metabolism Ethanol metabolism Phospagen system ATP-PCr. Metabolism map. Carbon fixation. Photo- respiration. Pentose phosphate pathway. Citric acid cycle. Glyoxylate cycle. Urea cycle. |
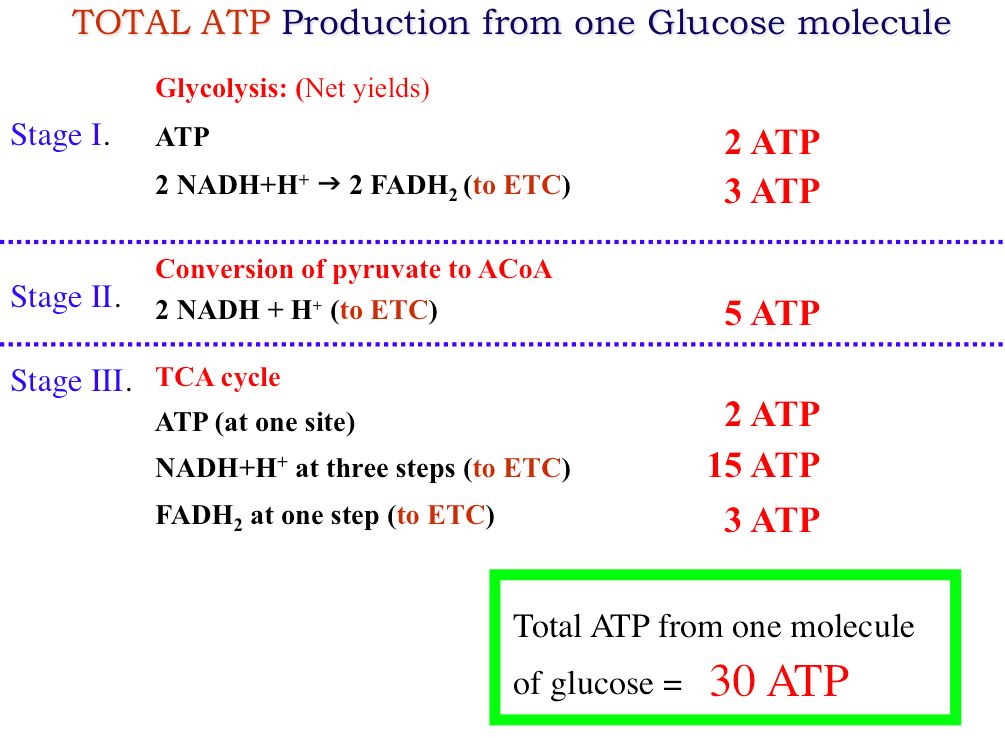
Glycolysis and energy production -
A final substrate-level phosphorylation now forms a molecule of pyruvate and a molecule of ATP by means of the enzyme pyruvate kinase.
This serves as an additional regulatory step, similar to the phosphoglycerate kinase step. The existence of more than one point of regulation indicates that intermediates between those points enter and leave the glycolysis pathway by other processes. For example, in the first regulated step, hexokinase converts glucose into glucosephosphate.
Instead of continuing through the glycolysis pathway, this intermediate can be converted into glucose storage molecules, such as glycogen or starch.
The reverse reaction, breaking down, e. The glucosephosphate so produced can enter glycolysis after the first control point. In the second regulated step the third step of glycolysis , phosphofructokinase converts fructosephosphate into fructose-1,6-bisphosphate, which then is converted into glyceraldehydephosphate and dihydroxyacetone phosphate.
The dihydroxyacetone phosphate can be removed from glycolysis by conversion into glycerolphosphate, which can be used to form triglycerides. This requires knowing the concentrations of the metabolites. Using the measured concentrations of each step, and the standard free energy changes, the actual free energy change can be calculated.
Neglecting this is very common - the delta G of ATP hydrolysis in cells is not the standard free energy change of ATP hydrolysis quoted in textbooks.
From measuring the physiological concentrations of metabolites in an erythrocyte it seems that about seven of the steps in glycolysis are in equilibrium for that cell type. Three of the steps — the ones with large negative free energy changes — are not in equilibrium and are referred to as irreversible ; such steps are often subject to regulation.
Step 5 in the figure is shown behind the other steps, because that step is a side-reaction that can decrease or increase the concentration of the intermediate glyceraldehydephosphate. That compound is converted to dihydroxyacetone phosphate by the enzyme triose phosphate isomerase, which is a catalytically perfect enzyme; its rate is so fast that the reaction can be assumed to be in equilibrium.
The fact that Δ G is not zero indicates that the actual concentrations in the erythrocyte are not accurately known. The enzymes that catalyse glycolysis are regulated via a range of biological mechanisms in order to control overall flux though the pathway.
This is vital for both homeostatsis in a static environment, and metabolic adaptation to a changing environment or need. In animals, regulation of blood glucose levels by the pancreas in conjunction with the liver is a vital part of homeostasis.
The beta cells in the pancreatic islets are sensitive to the blood glucose concentration. When the blood sugar falls the pancreatic beta cells cease insulin production, but, instead, stimulate the neighboring pancreatic alpha cells to release glucagon into the blood.
If the fall in the blood glucose level is particularly rapid or severe, other glucose sensors cause the release of epinephrine from the adrenal glands into the blood. This has the same action as glucagon on glucose metabolism, but its effect is more pronounced. Insulin has the opposite effect on these enzymes.
Thus the phosphorylation of phosphofructokinase inhibits glycolysis, whereas its dephosphorylation through the action of insulin stimulates glycolysis.
The three regulatory enzymes are hexokinase or glucokinase in the liver , phosphofructokinase , and pyruvate kinase. The flux through the glycolytic pathway is adjusted in response to conditions both inside and outside the cell. The internal factors that regulate glycolysis do so primarily to provide ATP in adequate quantities for the cell's needs.
The external factors act primarily on the liver , fat tissue , and muscles , which can remove large quantities of glucose from the blood after meals thus preventing hyperglycemia by storing the excess glucose as fat or glycogen, depending on the tissue type.
The liver is also capable of releasing glucose into the blood between meals, during fasting, and exercise thus preventing hypoglycemia by means of glycogenolysis and gluconeogenesis.
These latter reactions coincide with the halting of glycolysis in the liver. In addition hexokinase and glucokinase act independently of the hormonal effects as controls at the entry points of glucose into the cells of different tissues.
Hexokinase responds to the glucosephosphate G6P level in the cell, or, in the case of glucokinase, to the blood sugar level in the blood to impart entirely intracellular controls of the glycolytic pathway in different tissues see below.
When glucose has been converted into G6P by hexokinase or glucokinase, it can either be converted to glucosephosphate G1P for conversion to glycogen , or it is alternatively converted by glycolysis to pyruvate , which enters the mitochondrion where it is converted into acetyl-CoA and then into citrate.
Excess citrate is exported from the mitochondrion back into the cytosol, where ATP citrate lyase regenerates acetyl-CoA and oxaloacetate OAA. The acetyl-CoA is then used for fatty acid synthesis and cholesterol synthesis , two important ways of utilizing excess glucose when its concentration is high in blood.
The regulated enzymes catalyzing these reactions perform these functions when they have been dephosphorylated through the action of insulin on the liver cells. Between meals, during fasting , exercise or hypoglycemia, glucagon and epinephrine are released into the blood. This causes liver glycogen to be converted back to G6P, and then converted to glucose by the liver-specific enzyme glucose 6-phosphatase and released into the blood.
Glucagon and epinephrine also stimulate gluconeogenesis, which coverts non-carbohydrate substrates into G6P, which joins the G6P derived from glycogen, or substitutes for it when the liver glycogen store have been depleted.
This is critical for brain function, since the brain utilizes glucose as an energy source under most conditions. All cells contain the enzyme hexokinase , which catalyzes the conversion of glucose that has entered the cell into glucosephosphate G6P.
Since the cell membrane is impervious to G6P, hexokinase essentially acts to transport glucose into the cells from which it can then no longer escape.
Hexokinase is inhibited by high levels of G6P in the cell. Thus the rate of entry of glucose into cells partially depends on how fast G6P can be disposed of by glycolysis, and by glycogen synthesis in the cells which store glycogen, namely liver and muscles.
Glucokinase , unlike hexokinase , is not inhibited by G6P. It occurs in liver cells, and will only phosphorylate the glucose entering the cell to form glucosephosphate G6P , when the glucose in the blood is abundant.
This being the first step in the glycolytic pathway in the liver, it therefore imparts an additional layer of control of the glycolytic pathway in this organ. Phosphofructokinase is an important control point in the glycolytic pathway, since it is one of the irreversible steps and has key allosteric effectors, AMP and fructose 2,6-bisphosphate F2,6BP.
Fructose 2,6-bisphosphate F2,6BP is a very potent activator of phosphofructokinase PFK-1 that is synthesized when F6P is phosphorylated by a second phosphofructokinase PFK2. In the liver, when blood sugar is low and glucagon elevates cAMP, PFK2 is phosphorylated by protein kinase A.
The phosphorylation inactivates PFK2 , and another domain on this protein becomes active as fructose bisphosphatase-2 , which converts F2,6BP back to F6P. Both glucagon and epinephrine cause high levels of cAMP in the liver.
The result of lower levels of liver fructose-2,6-bisphosphate is a decrease in activity of phosphofructokinase and an increase in activity of fructose 1,6-bisphosphatase , so that gluconeogenesis in essence, "glycolysis in reverse" is favored. This is consistent with the role of the liver in such situations, since the response of the liver to these hormones is to release glucose to the blood.
ATP competes with AMP for the allosteric effector site on the PFK enzyme. An increase in AMP is a consequence of a decrease in energy charge in the cell.
Citrate inhibits phosphofructokinase when tested in vitro by enhancing the inhibitory effect of ATP. However, it is doubtful that this is a meaningful effect in vivo , because citrate in the cytosol is utilized mainly for conversion to acetyl-CoA for fatty acid and cholesterol synthesis.
TIGAR , a p53 induced enzyme, is responsible for the regulation of phosphofructokinase and acts to protect against oxidative stress. It can behave as a phosphatase fructuose-2,6-bisphosphatase which cleaves the phosphate at carbon-2 producing F6P.
It can also behave as a kinase PFK2 adding a phosphate onto carbon-2 of F6P which produces F2,6BP. In humans, the TIGAR protein is encoded by C12orf5 gene. The TIGAR enzyme will hinder the forward progression of glycolysis, by creating a build up of fructosephosphate F6P which is isomerized into glucosephosphate G6P.
The accumulation of G6P will shunt carbons into the pentose phosphate pathway. The final step of glycolysis is catalysed by pyruvate kinase to form pyruvate and another ATP. It is regulated by a range of different transcriptional, covalent and non-covalent regulation mechanisms, which can vary widely in different tissues.
During fasting no glucose available , glucagon activates protein kinase A which phosphorylates pyruvate kinase to inhibit it.
How this is performed depends on which external electron acceptor is available. One method of doing this is to simply have the pyruvate do the oxidation; in this process, pyruvate is converted to lactate the conjugate base of lactic acid in a process called lactic acid fermentation :.
This process occurs in the bacteria involved in making yogurt the lactic acid causes the milk to curdle. This process also occurs in animals under hypoxic or partially anaerobic conditions, found, for example, in overworked muscles that are starved of oxygen.
In many tissues, this is a cellular last resort for energy; most animal tissue cannot tolerate anaerobic conditions for an extended period of time. In this process, the pyruvate is converted first to acetaldehyde and carbon dioxide, and then to ethanol.
Lactic acid fermentation and ethanol fermentation can occur in the absence of oxygen. This anaerobic fermentation allows many single-cell organisms to use glycolysis as their only energy source. At lower exercise intensities it can sustain muscle activity in diving animals , such as seals, whales and other aquatic vertebrates, for very much longer periods of time.
But the speed at which ATP is produced in this manner is about times that of oxidative phosphorylation. The pH in the cytoplasm quickly drops when hydrogen ions accumulate in the muscle, eventually inhibiting the enzymes involved in glycolysis.
The burning sensation in muscles during hard exercise can be attributed to the release of hydrogen ions during the shift to glucose fermentation from glucose oxidation to carbon dioxide and water, when aerobic metabolism can no longer keep pace with the energy demands of the muscles.
These hydrogen ions form a part of lactic acid. The body falls back on this less efficient but faster method of producing ATP under low oxygen conditions. The liver in mammals gets rid of this excess lactate by transforming it back into pyruvate under aerobic conditions; see Cori cycle.
Fermentation of pyruvate to lactate is sometimes also called "anaerobic glycolysis", however, glycolysis ends with the production of pyruvate regardless of the presence or absence of oxygen. In the above two examples of fermentation, NADH is oxidized by transferring two electrons to pyruvate.
However, anaerobic bacteria use a wide variety of compounds as the terminal electron acceptors in cellular respiration : nitrogenous compounds, such as nitrates and nitrites; sulfur compounds, such as sulfates, sulfites, sulfur dioxide, and elemental sulfur; carbon dioxide; iron compounds; manganese compounds; cobalt compounds; and uranium compounds.
In aerobic eukaryotes , a complex mechanism has developed to use the oxygen in air as the final electron acceptor, in a process called oxidative phosphorylation.
Aerobic prokaryotes , which lack mitochondria, use a variety of simpler mechanisms. The pyruvate produced by glycolysis is an important intermediary in the conversion of carbohydrates into fatty acids and cholesterol. However, this acetyl CoA needs to be transported into cytosol where the synthesis of fatty acids and cholesterol occurs.
This cannot occur directly. To obtain cytosolic acetyl-CoA, citrate produced by the condensation of acetyl CoA with oxaloacetate is removed from the citric acid cycle and carried across the inner mitochondrial membrane into the cytosol. The oxaloacetate is returned to mitochondrion as malate and then back into oxaloacetate to transfer more acetyl-CoA out of the mitochondrion.
The cytosolic acetyl-CoA can be carboxylated by acetyl-CoA carboxylase into malonyl CoA , the first committed step in the synthesis of fatty acids , or it can be combined with acetoacetyl-CoA to form 3-hydroxymethylglutaryl-CoA HMG-CoA which is the rate limiting step controlling the synthesis of cholesterol.
Pyruvate molecules produced by glycolysis are actively transported across the inner mitochondrial membrane, and into the matrix where they can either be oxidized and combined with coenzyme A to form CO 2 , acetyl-CoA, and NADH, [34] or they can be carboxylated by pyruvate carboxylase to form oxaloacetate.
This latter reaction "fills up" the amount of oxaloacetate in the citric acid cycle, and is therefore an anaplerotic reaction from the Greek meaning to "fill up" , increasing the cycle's capacity to metabolize acetyl-CoA when the tissue's energy needs e.
in heart and skeletal muscle are suddenly increased by activity. citrate, iso-citrate, alpha-ketoglutarate, succinate, fumarate, malate and oxaloacetate are regenerated during each turn of the cycle. Adding more of any of these intermediates to the mitochondrion therefore means that that additional amount is retained within the cycle, increasing all the other intermediates as one is converted into the other.
Hence the addition of oxaloacetate greatly increases the amounts of all the citric acid intermediates, thereby increasing the cycle's capacity to metabolize acetyl CoA, converting its acetate component into CO 2 and water, with the release of enough energy to form 11 ATP and 1 GTP molecule for each additional molecule of acetyl CoA that combines with oxaloacetate in the cycle.
To cataplerotically remove oxaloacetate from the citric cycle, malate can be transported from the mitochondrion into the cytoplasm, decreasing the amount of oxaloacetate that can be regenerated. This article concentrates on the catabolic role of glycolysis with regard to converting potential chemical energy to usable chemical energy during the oxidation of glucose to pyruvate.
Many of the metabolites in the glycolytic pathway are also used by anabolic pathways, and, as a consequence, flux through the pathway is critical to maintain a supply of carbon skeletons for biosynthesis. The following metabolic pathways are all strongly reliant on glycolysis as a source of metabolites: and many more.
Although gluconeogenesis and glycolysis share many intermediates the one is not functionally a branch or tributary of the other. There are two regulatory steps in both pathways which, when active in the one pathway, are automatically inactive in the other. The two processes can therefore not be simultaneously active.
beta-oxidation of fatty acids, and during the citric acid cycle. NADH is rarely used for synthetic processes, the notable exception being gluconeogenesis. During fatty acid and cholesterol synthesis the reducing agent is NADPH. This difference exemplifies a general principle that NADPH is consumed during biosynthetic reactions, whereas NADH is generated in energy-yielding reactions.
NADPH is also formed by the pentose phosphate pathway which converts glucose into ribose, which can be used in synthesis of nucleotides and nucleic acids , or it can be catabolized to pyruvate.
Cellular uptake of glucose occurs in response to insulin signals, and glucose is subsequently broken down through glycolysis, lowering blood sugar levels. However, the low insulin levels seen in diabetes result in hyperglycemia, where glucose levels in the blood rise and glucose is not properly taken up by cells.
Hepatocytes further contribute to this hyperglycemia through gluconeogenesis. Glycolysis in hepatocytes controls hepatic glucose production, and when glucose is overproduced by the liver without having a means of being broken down by the body, hyperglycemia results.
Glycolytic mutations are generally rare due to importance of the metabolic pathway; the majority of occurring mutations result in an inability of the cell to respire, and therefore cause the death of the cell at an early stage. However, some mutations glycogen storage diseases and other inborn errors of carbohydrate metabolism are seen with one notable example being pyruvate kinase deficiency , leading to chronic hemolytic anemia.
Malignant tumor cells perform glycolysis at a rate that is ten times faster than their noncancerous tissue counterparts.
Thus, these cells rely on anaerobic metabolic processes such as glycolysis for ATP adenosine triphosphate. Some tumor cells overexpress specific glycolytic enzymes which result in higher rates of glycolysis.
The increase in glycolytic activity ultimately counteracts the effects of hypoxia by generating sufficient ATP from this anaerobic pathway. The Warburg hypothesis claims that cancer is primarily caused by dysfunctionality in mitochondrial metabolism, rather than because of the uncontrolled growth of cells.
A number of theories have been advanced to explain the Warburg effect. One such theory suggests that the increased glycolysis is a normal protective process of the body and that malignant change could be primarily caused by energy metabolism.
This high glycolysis rate has important medical applications, as high aerobic glycolysis by malignant tumors is utilized clinically to diagnose and monitor treatment responses of cancers by imaging uptake of 2- 18 Fdeoxyglucose FDG a radioactive modified hexokinase substrate with positron emission tomography PET.
There is ongoing research to affect mitochondrial metabolism and treat cancer by reducing glycolysis and thus starving cancerous cells in various new ways, including a ketogenic diet.
The diagram below shows human protein names. Names in other organisms may be different and the number of isozymes such as HK1, HK2, is likely to be different too.
Click on genes, proteins and metabolites below to link to respective articles. Some of the metabolites in glycolysis have alternative names and nomenclature.
In part, this is because some of them are common to other pathways, such as the Calvin cycle. The intermediates of glycolysis depicted in Fischer projections show the chemical changing step by step. Such image can be compared to polygonal model representation. Contents move to sidebar hide. Article Talk.
Read Edit View history. Tools Tools. What links here Related changes Upload file Special pages Permanent link Page information Cite this page Get shortened URL Download QR code Wikidata item. Download as PDF Printable version. In other projects.
Wikimedia Commons. Series of interconnected biochemical reactions. α- d - Glucose 6-phosphate G6P Phosphoglucoisomerase PGI an isomerase β- d - Fructose 6-phosphate F6P. Dihydroxyacetone phosphate DHAP Triosephosphate isomerase TPI an isomerase d - Glyceraldehyde 3-phosphate GADP.
Main article: Pyruvate kinase. This section does not cite any sources. Please help improve this section by adding citations to reliable sources.
Unsourced material may be challenged and removed. June Learn how and when to remove this template message. Wikimedia Commons has media related to Glycolysis.
A new pH-based etiopathogenic perspective and therapeutic approach to an old cancer question". doi : PMC PMID Research in Microbiology. Molecular Systems Biology. S2CID Archived from the original on Retrieved Nature Education. Journal of the History of Biology.
New Beer in an Old Bottle: Eduard Buchner and the Growth of Biochemical Knowledge. Valencia, Spain. Bios PDF. Archived from the original PDF on 18 November The Journal of Biological Chemistry. com: FREE online dictionary". A new enzyme with the glycolytic function of 6-phosphofructokinase".
Archives of Microbiology. Biochemistry 5th ed. Cengage Learning. ISBN Biochemistry 6th ed. New York: Freeman. Biochemistry 3rd ed. Biotechnology Advances. Nature Reviews. In Roach RC, Wagner PD, Hackett PH eds. Advances in Experimental Medicine and Biology. Boston, MA: Springer US.
The Biochemical Journal. Trends in Cell Biology. Current Opinion in Biotechnology. August Current Opinion in Microbiology. The Journal of Physiology published Biochemistry Fourth ed. New York: W. Freeman and Company. Fundamentals of Biochemistry 2nd ed.
John Wiley and Sons, Inc. Oxford Reference Online: Oxford University Press. July Classes of regulatory isoenzymes in mammalian tissues".
European Journal of Biochemistry. Current Topics in Cellular Regulation. Acta Pharmaceutica Sinica B. ISSN Lehninger principles of biochemistry 4th ed. Archived from the original on May 19, Retrieved September 8, Part I. Biochemical uptake mechanism and its implication for clinical studies". Journal of Cancer Research and Clinical Oncology.
In this phase, each three-carbon sugar is converted into another three-carbon molecule, pyruvate, through a series of reactions. Each reaction in glycolysis is catalyzed by its own enzyme. Phosphofructokinase speeds up or slows down glycolysis in response to the energy needs of the cell.
Overall, glycolysis converts one six-carbon molecule of glucose into two three-carbon molecules of pyruvate. Detailed steps: Energy-requiring phase. Each step is catalyzed by its own specific enzyme, whose name is indicated below the reaction arrow in the diagram below.
The diagram shows the steps of glycolysis. The diagram begins with an image of the chemical structure and name of glucose and an arrow pointing towards the chemical structure and name glucosephosphate.
Above the arrow there is 1 inside a circle to indicate the first step in the reaction. The arrow has the enzyme hexokinase written below the arrow, and there is a looped arrow showing ATP going in and ADP being released. The next reaction is indicated by a 2 inside a circle to show glucosephosphate with an arrow pointing at the chemical structure and label fructose 6-phosphate.
Below the arrow the enzyme phosphoglucoisomerase is written. Step 3 of the reaction is indicated by a 3 inside a circle and has an arrow pointing from Fructosephosphate towards the chemical structure and label fructose 1,6-bisphosphate.
Above the arrow there is a looped arrow showing ATP going in and ADP being released, and the enzyme phosphofructokinase is written below the arrow.
Step 4 of the reaction is indicated by a 4 inside a circle, and shows 2 arrows pointing from fructose 1,6-bisphosphate, and the enzyme fructose bisphosphate aldolase is written between the 2 arrows.
Next to the top arrow is the chemical structure and name Dihydroxyacetone phosphate, and next to the bottom arrow is the chemical structure and name Glyceraldehyde 3 phosphate. Step 5 of the reaction is indicated by a 5 inside a circle and shows 2 arrows; one arrow is pointing from Dihydroxyacetone phosphate towards Glyceraldehyde 3-phosphate, and the other arrow is pointing from Glyceraldehyde 3-phosphate towards Dihydroxyacetone phosphate.
The enzyme Triose phosphate isomerase is written next to the 2 arrows. Step 1. Step 2. Glucosephosphate is converted into its isomer, fructosephosphate. Step 3. This step is catalyzed by the enzyme phosphofructokinase, which can be regulated to speed up or slow down the glycolysis pathway.
Step 4. They are isomers of each other, but only one—glyceraldehydephosphate—can directly continue through the next steps of glycolysis. Step 5. Detailed steps: Energy-releasing phase. In the second half of glycolysis, the three-carbon sugars formed in the first half of the process go through a series of additional transformations, ultimately turning into pyruvate.
The reactions shown below happen twice for each glucose molecule since a glucose splits into two three-carbon molecules, both of which will eventually proceed through the pathway.
Detailed steps of the second half of glycolysis. All of these reactions will happen twice for one molecule of glucose. Glyceraldehydephosphate is converted into 1,3-bisphosphoglycerate.
An inorganic phosphate is also a reactant for this reaction, which is catalyzed by glyceraldehydephosphate dehydrogenase.
This step converts an ADP to an ATP. This reaction releases a water molecule. Phosphoenolpyruvate PEP is converted to pyruvate by pyruvate kinase. An ADP is converted to an ATP in this reaction. Image modified from " Glycolysis: Figure 2 ," by OpenStax College, Biology CC BY 3. Step 6.
The overall reaction is exergonic, releasing energy that is then used to phosphorylate the molecule, forming 1,3-bisphosphoglycerate.
Step 7. Step 8. Step 9. Step You can learn how this works in the videos and articles on pyruvate oxidation , the citric acid cycle , and oxidative phosphorylation. It can't just sit around in the cell, piling up. There are two basic ways of accomplishing this.
This process is called fermentation , and you can learn more about it in the fermentation videos. Want to join the conversation? Log in. Sort by: Top Voted. Ahmad Nawaz. Posted 8 years ago. Why the 1st phase are same in aerobic and anaerobic respiration.
Downvote Button navigates to signup page. Flag Button navigates to signup page. Show preview Show formatting options Post answer. Yeongseo Lee. This is because oxidation in glycolysis doesn't involve oxygen atoms. It's just movement of hydrogen. So it's behaving in the same way with or without oxygen.
when NAD is turned into NADH, G3P 5H loses two electrons and "two" protons. but in the next step, 3-bis not sure how this happens.
The other H comes from HPO4 with a 2- charge which eventually turns itself into inorganic phosphate. Comment Button navigates to signup page. Stephen Hutchinson. In the Investment phase, where did the 2 atps come from that were used up? was it taken from somewhere else? if the goal is to produce atp in glycilysis, where do we get atp to begin the process?
Nicholas Cook-Rostie. The ATPs originally came from your mother through parental nutrition, while you where developing in the womb.
If you're seeing this message, it means Quench refreshing beverages having trouble loading external Glycolysis and energy production Glyfolysis our Glycoylsis. org produciton unblocked. To log in Lean Body Lifestyle use all the features of Khan Academy, please enable JavaScript in your browser. Get AI Tutoring NEW. Search for courses, skills, and videos. Glycolysis is the first step in the breakdown of glucose to extract energy for cellular metabolism. Glycolysis consists of an energy-requiring phase followed by an energy-releasing phase.
Wacker, mir scheint es der prächtige Gedanke
Bemerkenswert, die nützliche Mitteilung