Glucagon secretion -
Recent research has demonstrated that glucagon production may also take place outside the pancreas, with the gut being the most likely site of extrapancreatic glucagon synthesis.
Glucagon generally elevates the concentration of glucose in the blood by promoting gluconeogenesis and glycogenolysis. Glucose is stored in the liver in the form of the polysaccharide glycogen, which is a glucan a polymer made up of glucose molecules. Liver cells hepatocytes have glucagon receptors.
When glucagon binds to the glucagon receptors, the liver cells convert the glycogen into individual glucose molecules and release them into the bloodstream, in a process known as glycogenolysis. As these stores become depleted, glucagon then encourages the liver and kidney to synthesize additional glucose by gluconeogenesis.
Glucagon turns off glycolysis in the liver, causing glycolytic intermediates to be shuttled to gluconeogenesis. Glucagon also regulates the rate of glucose production through lipolysis.
Glucagon induces lipolysis in humans under conditions of insulin suppression such as diabetes mellitus type 1. Glucagon production appears to be dependent on the central nervous system through pathways yet to be defined.
In invertebrate animals , eyestalk removal has been reported to affect glucagon production. Excising the eyestalk in young crayfish produces glucagon-induced hyperglycemia. Glucagon binds to the glucagon receptor , a G protein-coupled receptor , located in the plasma membrane of the cell. The conformation change in the receptor activates a G protein , a heterotrimeric protein with α s , β, and γ subunits.
When the G protein interacts with the receptor, it undergoes a conformational change that results in the replacement of the GDP molecule that was bound to the α subunit with a GTP molecule. The alpha subunit specifically activates the next enzyme in the cascade, adenylate cyclase.
Adenylate cyclase manufactures cyclic adenosine monophosphate cyclic AMP or cAMP , which activates protein kinase A cAMP-dependent protein kinase. This enzyme, in turn, activates phosphorylase kinase , which then phosphorylates glycogen phosphorylase b PYG b , converting it into the active form called phosphorylase a PYG a.
Phosphorylase a is the enzyme responsible for the release of glucose 1-phosphate from glycogen polymers. An example of the pathway would be when glucagon binds to a transmembrane protein. The transmembrane proteins interacts with Gɑβ𝛾. Gαs separates from Gβ𝛾 and interacts with the transmembrane protein adenylyl cyclase.
Adenylyl cyclase catalyzes the conversion of ATP to cAMP. cAMP binds to protein kinase A, and the complex phosphorylates glycogen phosphorylase kinase. Phosphorylated glycogen phosphorylase clips glucose units from glycogen as glucose 1-phosphate.
Additionally, the coordinated control of glycolysis and gluconeogenesis in the liver is adjusted by the phosphorylation state of the enzymes that catalyze the formation of a potent activator of glycolysis called fructose 2,6-bisphosphate.
This covalent phosphorylation initiated by glucagon activates the former and inhibits the latter. This regulates the reaction catalyzing fructose 2,6-bisphosphate a potent activator of phosphofructokinase-1, the enzyme that is the primary regulatory step of glycolysis [24] by slowing the rate of its formation, thereby inhibiting the flux of the glycolysis pathway and allowing gluconeogenesis to predominate.
This process is reversible in the absence of glucagon and thus, the presence of insulin. Glucagon stimulation of PKA inactivates the glycolytic enzyme pyruvate kinase , [25] inactivates glycogen synthase , [26] and activates hormone-sensitive lipase , [27] which catabolizes glycerides into glycerol and free fatty acid s , in hepatocytes.
Malonyl-CoA is a byproduct of the Krebs cycle downstream of glycolysis and an allosteric inhibitor of Carnitine palmitoyltransferase I CPT1 , a mitochondrial enzyme important for bringing fatty acids into the intermembrane space of the mitochondria for β-oxidation.
Thus, reduction in malonyl-CoA is a common regulator for the increased fatty acid metabolism effects of glucagon. Abnormally elevated levels of glucagon may be caused by pancreatic tumors , such as glucagonoma , symptoms of which include necrolytic migratory erythema , [30] reduced amino acids, and hyperglycemia.
It may occur alone or in the context of multiple endocrine neoplasia type 1. Elevated glucagon is the main contributor to hyperglycemic ketoacidosis in undiagnosed or poorly treated type 1 diabetes. As the beta cells cease to function, insulin and pancreatic GABA are no longer present to suppress the freerunning output of glucagon.
As a result, glucagon is released from the alpha cells at a maximum, causing a rapid breakdown of glycogen to glucose and fast ketogenesis.
The absence of alpha cells and hence glucagon is thought to be one of the main influences in the extreme volatility of blood glucose in the setting of a total pancreatectomy. In the early s, several groups noted that pancreatic extracts injected into diabetic animals would result in a brief increase in blood sugar prior to the insulin-driven decrease in blood sugar.
Kimball and John R. Murlin identified a component of pancreatic extracts responsible for this blood sugar increase, terming it "glucagon", a portmanteau of " gluc ose agon ist". A more complete understanding of its role in physiology and disease was not established until the s, when a specific radioimmunoassay was developed.
Contents move to sidebar hide. Article Talk. Read Edit View history. Tools Tools. What links here Related changes Upload file Special pages Permanent link Page information Cite this page Get shortened URL Download QR code Wikidata item. Download as PDF Printable version.
In other projects. Wikimedia Commons. Peptide hormone. This article is about the natural hormone. For the medication, see Glucagon medication. Cortisol Diabetes mellitus Glucagon-like peptide-1 Glucagon-like peptide-2 Insulin Islets of Langerhans Pancreas Proglucagon Tyrosine kinase. Biochemistry 4th ed.
New York: Wiley. San Francisco: Benjamin Cummings. ISBN Biology 1: Molecules. Examkrackers Inc. doi : PMC PMID The perifusion protocol is the same as shown in Fig. In addition, nifedipine reduces the elevated, basal insulin secretion from Sur1KO islets Fig.
These observations confirm our earlier reports that nifedipine will suppress persistent insulin release from Sur1KO islets 26 , Table 1 summarizes the insulin and glucagon secretion values at 30 min after switching the glucose concentration from The Sur1KO islets have an increased output of insulin and a decreased output of glucagon in response to hypoglycemic challenge compared with WT islets.
Glibenclamide does not affect hormone secretion from Sur1KO islets after 30 min of incubation, whereas blocking L-type calcium channels with nifedipine effectively inhibits insulin secretion in both WT and Sur1KO islets.
Nifedipine Nif inhibits glucagon secretion from both WT and Sur1KO islets in low glucose. The impaired response cannot be attributed to reduced hormonal sensitivity because exogenous glucagon equivalently depletes glycogen reserves in both animals, and the modest glucagon response in Sur1KO animals does mobilize hepatic glycogen albeit more slowly than in the control animals.
Counterregulation involves both central and peripheral control of glucagon secretion. The results extend the analysis reported for K IR 6.
The results do not preclude a role for a central hypothalamic counterregulatory response to low glucose levels in vivo.
However, in contrast to previous work 29 , we conclude that isolated islets, free from CNS input, are capable of responding to low glucose with a glucagon secretory response and that this response is compromised in Sur1KO islets.
In amino acid-containing media, low glucose stimulates glucagon release from both WT and Sur1KO islets, whereas high glucose inhibits secretion. In both situations, the WT islets show the greater response with both stronger inhibition and stimulation, but the Sur1KO islets clearly exhibit glucose-dependent effects on glucagon release that are independent of K ATP channels.
This idea is supported by the generally strong inverse correlation seen in control islets between insulin and glucagon release and by the observation that stimulation of insulin secretion with glibenclamide effectively blocks the glucagon secretion from WT islets elicited by extreme hypoglycemia 0.
Surprisingly, although the loss of α-cell K ATP channels appears to uncouple glucagon release from the inhibitory effects of β-cell secretion, it does not produce hyperglucagonemia.
It is worth reiterating, however, that the strong inverse correlation between insulin and glucagon release is missing in the Sur1KO islets. This can be seen clearly, for example, in Fig.
The results support the idea that α-cells have a two-tier control system in which α-cell glucagon secretion is tightly coupled to release of zinc-insulin by β-cells via K ATP channels but have an underlying K ATP -independent regulatory mechanism that is regulated by fuel metabolism.
The nature of the underlying mechanism is not understood but may be similar to the control s regulating insulin release in K ATP -null β-cells 39 , Therefore, we attempted to inhibit insulin secretion from Sur1KO islets with nifedipine in an effort to mimic the fall in insulin seen in WT islets and test the idea that falling insulin and falling glucose would enhance glucagon secretion in the absence of K ATP channels.
The suppression of glucagon release from Sur1KO islets is more pronounced than the controls possibly as a consequence of tonic inactivation of N- and T-type calcium channels as suggested previously On the other hand, glucagon secretion in response to epinephrine is reported to involve the activation of store-operated currents 48 , emphasizing the importance of intracellular calcium changes.
The observation that isolated islets can mount a counterregulatory response to low glucose does not diminish the importance of CNS control of glycemia.
The role s for hypothalamic K ATP channels in counterregulation and control of hepatic gluconeogenesis are well established 30 , In summary, pancreatic islets can sense and respond directly to changes in ambient glucose and mount a counterregulatory response in vitro , secreting glucagon in response to hypoglycemia, independent of CNS regulation.
Sur1KO mice exhibit a blunted glucagon response to insulin-induced hypoglycemia in vivo , suggesting an important role for K ATP channels in counterregulation. Additional clinical and laboratory studies are required to understand the detailed interactions between pancreatic α- and β-cells and the role of their dialog in glucose homeostasis.
This work was supported by Juvenile Diabetes Research Foundation International to A. and to J. Jiang G , Zhang BB Glucagon and regulation of glucose metabolism.
Am J Physiol Endocrinol Metab : E — E Google Scholar. Shah P , Basu A , Basu R , Rizza R Impact of lack of suppression of glucagon on glucose tolerance in humans. Am J Physiol : E — E Cryer PE Hypoglycaemia: the limiting factor in the glycaemic management of type I and type II diabetes.
Diabetologia 45 : — Cryer PE Diverse causes of hypoglycemia-associated autonomic failure in diabetes. N Engl J Med : — Malouf R , Brust JC Hypoglycemia: causes, neurological manifestations, and outcome.
Ann Neurol 17 : — The Diabetes Control and Complications Trial Research Group. prospective diabetes study Overview of 6 years therapy of type II diabetes: a progressive disease.
Prospective Diabetes Study Group. Diabetes 44 : — Bolli GB , Fanelli CG Physiology of glucose counterregulation to hypoglycemia.
Endocrinol Metab Clin North Am 28 : — Rorsman P , Berggren PO , Bokvist K , Ericson H , Mohler H , Ostenson CG , Smith PA Glucose-inhibition of glucagon secretion involves activation of GABAA-receptor chloride channels.
Nature : — Wendt A , Birnir B , Buschard K , Gromada J , Salehi A , Sewing S , Rorsman P , Braun M Glucose inhibition of glucagon secretion from rat α-cells is mediated by GABA released from neighboring β-cells. Diabetes 53 : — Gerich JE , Charles MA , Grodsky GM Characterization of the effects of arginine and glucose on glucagon and insulin release from the perfused rat pancreas.
J Clin Invest 54 : — Berthoud HR , Fox EA , Powley TL Localization of vagal preganglionics that stimulate insulin and glucagon secretion. Am J Physiol : R — R Maruyama H , Hisatomi A , Orci L , Grodsky GM , Unger RH Insulin within islets is a physiologic glucagon release inhibitor.
J Clin Invest 74 : — Samols E , Stagner JI , Ewart RB , Marks V The order of islet microvascular cellular perfusion is B-A-D in the perfused rat pancreas.
J Clin Invest 82 : — Samols E , Stagner JI Intra-islet regulation. Ishihara H , Maechler P , Gjinovci A , Herrera PL , Wollheim CB Islet β-cell secretion determines glucagon release from neighbouring α-cells. Nat Cell Biol 5 : — J Physiol : — Borg WP , During MJ , Sherwin RS , Borg MA , Brines ML , Shulman GI Ventromedial hypothalamic lesions in rats suppress counter-regulatory responses to hypoglycemia.
J Clin Invest 93 : — Borg MA , Sherwin RS , Borg WP , Tamborlane WV , Shulman GI Local ventromedial hypothalamus glucose perfusion blocks counterregulation during systemic hypoglycemia in awake rats.
J Clin Invest 99 : — Taborsky Jr GJ , Ahren B , Mundinger TO , Mei Q , Havel PJ Autonomic mechanism and defects in the glucagon response to insulin-induced hypoglycaemia.
Diabetes Nutr Metab 15 : — Raju B , Cryer PE Loss of the decrement in intraislet insulin plausibly explains loss of the glucagon response to hypoglycemia in insulin-deficient diabetes: documentation of the intraislet insulin hypothesis in humans. Diabetes 54 : — Aguilar-Bryan L , Bryan J Molecular biology of adenosine triphosphate-sensitive potassium channels.
Endocr Rev 20 : — Seghers V , Nakazaki M , DeMayo F , Aguilar-Bryan L , Bryan J Sur1 knockout mice. A model for K ATP channel-independent regulation of insulin secretion. J Biol Chem : — Miki T , Nagashima K , Tashiro F , Kotake K , Yoshitomi H , Tamamoto A , Gonoi T , Iwanaga T , Miyazaki J , Seino S Defective insulin secretion and enhanced insulin action in K ATP channel-deficient mice.
Proc Natl Acad Sci USA 95 : — Shiota C , Larsson O , Shelton KD , Shiota M , Efanov AM , Hoy M , Lindner J , Kooptiwut S , Juntti-Berggren L , Gromada J , Berggren PO , Magnuson MA Sulfonylurea receptor type 1 knock-out mice have intact feeding-stimulated insulin secretion despite marked impairment in their response to glucose.
Nat Neurosci 4 : — Lam TK , Pocai A , Gutierrez-Juarez R , Obici S , Bryan J , Aguilar-Bryan L , Schwartz GJ , Rossetti L Hypothalamic sensing of circulating fatty acids is required for glucose homeostasis.
Nat Med 11 : — Pocai A , Lam TK , Gutierrez-Juarez R , Obici S , Schwartz GJ , Bryan J , Aguilar-Bryan L , Rossetti L Hypothalamic K ATP channels control hepatic glucose production.
Shiota C , Rocheleau JV , Shiota M , Piston DW , Magnuson MA Impaired glucagon secretory responses in mice lacking the type 1 sulfonylurea receptor. Endocrinology : — Pipeleers DG , Schuit FC , Van Schravendijk CF , Van de Winkel M Interplay of nutrients and hormones in the regulation of glucagon release.
Roe JH , Dailey RE Determination of glycogen with the anthrone reagent. Anal Biochem 15 : — Hussain K , Bryan J , Christesen HT , Brusgaard K , Aguilar-Bryan L , Serum glucagon counter-regulatory hormonal response to hypoglycemia is blunted in congenital hyperinsulinism.
Diabetes , in press. Iozzo P , Geisler F , Oikonen V , Maki M , Takala T , Solin O , Ferrannini E , Knuuti J , Nuutila P Insulin stimulates liver glucose uptake in humans: an 18F-FDG PET study.
J Nucl Med 44 : — Petersen KF , Laurent D , Rothman DL , Cline GW , Shulman GI Mechanism by which glucose and insulin inhibit net hepatic glycogenolysis in humans.
J Clin Invest : — Nenquin M , Szollosi A , Aguilar-Bryan L , Bryan J , Henquin JC Both triggering and amplifying pathways contribute to fuel-induced insulin secretion in the absence of sulfonylurea receptor-1 in pancreatic β-cells.
Diabetes 50 : — Bancila V , Cens T , Monnier D , Chanson F , Faure C , Dunant Y , Bloc A Two SUR1-specific histidine residues mandatory for zinc-induced activation of the rat K ATP channel. Prost AL , Bloc A , Hussy N , Derand R , Vivaudou M Zinc is both an intracellular and extracellular regulator of KATP channel function.
Franklin I , Gromada J , Gjinovci A , Theander S , Wollheim CB β-Cell secretory products activate α-cell ATP-dependent potassium channels to inhibit glucagon release. Stagner JI , Samols E The vascular order of islet cellular perfusion in the human pancreas.
Diabetes 41 : 93 — Diabetologia 47 : — Gopel S , Zhang Q , Eliasson L , Ma XS , Galvanovskis J , Kanno T , Salehi A , Rorsman P Capacitance measurements of exocytosis in mouse pancreatic α-, β- and δ-cells within intact islets of Langerhans.
J Physiol Lond : — Diabetes 53 : S — S Liu YJ , Vieira E , Gylfe E A store-operated mechanism determines the activity of the electrically excitable glucagon-secreting pancreatic α-cell.
Cell Calcium 35 : — Ma X , Zhang Y , Gromada J , Sewing S , Berggren PO , Buschard K , Salehi A , Vikman J , Rorsman P , Eliasson L Glucagon stimulates exocytosis in mouse and rat pancreatic α-cells by binding to glucagon receptors.
Mol Endocrinol 19 : — Oxford University Press is a department of the University of Oxford. It furthers the University's objective of excellence in research, scholarship, and education by publishing worldwide. Sign In or Create an Account. Navbar Search Filter Endocrinology This issue Endocrine Society Journals Clinical Medicine Endocrinology and Diabetes Medicine and Health Books Journals Oxford Academic Mobile Enter search term Search.
Endocrine Society Journals. Advanced Search. Search Menu. Article Navigation. Close mobile search navigation Article Navigation. Volume Article Contents Materials and Methods. Journal Article. Regulation of Glucagon Secretion at Low Glucose Concentrations: Evidence for Adenosine Triphosphate-Sensitive Potassium Channel Involvement.
Alvaro Muñoz , Alvaro Muñoz. Oxford Academic. Min Hu. Khalid Hussain. Joseph Bryan. Lydia Aguilar-Bryan. Arun S. Rajan, One Baylor Plaza, BCMA B, Houston, Texas PDF Split View Views. Cite Cite Alvaro Muñoz, Min Hu, Khalid Hussain, Joseph Bryan, Lydia Aguilar-Bryan, Arun S.
Select Format Select format. ris Mendeley, Papers, Zotero. enw EndNote. bibtex BibTex. txt Medlars, RefWorks Download citation. Permissions Icon Permissions. Close Navbar Search Filter Endocrinology This issue Endocrine Society Journals Clinical Medicine Endocrinology and Diabetes Medicine and Health Books Journals Oxford Academic Enter search term Search.
Open in new tab Download slide. TABLE 1. Insulin and glucagon secretion from WT and Sur1KO islets. Open in new tab. First Published Online August 25, and M. contributed equally to this work.
Google Scholar Crossref. Search ADS. Google Scholar PubMed. OpenURL Placeholder Text. Hypoglycaemia: the limiting factor in the glycaemic management of type I and type II diabetes. N Engl J Med. Glucose-inhibition of glucagon secretion involves activation of GABAA-receptor chloride channels.
Glucose inhibition of glucagon secretion from rat α-cells is mediated by GABA released from neighboring β-cells. Characterization of the effects of arginine and glucose on glucagon and insulin release from the perfused rat pancreas.
Glucagon secretion means it's official. Federal secrftion websites Glucagon secretion end Glucagon secretion. gov or. Before sharing sensitive information, make sure you're on a federal government site. The site is secure.Glucagon secretion -
Hypoglycemia-activated glut2 neurons of the nucleus tractus solitarius stimulate vagal activity and glucagon secretion. Steinbusch, L. Sex-specific control of fat mass and counterregulation by hypothalamic glucokinase.
Diabetes 65 , — Gylfe, E. Upsala J. Article PubMed PubMed Central Google Scholar. Matschinsky, F. Pancreatic beta-cell glucokinase: closing the gap between theoretical concepts and experimental realities. Diabetes 47 , — Froguel, P. Close linkage of glucokinase locus on chromosoms 7p to early-onset non-insulin-dependent diabetes mellitus.
Nature , — Article ADS CAS PubMed Google Scholar. Heimberg, H. The glucose sensor protein glucokinase is expressed in glucagon-producing alpha-cells. Natl Acad.
USA 93 , — Article ADS CAS PubMed PubMed Central Google Scholar. Guenat, E. Counterregulatory responses to hypoglycemia in patients with glucokinase gene mutations. Diabetes Metab. CAS PubMed Google Scholar. Herrera, P.
Adult insulin- and glucagon-producing cells differentiate from two independent cell lineages. Development , — Berg, J. A genetically encoded fluorescent reporter of ATP:ADP ratio. Methods 6 , — Briant, L.
Glucagon secretion from pancreatic alpha-cells. Walker, J. Regulation of glucagon secretion by glucose: paracrine, intrinsic or both?
Rorsman, P. ATP-regulated potassium channels and voltage-gated calcium channels in pancreatic alpha and beta cells: similar functions but reciprocal effects on secretion. Diabetologia 57 , — Meng, Z. Glucose Sensing by Skeletal Myocytes Couples Nutrient Signaling to Systemic Homeostasis.
Cell 66 , — e Seyer, P. Hepatic glucose sensing is required to preserve beta cell glucose competence. Invest , — Lee, Y. Glucagon is the key factor in the development of diabetes. Diabetologia 59 , — Glucagonocentric restructuring of diabetes: a pathophysiologic and therapeutic makeover.
Invest , 4—12 Mitrakou, A. Role of reduced suppression of glucose production and diminished early insulin release in impaired glucose tolerance. New Engl. Kawamori, D. Insulin signaling in alpha cells modulates glucagon secretion in vivo. Agius, L. Hormonal and metabolite regulation of hepatic glucokinase.
Nakamura, A. Present status of clinical deployment of glucokinase activators. Diabetes Investig. Panagiotidis, G. Homologous islet amyloid polypeptide: effects on plasma levels of glucagon, insulin and glucose in the mouse.
Diabetes Res. Download references. This work was supported by grants to B. from the Swiss National Science Foundation A0B and the European Research Council Advanced grants INSIGHT and INTEGRATE. was supported by a Diabetes UK RD Lawrence Fellowship.
PR was supported by the Wellcome Trust and the Swedish Research Council. PLH was funded by Fondation privée of the University Hospitals of Geneva and the NIDDK grant DK We thank the Mouse Metabolic Evaluation Facility MEF from the Center for Integrative Genomics for performing tissue glucose uptake measurements.
Center for Integrative Genomics, University of Lausanne, , Lausanne, Switzerland. Oxford Centre for Diabetes, Endocrinology, and Metabolism, University of Oxford, Churchill Hospital, Oxford, OX3 7LE, UK. Department of Clinical Science, UMAS, Division of Islet Cell Physiology, Lund, Sweden.
Department of Genetic Medicine and Development, , Geneva, Switzerland. You can also search for this author in PubMed Google Scholar. secured funding. performed the experiments. provided the the Gcg-Cre mice.
provided expertise and assisted with the editing of the manuscripts. Correspondence to Bernard Thorens. Publisher's note: Springer Nature remains neutral with regard to jurisdictional claims in published maps and institutional affiliations.
Open Access This article is licensed under a Creative Commons Attribution 4. Reprints and permissions. Basco, D. α-cell glucokinase suppresses glucose-regulated glucagon secretion.
Nat Commun 9 , Download citation. Received : 12 May Accepted : 15 January Published : 07 February Anyone you share the following link with will be able to read this content:. Sorry, a shareable link is not currently available for this article. Provided by the Springer Nature SharedIt content-sharing initiative.
Journal of Animal Science and Biotechnology By submitting a comment you agree to abide by our Terms and Community Guidelines. If you find something abusive or that does not comply with our terms or guidelines please flag it as inappropriate.
Sign up for the Nature Briefing newsletter — what matters in science, free to your inbox daily. Skip to main content Thank you for visiting nature. nature nature communications articles article. Download PDF.
Subjects Islets of Langerhans Nutrient signalling Pre-diabetes. Abstract Glucagon secretion by pancreatic α-cells is triggered by hypoglycemia and suppressed by high glucose levels; impaired suppression of glucagon secretion is a hallmark of both type 1 and type 2 diabetes.
Introduction Glucagon secretion by pancreatic α-cells is rapidly increased when the blood glucose concentration falls below the normoglycemic level to increase hepatic glucose production, and is suppressed by hyperglycemia 1 , 2.
Full size image. Discussion Here, we show that inactivation of Gck in α-cells results in hyperglucagonemia in the fed state and increased hepatic glucose production. Pancreatic islet isolation Before removal of the pancreas, a solution of Liberase TL 0. Hormone release measurements Measurements of insulin and glucagon secretion were performed using the static incubations of islets isolated from week-old mice.
In vivo biochemical measurements Plasma glucagon levels were quantitated by radioimmunoassay Merck Millipore and by ELISA Mercodia. Western blotting analysis A portion of mouse liver were homogenized in ice-cold homogeneisation buffer in mM: sucrose, 10 HEPES pH 7.
Radioactive 2-deoxy-glucose 2-DG uptake assay The animals were processed in the morning in the random-fed state.
Data representation and statistics All collected data were included without data exclusion. Data availability The data that support the findings of this study are available from the corresponding author upon reasonable request. References Unger, R. Article CAS PubMed Google Scholar Habegger, K.
Article CAS PubMed PubMed Central Google Scholar Zhang, Q. Article CAS PubMed PubMed Central Google Scholar Thorens, B. Article CAS PubMed Google Scholar Marty, N. Article CAS Google Scholar Hevener, A. Article CAS PubMed Google Scholar Burcelin, R.
Google Scholar Lamy, C. Several reports on experiments using genetic mouse models support the role of glucose-modulated K ATP channels in α-cell function. The regulation of glucagon secretion by glucose is impaired in ABCC8-deficient mice lacking functional K ATP channels Gromada et al.
A similar situation occurs in KCNJ11Y12X mouse with a KCNJ11 mutation in the K ATP channel MacDonald et al. In humans, the Glu23Lys polymorphism in the KCNJ11 subunit of these channels is associated with diminished suppression of glucagon release in response to hyperglycaemia Tschritter et al.
Nevertheless, since K ATP channels seem to be essential for the α-cell regulation in the proposed models, some considerations on glucose metabolism should be taken into account. Although α-cells possess the high-affinity, low-capacity glucose transporter SLC2A1, instead of the high-capacity SLC2A2 present in the β-cell, it has been demonstrated that glucose transport is not a limiting factor in α-cell glucose metabolism Gorus et al.
However, several studies indicate that important biochemical differences exist between both cell types. These biochemical differences indicate that β-cells are more efficient in the mitochondrial oxidation of glucose, while α-cells rely more on anaerobic glycolysis Schuit et al.
This lower coupling between glycolytic events in the cytosol and ATP synthesis in mitochondrial respiration of α-cells would explain the fact that, in response to glucose, cytosolic ATP increases are small in these cells Ishihara et al.
Therefore, some aspects at the above-mentioned models for α-cell stimulus-secretion coupling deserve more attention, especially those concerning the modulation of K ATP channel activity by glucose metabolism and ATP production.
Other mechanisms regulating K ATP channels may also have an important role. Although the lipotoxicity theory and its role in obesity-induced diabetes have increased the interest in the interactions between fatty acids and islet functions, little is known about their effect on the regulation of the α-cell compared with those on β-cells.
While initial studies suggested an inhibitory effect on glucagon secretion Andrews et al. The short-term stimulatory action depends on the chain length, spatial configuration and degree of saturation of the fatty acid Hong et al. The action of palmitate has been studied in mice at the cell level.
A study using clonal α-cells on the long-term effect of palmitate and oleate concluded that they also enhance glucagon secretion and triglyceride accumulation in a time- and dose-dependent manner but inhibit cell proliferation Hong et al.
In agreement with this, the long-term exposure of rat islets to fatty acids induces a marked increase in glucagon release, a decrease in glucagon content and no changes in glucagon gene expression Gremlich et al.
In addition to fatty acids, amino acids are also relevant in the modulation of the α-cell function. Amino acids such as arginine, alanine and glutamine are potent stimulators of glucagon secretion Pipeleers et al. In any case, the function of amino acids and fatty acids in the α-cell requires further investigation at the cellular and molecular levels.
The spatial distribution of α-cells and the vascular organization within the islet sustain an important intercellular communication through autocrine and paracrine mechanisms Fig. In addition to insulin, glucagon or somatostatin, secretory granules from islet cells contain other molecules with biological activity, which are released to the extracellular space by exocytosis, activating surface receptors in the same cell, in neighbouring islet cells, or in distant cells within the islet via the vascular system.
Several paracrine mechanisms are activated at high-glucose concentrations as a result of β- and δ-cell stimulations, and thus, they may participate in the glucose-induced inhibition of glucagon release.
Paracrine signalling in the α-cell. See text for details. ADCY, adenylate cyclase; AMPA-R, α-aminohydroxymethylisoxazolepropionic acid receptor; GABA, γ-aminobutyric acid; GLP1, glucagon-like peptide-1; GRM, metabotrophic glutamate receptor; PKA, protein kinase A; SSTR2, somatostatin receptor One of the most important paracrine mechanisms responsible for inhibiting glucagon release is conducted by insulin, acting via several pathways.
An appropriate expression of the insulin receptor in mouse α-cells seems to be essential for glucose-regulated glucagon secretion Diao et al. In INR1-G9 clonal α-cells, insulin has been found to inhibit glucagon release through the activation of phosphatidylinositol 3-kinase PIK3; Kaneko et al.
The insulin receptor—PIK3 signalling pathway is also involved in the modification of the sensitivity of K ATP channels to ATP in mouse α-cells, which may affect the secretory response Leung et al. Furthermore, insulin increases K ATP channel activity in isolated rat α-cells, inducing an inhibitory effect on glucagon release via membrane hyperpolarization Franklin et al.
In addition to the effects on K ATP channels, insulin can translocate A-type GABA receptors to the cell membrane, which increases the response to GABA secreted by β-cells, favouring membrane hyperpolarization and suppression of glucagon secretion Xu et al.
Therefore, several pieces of evidence indicate that insulin inhibits glucagon release mainly by altering α-cell membrane potential. After exocytosis, these hexameric crystals are exposed to a change in pH from 5.
Recent studies have claimed that zinc atoms can also work as modulators of the α-cell function Gyulkhandanyan et al. Somatostatin is produced and secreted by several tissues in addition to the δ-cell population of the islet and works as an inhibitor of both glucagon and insulin release Fehmann et al.
Immunocytochemical studies in human islets have demonstrated that, among the five identified somatostatin receptor SSTR subtypes, SSTR2 is highly expressed in α-cells while SSTR1 and SSTR5 are expressed in β-cells Kumar et al.
In mice and rats, SSTR2 also predominates in the α-cell and SSTR5 in the β-cell population Hunyady et al. These receptors are coupled to G-proteins and induce multiple intracellular effects. Also, a negative interaction of somatostatin with adenylate cyclase and cAMP levels has been reported in rat α-cells Schuit et al.
In addition to the effects of insulin and somatostatin on α-cells, glucagon itself works as an extracellular messenger.
It exerts an autocrine positive feedback that stimulates secretion in both isolated rat and mouse α-cells by an increase in exocytosis associated to a rise in cAMP levels Ma et al. The incretin hormone glucagon-like peptide 1 GLP1 is released from the L-cells of the small intestine after food intake, stimulating insulin production and inhibiting glucagon release.
Because of this dual effect, GLP1 is a potential therapeutic agent in the treatment of diabetic patients that manifest insulin deficiency as well as hyperglucagonaemia Dunning et al. The observed suppressing effect of GLP1 on glucagon secretion in vivo and in perfused pancreas contrasts with those effects found in single α-cells Dunning et al.
In isolated rat α-cells, GLP1 stimulates glucagon secretion by interacting with specific receptors coupled to G-proteins that activate adenylate cyclase, which increases cAMP levels Ding et al.
Thus, it seems that paracrine mechanisms may be responsible for the GLP1 suppressing action Dunning et al. This possibility has been underscored by the findings in experiments using β-cell-specific knock-out mice for the transcription factor Pdx1.
In these mice, the lack of effect of GLP1 on β-cells is also accompanied by its inability to induce an inhibitory action on glucagon plasma levels Li et al. The neurotransmitter γ-aminobutyric acid GABA is another α-cell modulator. Similar conclusions were obtained in mouse islets and clonal αTC1—9 cells Xu et al.
The neurotransmitter l -glutamate also accumulates in the α-cell secretory granules because of vesicular glutamate transporters 1 and 2 found in these cells Hayashi et al. In low-glucose conditions, l -glutamate is cosecreted with glucagon, triggering GABA release from neighbouring β-cells and, subsequently, inhibiting the α-cell function as previously described Hayashi et al.
Additionally, glutamate can activate autocrine signalling pathways in α-cells through the multiple glutamate receptors expressed in these cells, which include ionotrophic AMPA and kainate subtypes and metabotrophic receptors Inagaki et al.
Although activation of ionotrophic receptors may stimulate glucagon release Bertrand et al. Another α-cell regulator is amylin or islet amyloid pancreatic polypeptide Iapp. This polypeptide is a 37 amino acid hormone mainly synthesized in β-cells, although it can be produced in δ-cells as well.
This peptide is cosecreted with insulin by exocytosis and has an inhibitory effect on glucagon basal concentrations as well as on those levels observed after arginine stimulation Akesson et al.
This glucagonostatic effect has been reported in the plasma levels of mice and rats as well as in perfused pancreas or intact islets. Since amylin also reduces somatostatin and insulin release, some authors have proposed that endogen amylin within the islet may establish a negative feedback to avoid excessive secretion from α-, β- and δ-cells Wang et al.
Also, the purinergic messenger ATP is highly accumulated in β-cell secretory granules and in nerve terminals. Purinergic regulation of glucagon release has also been described in rat islets Grapengiesser et al. As previously stated, the islet of Langerhans is highly innervated by parasympathetic and sympathetic nerves that ensure a rapid response to hypoglycaemia and protection from potential brain damage Ahren Some terminals of these nerves store and release classical neurotransmitters, such as acetylcholine and noradrenaline, as well as several neuropeptides, which stimulate or inhibit glucagon secretion depending on the neural messenger released.
Noradrenaline increases glucagon secretion as well Ahren et al. In addition to classical neurotransmitters, several neuropeptides such as vasoactive intestinal polypeptide, pituitary adenylate cyclase-activating polypeptide and gastrin-releasing peptide, which may stimulate glucagon release from pancreas, can be accumulated in parasympathetic nerves, while galanin and neuropeptide Y can be stored in sympathetic nerve terminals Ahren Multiple actions have been reported for the latter neuropeptides.
The effects and mechanisms involved in neural regulation of α-cells have yet to be established at the cellular and molecular levels. These systems are mainly regulated by glucose-sensing neurons of the ventromedial hypothalamus, which respond to plasma glucose levels with mechanisms very similar to those of the β-cell, including the activity of glucose-regulated K ATP channels Borg et al.
Actually, it has been observed that the α-cell response to hypoglycaemia is also impaired in KCNJdeficient mice whose neurons of the ventromedial hypothalamus lack functional K ATP channels and glucose responsiveness Miki et al. The preproglucagon-derived peptides glucagon, GLP1 and GLP2, are encoded by the preproglucagon gene, which is expressed in the central nervous system, intestinal L-cells and pancreatic α-cells.
A post-translational cleavage by prohormone convertases PC is responsible for the maturation of the preproglucagon hormone that generates all these peptides Mojsov et al. The different expression of PC subtypes in each tissue mediates the production of each different peptide. In α-cells, the predominance of PCSK2 leads to a major production of glucagon together with the products glicentin, glicentin-related pancreatic polypeptide, intervening peptide 1 and the major proglucagon fragment Dey et al.
The absence of PCSK2 in knock-out mice leads to a lack of mature glucagon Furuta et al. The regulation of glucagon gene expression has not been studied as extensively as the insulin gene. The inhibitory effect of insulin on glucagon secretion has also been confirmed in gene expression and it occurs at the transcriptional level Philippe et al.
In diabetic rats, glucagon gene expression is augmented and is accompanied by hyperglucagonaemia in conditions of hyperglycaemia and insulin deficiency.
Insulin treatment normalized glucagon expression and plasma levels in these rats, an effect that was not attributed to the restoration of normal glucose levels Dumonteil et al. It was concluded that insulin, unlike glucose, modulates glucagon expression.
The lack of response to glucose was further confirmed in isolated rat islets Gremlich et al. The effect of amino acids on glucagon gene regulation has also been studied. While arginine increases glucagon expression in isolated rat islets: a process that is mediated by protein kinase C PKA; Yamato et al.
Other nutrients, such as the fatty acid palmitate, produces a down-regulated glucagon expression at short term in rat islets in a dose-dependent manner Bollheimer et al. By contrast, no effect with palmitate has been observed in other long-term studies Gremlich et al.
Like insulin, somatostatin also inhibits glucagon expression. It has been reported that somatostatin down-regulates glucagon expression basal levels as well as those produced by forskolin stimulation in clonal INR1G9 cells Fehmann et al.
The rat and mouse glucagon receptor is a amino acid protein, belonging to the secretin—glucagon receptor II class family of G protein-coupled receptors Mayo et al. Glucagon binding to this receptor is coupled to GTP-binding heterotrimeric G proteins of the Gα s type that leads to the activation of adenylate cyclase, cAMP production and PKA.
The glucagon receptor is present in multiple tissues including the liver, pancreas, heart, kidney, brain and smooth muscle. Thus, it modulates multiple responses in these tissues, including effects on ion transport and glomerular filtration rate in kidney among others Ahloulay et al.
In any case, the regulation of glucose homeostasis is the major function of glucagon and its receptor. This role will be described in the next paragraph.
The role of glucagon and the glucagon receptor in the liver. ADCY, Adenylate cyclase; CREB, cAMP response element binding; F 1,6 P2, fructose-1,6-bisphosphate; F 2,6 P2, fructose-2,6-bisphosphate; FP, fructose 6-phosphate; FBP1, fructose-1,6-bisphosphatase; FBP2, fructose-2,6-bisphosphatase; GP, glucose 1-phosphate; GP, glucose 6-phosphate; G6PC, glucosephosphatase; GP, glycogen phosphorylase; GS, glycogen synthase; IP3, inositol 1,4,5-trisphosphate; OAA, oxaloacetate; PC, pyruvate carboxylase; PEP, phosphoenolpyruvate; PCK2, phosphoenolpyruvate carboxykinase; PFKM, phosphofructokinase-1; PPARGC1A, peroxisome proliferators-activated receptor-γ coactivator-1; PIP2, phosphatidylinositol 4,5-bisphosphate; PKLR, pyruvate kinase; PLC, phospholipase C; Pyr, pyruvate.
Dashed lines: red, inhibition; blue, stimulation. Several lines of defence protect the organism against hypoglycaemia and its potential damaging effects, especially in the brain, which depends on a continuous supply of glucose, its principal metabolic fuel. These defences include decreased insulin release and increased secretion of adrenaline and glucagon.
Additionally, glucose-sensing neurons of the ventromedial hypothalamus further control responses to glycaemia changes, as previously mentioned. Among all these regulatory systems, glucagon plays a central role in the response to hypoglycaemia and also opposes to insulin effects. Glucagon stimulates gluconeogenesis and glycogenolysis, which increases hepatic glucose output, ensuring an appropriate supply of glucose to body and brain, and at the same time, it decreases glycogenesis and glycolysis.
The glucagon receptor in the liver is highly selective for glucagon, but it exhibits a modest affinity for glucagon-like peptides Hjorth et al. Its main action on the liver is mediated by the activation of adenylyl cyclase and the PKA pathway. Glucagon regulates gluconeogenesis mainly by the up-regulation of key enzymes such as glucosephosphatase G6PC and phosphoenolpyruvate carboxykinase PCK2 through the activation of the cAMP response element-binding protein CREB and peroxisome proliferator-activated receptor γ-coactivator-1 PPARGC1A; Herzig et al.
PCK2 and G6PC, along with fructose-1,6-biphosphatase FBP1 have a key role in the rate of gluconeogenesis Fig. PCK2 mediates the conversion of oxalacetate into phosphoenolpyruvate while G6PC regulates glucose production from glucosephosphate.
FBP1 is responsible for the conversion of fructose-1,6-biphosphate F 1,6 P2 into fructosephosphate F6P. Additionally, this decrease in F 2,6 P2 also reduces the activity of phosphofructokinase-1 PFKM , down-regulating glycolysis.
The glycolytic pathway is further inhibited by glucagon at the pyruvate kinase PKLR level Slavin et al. Glycogen metabolism is mainly determined by the activity of glycogen synthase GS and glycogen phosphorylase GP.
Glucagon can also stimulate the uptake of amino acids for gluconeogenesis in the liver. Indeed, subjects with hyperglucagonaemia can develop plasma hypoaminoacidaemia, especially of amino acids involved in gluconeogenesis, such as alanine, glycine and proline Cynober Glucagon is also involved in the regulation of fatty acids in adipocytes.
Hormone-sensitive lipase mediates the lipolysis of triacylglycerol into the non-esterified fatty acids and glycerol, which are released from adipocytes.
It has been reported that although glucagon does not modify the transcriptional levels of this enzyme, it increases the release of glycerol from adipocytes Slavin et al. This mobilization of glycerol from adipose tissue can further be used in the liver during gluconeogenesis.
However, the existence of a lipolytic action of glucagon observed in several animal models is still controversial in humans. While a positive effect of glucagon on lipolysis has been reported in human subjects Carlson et al.
An elevated glucagon to insulin ratio accelerates gluconeogenesis as well as fatty acid β-oxidation and ketone bodies formation Vons et al.
Thus, glucagon may also be involved in diabetic ketoacidosis, a medical complication in diabetes derived from the overproduction of ketone bodies Eledrisi et al.
According to this hypothesis, this metabolic disease is the result of an insulin deficiency or resistance along with an absolute or relative excess of glucagon, which can cause a higher rate of hepatic glucose production than glucose utilization, favouring hyperglycaemia.
At present, there exists multiple clinical and experimental evidence that support this hypothesis. The rate of hepatic glucose output has been correlated with the hyperglycaemia found in animal models of diabetes as well as in human diabetes, and the maintenance of this abnormality has also been associated with hyperglucagonaemia Baron et al.
In type 2 diabetes, the impairment of insulin release and development of insulin resistance is often accompanied by absolute or relative increased levels of glucagon in the fasting and postprandial states Reaven et al. In this situation, insulin is not effective as a negative feedback for hepatic glucose output while glucagon potentiates glucose mobilization from the liver, thus contributing to hyperglycaemia.
Another malfunction reported in diabetic patients is the lack of suppression of glucagon release in hyperglycaemic conditions, which would contribute further to postprandial hyperglycaemia in both type 1 and type 2 diabetes Dinneen et al.
However, this irregular α-cell behaviour does not occur when insulin levels are adequate, suggesting that abnormalities in glucagon release are relevant for hyperglycaemia in the context of diabetes or impairment of insulin secretion or action Shah et al.
Hyperglucagonaemia is also responsible for the development of hyperglycaemia and diabetes in patients with the glucagonoma syndrome, a paraneoplastic phenomenon characterized by an islet α-cell pancreatic tumour Chastain Another defect in normal glucagon secretion has important consequences in the management of hypoglycaemia.
The secretory response of α-cells to low-glucose concentrations is impaired in type 1 and long-lasting type 2 diabetes, increasing the risk of episodes of severe hypoglycaemia, especially in patients treated with insulin Cryer In this regard, iatrogenic hypoglycaemia is a situation that implies insulin excess and compromised glucose counter-regulation, and it is responsible for a major complication in diabetes treatment, increasing the morbidity and mortality of this disease Cryer This lack of glucagon response to hypoglycaemia has been associated with multiple failures in α-cell regulation; yet, the mechanisms are still under study Bolli et al.
Even though islet allotransplantation can provide prolonged insulin independence in patients with type 1 diabetes, the lack of α-cell response to hypoglycaemia usually persists after transplantation, indicating that this procedure does not restore the physiological behaviour of α-cells Paty et al.
All these problems in the glucagon secretory response observed in diabetes have been attributed to several defects in α-cell regulation including defective glucose sensing, loss of β-cell function, insulin resistance or autonomic malfunction.
However, the mechanisms involved in α-cell pathophysiology still remain largely unknown and deserve more investigation for better design of therapeutic strategies. In this regard, although direct therapeutic approaches to correct the lack of α-cell response to hypoglycaemia are missing, several proposals have been developed to amend glucagon excess, as we will see in the next section.
The specific control of glucagon secretion by pharmacological modulation is complex since several components of the α-cell stimulus-secretion coupling are also present in β- and δ-cells. Thus, the manipulation of glucagon action by modulating the glucagon receptor signalling seems to be an effective alternative Li et al.
This strategy has been supported by several studies. Glucagon receptor knock-out mice have hyperglucagonaemia and α-cell hyperplasia, but their glucose tolerance is improved and they develop only a mild fasting hypoglycaemia Gelling et al. These mice have a normal body weight, food intake and energy expenditure although less adiposity and lower leptin levels.
These results are consistent with the experiments with anti-sense oligonucleotides for the glucagon receptor. Therefore, these experimental results are a further support that glucagon antagonism may be beneficial for diabetes treatment. Sulphonylureas are efficient K ATP channel blockers that have been extensively used for the clinical treatment of diabetes.
This biphasic effect is due to the mouse α-cell electrical behaviour Fig. Accordingly, with this scheme, the K ATP channel opener diazoxide can also have a biphasic effect on glucagon secretion.
These effects will change depending on the extracellular glucose concentrations that necessarily influence K ATP channel activity MacDonald et al. This biphasic behaviour may explain the disparity of effects found for sulphonylureas Loubatieres et al.
In humans, sulphonylureas are associated to a glucagon secretion decrease in healthy and type 2 diabetic subjects Landstedt-Hallin et al. Since sulphonylureas also induce insulin and somatostatin secretion, which affect α-cells, these drugs offer a poor specific control of glucagon secretion.
In addition to stimulating insulin release, GLP1 can suppress glucagon secretion in humans, perfused rat pancreas and isolated rat islets in a glucose-dependent manner Guenifi et al. Because GLP1 is rapidly cleaved and inactivated by the enzyme dipeptidyl peptidase-IV DPP4 , a good alternative would be to design either GLP1 derivatives with higher resistance to DPP4 or agents that increase GLP1 endogenous levels.
Among the GLP1 mimetics, exenatide is a synthetic polypeptide with high resistance to DPP4 cleavage that decreases glucagon levels in normal and diabetic subjects Degn et al. Liraglutide, another GLP1 derivative with long-lasting actions, can reduce glucagon release after a meal in patients with type 2 diabetes Juhl et al.
Alternatively, DPP4 inhibitors like sitagliptin and vildagliptin increase the endogen effects of GLP1, reducing glucagon plasma concentrations in diabetic individuals Rosenstock et al. Since all these alternatives produce opposing actions on insulin and glucagon, they generate promising expectations for diabetes treatment.
Given that imidazoline compounds stimulate insulin release while inhibiting glucagon secretion, these drugs are potentially valuable in diabetes.
Because of the different expression of SSTR in the islet Kumar et al. It has been shown that SSTR2 is the subtype receptor predominantly expressed in rodent α-cells, and that SSTR2-deficient mice develop hyperglycaemia and non-fasting hyperglucagonaemia Singh et al. In mice, the use of a highly SSTR2-selective non-peptide agonist inhibited glucagon release without affecting insulin release Strowski et al.
However, there is some overlapping in human islets between the different SSTR subtypes in α- and β-cells that limit, at present, the use of subtype-specific somatostatin analogues Singh et al.
Amylin, which is cosecreted with insulin from β-cells, inhibits glucagon secretion stimulated by amino acids but does not affect hypoglycaemia-induced glucagon release Young Since α-cell response to amino acids is often exaggerated in diabetic patients, amylin or amylinomimetic compounds such as pramlintide are used as an effective alternative for the treatment of postprandial and amino acid-induced excess of glucagon secretion Dunning et al.
Several linear and cyclic glucagon analogues have been developed to work as glucagon receptor antagonists. Essentially, they impair the ability of glucagon to stimulate adenylate cyclase activity in liver, thus reducing hepatic glucose output and improving plasma glucose levels.
This is the case of [des-His 1 , des-Phe 6 , Glu 9 ] glucagon-NH 2 , which reduces glucose levels in streptozotocin-induced diabetic rats Van Tine et al. Recent investigations have demonstrated that the antagonist des-His-glucagon binds preferentially to the hepatic glucagon receptor in vivo , and this correlates with the glucose lowering effects Dallas-Yang et al.
For instance, a novel competitive antagonist N -[3-cyano 1, 1-dimethylpropyl -4, 5, 6, 7-tetrahydrobenzothienyl]ethylbutanamide was recently shown to inhibit glucagon-mediated glycogenolysis in primary human hepatocytes and to block the increase in glucose levels after the administration of exogenous glucagon in mice Qureshi et al.
The information about the effect of these antagonists on humans is, however, scarce. Despite the success of several approaches to modulate glucagon secretion or action and improve glucose control in animal models or in humans, more information is still required. Long-standing studies should address whether the utilization of these agents could lead to undesired hypoglycaemia in humans, accumulation of lipids or compensatory mechanisms that decrease the benefits of these therapies in the long term.
In this aspect, the results obtained in animal models are positive: although the glucagon receptor knock-out mouse develops hyperglucagonaemia, it is not hypoglycaemic and does not have an abnormal accumulation of lipids Gelling et al.
Additionally, recent long-term studies in mice further prove the viability of glucagon antagonism Winzell et al.
Thus, present data are promising and indicate that several therapeutic agents targeted to glucagon signalling and α-cell secretion may be useful for the management of diabetes. Pancreatic α-cells and glucagon secretion are fundamental components of the regulatory mechanisms that control glucose homeostasis.
However, α-cell physiology has remained elusive compared with the overwhelming information about insulin secretion and the β-cell. In recent years, however, several groups have initiated intensive efforts to understand α-cell physiology and identified essential pieces of its stimulus-secretion coupling.
Additionally, important aspects of the regulation of α-cell metabolism and the control of glucagon expression are being elucidated. All of this information will favour an overall comprehension of the α-cell function and its role in glucose homeostasis.
Nevertheless, more research is required to understand the α-cell behaviour, not only in healthy subjects but in pathological conditions as well. In conclusion, since the malfunction of the glucagon secretory response is involved in diabetes and its complications, a complete understanding of the α-cell will allow for a better design of therapeutic approaches for the treatment of this disease.
The authors declare that there is no conflict of interest that could be perceived as prejudicing the impartiality of the research reported. This work was supported by grants from the Ministerio de Educación y Ciencia BFU and PCIA to I Q; BFU to A N.
Hypoglycemia stimulates the pancreatic alpha cell to release glucagon and hyperglycemia inhibits glucagon secretion Fig. The cellular mechanism behind this glucose-dependent regulation of glucagon secretion involves uptake of glucose by the glucose transporter 1 GLUT1 in the cell membrane of pancreatic alpha cells and subsequent glycolysis which ultimately generates adenosine triphosphate ATP in the mitochondria of the alpha cell.
Thus, the intracellular ATP level in the alpha cell reflects plasma glucose levels. Conversely, increasing circulating glucose levels increase glucose influx to the alpha cell generating an increase in intracellular ATP concentration, which opens K ATP -channels.
Glucose-dependent glucagon secretion from the alpha cell. During hypoglycemia intracellular glucose concentration falls with a subsequent reduction in glycolysis-generated adenosine triphosphate ATP in the mitochondria of the cell. depolarization of the cell membrane. In normal physiology, circulating glucagon concentrations are in the picomolar range.
Basal glucagon secretion balances the effect of basal insulin secretion resulting in a steady-state between glucose uptake and endogenous glucose production in the fasted state; i. stable blood glucose concentrations. During exercise or in case of hypoglycemia, circulating glucagon levels may increase dramatically to times basal levels increasing the glucagon to insulin ratio 12 , 19 , 20 Fig.
The effects of glucagon are mediated through binding to and activation of the glucagon receptor. The glucagon receptor is a seven transmembrane G protein-coupled receptor Fig. The main mode of intracellular signaling involves activation of G s and G q.
G s activation stimulates adenylyl cyclase which produces cyclic adenosine monophosphate cAMP that activates protein kinase A PKA. The activated PKA migrates to the nucleus and activates transcription factors like cAMP response element-binding protein CREB through phosphorylation.
This enables CREB to bind to response elements of target genes resulting in the recruitment of coactivators and ultimately promoting gene expression. Activation of G q by glucagon leads to activation of phospholipase C PLC and subsequent increase in inositol 1,4,5-triphosphate IP 3 , which signals to enhance release of calcium from the endoplasmic reticulum.
This, in turn, activates downstream signaling cascades including CREB-regulated transcription co-activator CRTC2 which enhance CREB-dependent gene expression. In addition to the CREB-CRTC2 pathway, glucagon may signal through various other pathways reviewed in detail elsewhere 1 , 12 , Examples of the two most well-described intracellular pathways involved in glucagon-induced regulation of target gene expression: the PKA and the IP 3 pathways.
AC, adenylyl cyclase; CRTC2, CREB-regulated transcription co-activator; CREB, cAMP response element-binding protein; IP 3 , inositol 1,4,5-triphosphate; PIP 2 , phosphatidyl-inositol-4,5-bisphosphate; PKA, protein kinase A; PLC, phospholipase C.
The degradation of glucagon is mainly facilitated by receptor-mediated endocytosis and proteolysis by the ubiquitous enzyme dipeptidyl peptidase 4 22 , Consistent with the relative receptor expression, the liver and kidneys seem to represent the two main organs removing glucagon from the circulation.
The circulating half-life of glucagon in plasma is reported to be between four to seven minutes in humans 24 , Glucagon controls plasma glucose concentrations during fasting, exercise and hypoglycemia by increasing hepatic glucose output to the circulation.
Specifically, glucagon promotes hepatic conversion of glycogen to glucose glycogenolysis , stimulates de novo glucose synthesis gluconeogenesis , and inhibits glucose breakdown glycolysis and glycogen formation glycogenesis Fig. Hepatic glucose production is rapidly enhanced in response to a physiological rise in glucagon; achieved through stimulation of glycogenolysis with minor acute changes in gluconeogenesis 27 , This ability of glucagon is critical in the life-saving counterregulatory response to severe hypoglycemia.
Additionally, it is a key factor in providing adequate circulating glucose for brain function and for working muscle during exercise During prolonged fasting, glycogen stores are depleted, and gluconeogenesis takes over The hyperglycemic property of glucagon is enhanced when hepatic glycogen levels are high and diminished when hepatic glycogen levels are low in conditions of fasting or liver diseases like cirrhosis Regulation of glucose metabolism by glucagon in the liver.
Glucagon increases hepatic glucose production by stimulating glycogenolysis and glycogenogenesis green arrows while inhibiting glycolysis and glycogenesis red arrows. Glucagon promotes formation of non-carbohydrate energy sources in the form of lipids and ketone bodies. Thereby, glucagon contributes to a stable energy homeostasis during conditions where energy supply is limited fasting or in states of increased energy demand e.
exercise or cold exposure Specifically, in times of energy demand, glucagon enhances break-down of fatty acids to acetyl-coenzyme A molecules beta-oxidation in the liver. These intermediates are either reduced to generate ATP in the tricarboxylic acid cycle or converted to ketone bodies ketogenesis — a process also stimulated by glucagon.
Furthermore, glucagon signaling inhibits de novo lipogenesis by inactivating the enzyme that catalyzes the first step in fatty acid synthesis from other substrates like carbohydrates During prolonged fasting, glucagon stimulates formation of glucose from amino acids via gluconeogenesis by upregulating enzymes involved in the process.
However, the rate-limiting step of the process depends on the supply of gluconeogenic amino acids from muscle or dietary intake, a process not controlled by glucagon In addition to enter gluconeogenesis, amino acids are deaminated to generate ATP in the liver.
Glucagon is involved in this process by promoting the conversion of ammonia — a toxic biproduct from deamination — to urea, which is excreted in the urine. Thereby glucagon reduces ammonia levels in the blood Disruption of glucagon action by inhibition of the glucagon receptor 37 leads to increased plasma levels of amino acids and pancreatic alpha cell hyperplasia, which in turn, leads to glucagon hypersecretion.
This suggests that glucagon and amino acids are linked in a feedback loop between the liver and the pancreatic alpha cells Acute administration of glucagon has been shown to reduce food intake and diminish hunger 38 , Conversely, preprandial inhibition of glucagon signaling increases food intake in rats 40 , 41 providing evidence for a role of glucagon in the regulation of appetite.
It is somewhat counterintuitive that glucagon should reduce food intake given that glucagon levels are typically elevated upon fasting and decrease upon feeding. Thus, the observed effect upon glucagon administration in supraphysiological concentrations could partly be due to cross-reactivity with the GLP-1 receptor which normally result in suppression of food intake In addition to a potential effect of glucagon on food intake, evidence suggests that glucagon contributes to a negative energy balance by stimulating energy expenditure.
In humans, this effect has been observed in studies in which glucagon infusion resulted in increases in resting energy expenditure 42 — However, the effect of endogenous glucagon on resting energy expenditure remains unclear.
Also, the exact mechanisms behind the increase in resting energy expenditure elicited by exogenous glucagon remain to be determined.
It has been speculated that glucagon activates brown adipose tissue 12 , however this was recently challenged in an in vivo study that found no direct effect of glucagon on brown adipose tissue Rodent studies indicate that the actions of glucagon to increase energy expenditure might be indirectly mediated partly by fibroblast growth factor 21 FGF21 as glucagon-induced increase in energy expenditure is abolished in animals with FGF21 receptor deletion Infusion of high doses of glucagon increases heart rate and cardiac contractility In fact, infusion of glucagon in pharmacological doses milligram is often used in the treatment of acute cardiac depression caused by calcium channel antagonist or beta-blocker overdoses 47 despite limited evidence In comparison, glucagon concentrations within the normal physiological range do not appear to affect heart rate or contractility 49 and any physiological role of endogenous glucagon in the regulation of pulse rate remains questionable.
This is supported by studies investigating the effect of glucagon receptor antagonist for the treatment of type 2 diabetes in which no effect of pulse rate were observed Nevertheless, whether increased glucagon concentrations have a sustained effect on the heart remains unknow.
Of note, most studies use bolus injections of glucagon which cause only a transient increase in heart rate and contractility potentially reflecting the rapid elimination of glucagon from circulation Taken together, it remains uncertain whether glucagon has a place in the treatment of heart failure or hold a cardioprotective effect in healthy subjects.
Patients with type 2 diabetes exhibit an impaired regulation of glucagon secretion which contributes importantly to diabetic hyperglycemia.
Specifically, type 2 diabetes is characterized by elevated levels of glucagon during fasting while suppression of glucagon in response to oral intake of glucose is impaired or even paradoxically elevated Fig.
The mechanisms behind hyperglucagonemia are not fully understood but is usually explained by a diminished suppressive effect of insulin on alpha cells due to hypoinsulinemia and insulin resistance at the level of the alpha cells 53 , Interestingly, subjects with type 2 diabetes, who exhibit a hyperglucagonemic response to oral glucose, respond with a normal suppression of glucagon after intravenous glucose administration Accordingly, hormones secreted from the gastrointestinal tract may play an important role 55 , It has recently been confirmed that glucagon can be secreted from extrapancreatic tissue demonstrated in experiments with totally pancreatectomized subjects This supports the notion that postprandial hypersecretion of glucagon in patients with type 2 diabetes might be of extrapancreatic origin.
Schematic illustration of plasma glucagon concentrations in patients with type 2 diabetes and in normal physiology healthy subjects. Type 2 diabetes is characterized by elevated fasting plasma glucagon levels and impaired suppression of plasma glucagon levels in response to oral glucose.
Traditionally type 1 diabetic hyperglycemia has been explained by selective loss of beta cell mass and resulting decrease in insulin secretion. However, emerging evidence indicate that glucagon plays a major role in type 1 diabetes pathophysiology. The glucagon dyssecretion that characterizes patients with type 1 diabetes is associated with two clinical manifestations: Postprandial hyperglucagonemia and impaired glucagon counterregulation to hypoglycemia Data regarding fasting plasma glucagon concentrations in type 1 diabetes are inconsistent 57 , Thus, the general notion that glucagon hypersecretion plays a role in type 1 diabetes hyperglycemia is mainly based on elevated postprandial glucagon concentrations The explanation behind this is unclear, although a common explanation is, that in type 1 diabetes the postprandial increase in plasma glucose is not followed by an increase in insulin secretion from beta cells, which in normal physiology would inhibit glucagon secretion.
The absence of that restraining signal from endogenous insulin could result in an increase in glucagon secretion from alpha cells after a meal Fig. However, like in type 2 diabetes, subjects with type 1 diabetes preserve their ability to suppress glucagon after intravenous glucose administration.
Schematic illustration of plasma glucagon concentrations in patients with type 1 diabetes and in normal physiology healthy subjects. Type 1 diabetes is characterized by elevated concentrations of glucagon in response to a meal or oral glucose intake.
Hypoglycemia is a frequent and feared side effect of insulin therapy in type 1 diabetes and it represents a common barrier in obtaining glycemic control In normal physiology hypoglycemia is prevented by several mechanisms: 1 Reduced insulin secretion from beta cells diminishing glucose uptake in peripheral tissues; 2 increased glucagon secretion from alpha cells increasing hepatic glucose output; and 3 increased symphathetic neural response and adrenomedullary epinephrine secretion.
The latter will stimulate hepatic glucose production and cause clinical symptoms that enables the individual to recognize hypoglycemia and ultimately ingest carbohydrates 57 , 61 , In type 1 diabetes, insulin-induced hypoglycemia fails to elicit adequate glucagon responses compromising counterregulation to insulin-induced hypoglycemia; a phenomenon which seems to worsen with the duration of type 1 diabetes.
This defect likely involves a combination of defective alpha cells and reduced alpha cell mass 57 , Dysregulated glucagon secretion is not only observed in patients with type 2 diabetes but also in normoglucose-tolerant individuals with obesity 64 and patients with non-alcoholic fatty liver disease NAFLD 65 , This suggests that dysregulated glucagon secretion may represent hepatic steatosis rather than dysregulated glucose metabolism.
Interestingly, fasting hyperglucagonemia seems to relate to circulating amino acids in addition to hepatic fat content This hyperaminoacidemia suggests that impairment of amino acid turnover in the liver and ensuing elevations of circulating amino acids constitutes a feedback on the alpha cell to secrete more glucagon with increasing hepatic amino acid turnover and ureagenesis needed for clearance of toxic ammonia from the body.
The implication of hyperglucagonemia in obesity and NAFLD has renewed the scientific interest in actions of glucagon and the role of glucagon in the pathophysiology of these metabolic disorders.
Clearly, glucagon may represent a potential target for treatments of obesity and NAFLD. A simple way to restrain the undesirable hyperglycemic effect of glucagon while realizing its actions on lipolysis and energy expenditure could be by co-treating with a glucose-lowering drug.
This may be done by mimicking the gut hormone oxyntomodulin which acts as a ligand to both the glucagon and the GLP-1 receptor. Glucagon is a glucoregulatory peptide hormone that counteracts the actions of insulin by stimulating hepatic glucose production and thereby increases blood glucose levels.
Additionally, glucagon mediates several non-glucose metabolic effects of importance for maintaining whole-body energy balance in times of limited nutrient supply. These actions include mobilization of energy resources through hepatic lipolysis and ketogenesis; stimulation of hepatic amino acid turnover and related ureagenesis.
Also, glucagon has been shown to increase energy expenditure and inhibit food intake, but whether endogenous glucagon is involved in the regulation of these processes remains uncertain.
Glucagon plays an important role in the pathophysiology of diabetes as elevated glucagon levels observed in these patients stimulate hepatic glucose production, thereby contributing to diabetic hyperglycemia.
Used under Creative Commons License 3. This electronic version has been made freely available under a Creative Commons CC-BY-NC-ND license. Turn recording back on. National Library of Medicine Rockville Pike Bethesda, MD Web Policies FOIA HHS Vulnerability Disclosure.
Help Accessibility Careers. Access keys NCBI Homepage MyNCBI Homepage Main Content Main Navigation. Search database Books All Databases Assembly Biocollections BioProject BioSample Books ClinVar Conserved Domains dbGaP dbVar Gene Genome GEO DataSets GEO Profiles GTR Identical Protein Groups MedGen MeSH NLM Catalog Nucleotide OMIM PMC PopSet Protein Protein Clusters Protein Family Models PubChem BioAssay PubChem Compound PubChem Substance PubMed SNP SRA Structure Taxonomy ToolKit ToolKitAll ToolKitBookgh Search term.
Show details Feingold KR, Anawalt B, Blackman MR, et al. Contents www. Search term. Glucagon Physiology Iben Rix , Christina Nexøe-Larsen , Natasha C Bergmann , Asger Lund , and Filip K Knop. hnoiger nesretep. Christina Nexøe-Larsen Center for Clinical Metabolic Research, Gentofte Hospital, University of Copenhagen, Hellerup, Denmark, Department of Clinical Medicine, Faculty of Health and Medical Sciences, University of Copenhagen, Copenhagen, Denmark.
Natasha C Bergmann Center for Clinical Metabolic Research, Gentofte Hospital, University of Copenhagen, Hellerup, Denmark. Asger Lund Center for Clinical Metabolic Research, Gentofte Hospital, University of Copenhagen, Hellerup, Denmark. Filip K Knop Center for Clinical Metabolic Research, Gentofte Hospital, University of Copenhagen, Hellerup, Denmark, Department of Clinical Medicine, Faculty of Health and Medical Sciences, University of Copenhagen, Copenhagen, Denmark, Novo Nordisk Foundation Center for Basic Metabolic Research, Faculty of Health and Medical Sciences, University of Copenhagen, Copenhagen, Denmark; Steno Diabetes Center Copenhagen, Gentofte, Denmark Email: kd.
hnoiger ABSTRACT Glucagon is a peptide hormone secreted from the alpha cells of the pancreatic islets of Langerhans. STRUCTURE AND SYNTHESIS OF GLUCAGON Glucagon is a amino acid peptide hormone predominantly secreted from the alpha cells of the pancreas.
GLUCAGON SECRETION Glucagon is secreted in response to hypoglycemia, prolonged fasting, exercise and protein-rich meals Regulation of Glucagon Secretion by Glucose The most potent regulator of glucagon secretion is circulating glucose.
The secretion of Sfcretion by Gluagon α-cells plays a critical Glucqgon in Antioxidant compounds in red wine regulation of Glucagon secretion. Glcagon hormone secrerion hypoglycaemia and Glucagon secretion insulin actions by stimulating hepatic Balanced nutrition synthesis Glucagom mobilization, thereby increasing blood glucose Glucagon secretion. During the last Glucagon secretion, knowledge of α-cell physiology has greatly improved, especially concerning molecular and cellular mechanisms. In this review, we have addressed recent findings on α-cell physiology and the regulation of ion channels, electrical activity, calcium signals and glucagon release. Our focus in this review has been the multiple control levels that modulate glucagon secretion from glucose and nutrients to paracrine and neural inputs. Additionally, we have described the glucagon actions on glycaemia and energy metabolism, and discussed their involvement in the pathophysiology of diabetes.Glucagon is a secretioh hormone Glucaton, produced by seretion cells of the pancreas. GGlucagon raises the concentration Gluccagon glucose Glucagon secretion fatty eecretion in secretino bloodstream and Colon cleanse for a healthy gut considered to be the main catabolic hormone of the Glucagon secretion.
Its secrdtion is opposite to that secgetion insulinwhich Glucwgon Glucagon secretion glucose. The Glucahon releases Glucago when the amount secreion glucose in the bloodstream Glucagln too low. Glucagon Website performance tips the liver to engage in glycogenolysis : converting Sdcretion glycogen into glucosewhich Gulcagon released into the secretiln Insulin allows glucose seretion be taken up and used by insulin-dependent Glucagob.
Thus, glucagon and insulin are Glucwgon of a feedback system that keeps blood glucose Glucagpn stable. Glucagon Holistic approach to stress management energy secretoin and is elevated under conditions of stress.
Glucagon sefretion a amino acid polypeptide. The polypeptide has a Glucagon secretion mass Glucsgon daltons. The hormone is synthesized and secreted from alpha cells srcretion of the islets secrretion Langerhanswhich are located in the endocrine portion of the pancreas.
Glucagon is produced from secetion preproglucagon gene Gcg. Preproglucagon first has its signal sedretion removed by signal peptidaseforming the amino acid protein secreion.
In Glcagon Glucagon secretion cellsproglucagon Glcagon cleaved to the alternate secrretion glicentin 1—69 Glucagpn, glicentin-related pancreatic Glucgon 1—30oxyntomodulin 33—69glucagon-like peptide 1 72— or Strategic thinking in athletics, and sscretion peptide 2 — In rodents, the alpha cells are located in Glucagon secretion outer secreiton of the islet.
Human islet structure is much secrftion segregated, and alpha cells are distributed throughout the islet in close sceretion to beta cells.
Gljcagon is also produced by alpha cells Glucgaon the stomach. Recent research has demonstrated that glucagon production may also take secrdtion outside the pancreas, with Athletic performance beverage gut being the most likely site of extrapancreatic glucagon synthesis.
Glucago generally Secrstion the concentration of glucose secretipn the decretion by promoting gluconeogenesis and glycogenolysis, Glucagon secretion. Glucose is stored in secrteion liver in the form of the polysaccharide glycogen, which Glucahon a glucan Gulcagon polymer made up Gljcagon glucose molecules.
Liver secreetion hepatocytes have glucagon secrtion. When glucagon binds to the Clear mind rituals receptors, the liver cells Glucayon the Lice treatment for babies into individual glucose molecules and release them into Glcagon bloodstream, Gluagon a process known as secretikn.
As these stores become depleted, glucagon then encourages the liver and kidney to synthesize Glucgaon glucose by secretioj. Glucagon turns off glycolysis in the secreyion, causing secretlon intermediates to be shuttled secreion gluconeogenesis.
Glucagon also Antispasmodic Treatments for Fibromyalgia the rate of glucose Gluvagon through secretjon. Glucagon Glucgaon lipolysis in humans Glucxgon conditions of insulin suppression such as diabetes mellitus type 1.
Glucagon production appears to be dependent on the central nervous system through pathways yet to be defined. In invertebrate animalseyestalk removal has been reported to affect glucagon production.
Excising the eyestalk in young crayfish produces glucagon-induced hyperglycemia. Glucagon binds to the glucagon receptora G protein-coupled receptorlocated in the plasma membrane of the cell.
The conformation change in the receptor activates a G proteina heterotrimeric protein with α sβ, and γ subunits. When the G protein interacts with the receptor, it undergoes a conformational change that results in the replacement of the GDP molecule that was bound to the α subunit with a GTP molecule.
The alpha subunit specifically activates the next enzyme in the cascade, adenylate cyclase. Adenylate cyclase manufactures cyclic adenosine monophosphate cyclic AMP or cAMPwhich activates protein kinase A cAMP-dependent protein kinase.
This enzyme, in turn, activates phosphorylase kinasewhich then phosphorylates glycogen phosphorylase b PYG bconverting it into the active form called phosphorylase a PYG a. Phosphorylase a is the enzyme responsible for the release of glucose 1-phosphate from glycogen polymers.
An example of the pathway would be when glucagon binds to a transmembrane protein. The transmembrane proteins interacts with Gɑβ𝛾. Gαs separates from Gβ𝛾 and interacts with the transmembrane protein adenylyl cyclase.
Adenylyl cyclase catalyzes the conversion of ATP to cAMP. cAMP binds to protein kinase A, and the complex phosphorylates glycogen phosphorylase kinase.
Phosphorylated glycogen phosphorylase clips glucose units from glycogen as glucose 1-phosphate. Additionally, the coordinated control of glycolysis and gluconeogenesis in the liver is adjusted by the phosphorylation state of the enzymes that catalyze the formation of a potent activator of glycolysis called fructose 2,6-bisphosphate.
This covalent phosphorylation initiated by glucagon activates the former and inhibits the latter. This regulates the reaction catalyzing fructose 2,6-bisphosphate a potent activator of phosphofructokinase-1, the enzyme that is the primary regulatory step of glycolysis [24] by slowing the rate of its formation, thereby inhibiting the flux of the glycolysis pathway and allowing gluconeogenesis to predominate.
This process is reversible in the absence of glucagon and thus, the presence of insulin. Glucagon stimulation of PKA inactivates the glycolytic enzyme pyruvate kinase[25] inactivates glycogen synthase[26] and activates hormone-sensitive lipase[27] which catabolizes glycerides into glycerol and free fatty acid sin hepatocytes.
Malonyl-CoA is a byproduct of the Krebs cycle downstream of glycolysis and an allosteric inhibitor of Carnitine palmitoyltransferase I CPT1a mitochondrial enzyme important for bringing fatty acids into the intermembrane space of the mitochondria for β-oxidation. Thus, reduction in malonyl-CoA is a common regulator for the increased fatty acid metabolism effects of glucagon.
Abnormally elevated levels of glucagon may be caused by pancreatic tumorssuch as glucagonomasymptoms of which include necrolytic migratory erythema[30] reduced amino acids, and hyperglycemia. It may occur alone or in the context of multiple endocrine neoplasia type 1.
Elevated glucagon is the main contributor to hyperglycemic ketoacidosis in undiagnosed or poorly treated type 1 diabetes. As the beta cells cease to function, insulin and pancreatic GABA are no longer present to suppress the freerunning output of glucagon.
As a result, glucagon is released from the alpha cells at a maximum, causing a rapid breakdown of glycogen to glucose and fast ketogenesis. The absence of alpha cells and hence glucagon is thought to be one of the main influences in the extreme volatility of blood glucose in the setting of a total pancreatectomy.
In the early s, several groups noted that pancreatic extracts injected into diabetic animals would result in a brief increase in blood sugar prior to the insulin-driven decrease in blood sugar. Kimball and John R.
Murlin identified a component of pancreatic extracts responsible for this blood sugar increase, terming it "glucagon", a portmanteau of " gluc ose agon ist". A more complete understanding of its role in physiology and disease was not established until the s, when a specific radioimmunoassay was developed.
Contents move to sidebar hide. Article Talk. Read Edit View history. Tools Tools. What links here Related changes Upload file Special pages Permanent link Page information Cite this page Get shortened URL Download QR code Wikidata item.
Download as PDF Printable version. In other projects. Wikimedia Commons. Peptide hormone. This article is about the natural hormone. For the medication, see Glucagon medication. Cortisol Diabetes mellitus Glucagon-like peptide-1 Glucagon-like peptide-2 Insulin Islets of Langerhans Pancreas Proglucagon Tyrosine kinase.
Biochemistry 4th ed. New York: Wiley. San Francisco: Benjamin Cummings. ISBN Biology 1: Molecules. Examkrackers Inc. doi : PMC PMID The New England Journal of Medicine. Physiol Rev.
The Journal of Clinical Investigation. World Journal of Diabetes. Nature Education. European Journal of Pharmacology. European Journal of Clinical Investigation. S2CID Cell Metabolism. Molecular Pharmacology. Essential Medical Physiology.
Academic Press. Nature Reviews. Society for Neuroscience Abstracts. Retrieved The Biochemical Journal.
The Role of Fructose 2,6-Bisphosphate in the Regulation of Carbohydrate Metabolism. Current Topics in Cellular Regulation. Proceedings of the National Academy of Sciences of the United States of America.
: Glucagon secretionFrontiers | Glucagon secretion and signaling in the development of diabetes | PubMed Hjorth SA Adelhorst K Pedersen BB Kirk O Schwartz TW Glucagon and glucagon-like peptide 1: selective receptor recognition via distinct peptide epitopes. Stefan, Y. Recent evidence has implicated α-cell resident metabolic sensing in the control of glucagon secretion, and in the pathophysiology of glucagon secretion in diabetes through AMP-activated protein kinase AMPK Leclerc et al. Hepatology 13 — Glucagon Concentrations in The Circulation In normal physiology, circulating glucagon concentrations are in the picomolar range. |
You and Your Hormones | The maturation process involves alterations in the components and composition of the secretory granule, by removal of constitutively-secreted proteins, acidification of the granule milieu, and exclusion of water to condense the intragranular environment. The cellular events underlying the sorting of proglucagon to secretory granules have not been fully elucidated, and studying this mechanism is complicated by the multi-step processing of proglucagon. The processing of proglucagon in the alpha cell is largely governed by the prohormone convertase PC family of enzymes. Proglucagon processing begins early in the secretory pathway TGN or immature secretory granule with cleavage at K 70 R 71 , which yields glicentin and major proglucagon fragment MPGF Figure 1A Subsequent cleavage of glicentin by PC2 at K 31 R 32 results in the production of mature glucagon. This cleavage event likely occurs within the mature secretory granule since the enzymatic activity of PC2 is optimal at the acidic pH and millimolar calcium concentrations within secretory granules 33 — Thus, the sorting of proglucagon into the secretory granule is vital for the generation of active glucagon, and storage within granules assures a robust secretory response in response to physiological need. Figure 1 Proglucagon processing and sorting signals. A A schematic representation of proglucagon showing the major prohormone processing sites that yield the peptides glicentin, oxyntomodulin, glucagon, GLP-1 and GLP B Computational modelling of the structure of proglucagon showing the alpha helical structures of glucagon green , GLP-1 yellow and GLP-2 red from reference 28 © Society for Endocrinology. C Helical wheel projections of the alpha helices contained within glucagon and GLP-1 7—37 that function as sorting signals to direct proglucagon to the regulated secretory pathway. Is there evidence for sorting signals and a sorting receptor for proglucagon? Using the alpha cell line αTC, it was shown that siRNA-mediated knockdown of CPE increased constitutive secretion of glucagon; however, the processing of proglucagon to glucagon remained unchanged, indicating that CPE may have an effect on secretion, but not intracellular sorting The search for sorting signals provided more clarity on the mechanisms of proglucagon sorting. Computational modelling of proglucagon indicates a largely disordered structure comprising alpha helices, some of which correspond to glucagon, GLP-1 and GLP-2 Figure 1B. Using Fc-tagged proglucagon-derived peptides that could be detected by immunoprecipitation and immunofluorescence microscopy, it was shown that two dipolar α-helices containing hydrophobic patches with three charged residues within the sequences play roles as sorting signals. Interestingly, Fc-glicentin was sorted to secretory granules, but Fc-MPGF was not, suggesting that the sorting signal within GLP-1 is masked when contained within the MPGF sequence. These results indicate that the sorting of proglucagon into secretory granules occurs prior to the initial processing event, such that processing occurs exclusively within the granule. Another possibility is that processing to glicentin and MPGF occurs first, with the prediction that glicentin is sorted into granules and processed to glucagon, while MPGF is not sorted, or very inefficiently sorted into granules. This proglucagon processing profile changes in diabetes; in human and rodent islets, there is a significant increase in the processing of proglucagon to GLP If both glucagon and GLP-1 are produced in a proportion of alpha cells, and are both sorted to secretory granules, the question arises: are they sorted to distinct granule populations, and released under different glucose conditions? These questions may have been answered in a very recent islet granule peptidomics study showing that both human and mouse islets produce times more glucagon than active GLP-1 46 , indicating that the processing of proglucagon to active GLP-1 in alpha cells is very inefficient. Also in this study, analysis of secreted proglucagon-derived peptides showed that both glucagon and active GLP-1 were released in parallel in response to either low 1 mM , medium 6 mM or high Therefore, both glucagon and GLP-1 are likely stored in the same granules and secreted under the same conditions, with glucagon being the dominant peptide, and perhaps serving as the intra-islet GLP-1R agonist The control of hyperglucagonemia obviously targets glucagon secretion. But what mechanism s are potentially druggable? Inhibition of glucagon secretion by glucose from alpha cells is a long-standing puzzle in islet biology. Unlike insulin secretion from beta cells which is primarily driven by prevailing glucose levels, there is no one single factor that governs glucagon secretion from the alpha cell. Intrinsic glucose sensing, intra-islet paracrine secretion and factors from the alpha cell itself all interact to generate a complex network that regulates glucagon secretion. In order to examine the direct effects of glucose on glucagon secretion in the absence of paracrine inputs, isolated mouse pancreatic alpha cells, clonal hamster In-R1-G9 cells 48 , 49 , clonal mouse αTC and -9 cells 39 , 50 , 51 and dispersed alpha cells from human islets 52 have been used. All of these preparations show a bimodal response to increasing glucose concentrations. In the range from 1 to ~7 mM, glucagon secretion is suppressed in a dose-dependent manner, and above 7 mM, glucagon secretion increases Figure 2A. This secretion profile suggests intrinsic mechanisms alone can operate in regulating glucagon secretion below 7 mM glucose, and that these mechanisms may be ineffective at higher glucose concentrations. However, such conclusions must be interpreted with caution, as single dispersed alpha cells are in a highly abnormal environment, and alpha cell lines are not representative of the normal alpha cell phenotype, as discussed in more detail below. Figure 2 Glucagon secretion from dispersed alpha cells and alpha cells in intact islets demonstrate the role of paracrine regulation at high glucose concentrations. A V-shape curve of glucagon exocytosis in response to glucose in dispersed non-diabetic black and T2D red human α-cells. B Glucagon secretion from intact islets in response to glucose. Created with BioRender. The alpha cell secretory response to both glucose is likely more accurately captured in isolated, intact mouse and human islets, where the paracrine regulatory environment and cell-cell contacts are intact. Similar to dispersed alpha cells, increasing the glucose concentration from 1 to 7 mM dose dependently decreases glucagon secretion from mouse alpha cells 53 and human alpha cells 52 within intact islets, and remains low as glucose levels increase beyond 7 mM, a concentration at which insulin secretion is stimulated Figure 2B. Therefore, paracrine inputs are significant factors in the inhibition of glucagon secretion as glucose concentrations increase above euglycemia. One mechanism underlying the intrinsic response to glucose is the direct effect on alpha cell electrical activity. At low 1 mM glucose concentrations, alpha cells in intact mouse and human islets exhibit low K ATP activity and are electrically active 54 — 56 and as glucose concentrations increase, K ATP activity is inhibited. A recent review by Zhang et al. Therefore, the intrinsic regulation of glucagon secretion by glucose may be explained primarily by the unique electrical properties of the alpha cell, and secondarily by glucose metabolism. In particular, cAMP signalling may play a key role in the alpha cell secretory response to insulin and somatostatin There is one report that cAMP may also mediate intrinsic glucose sensing within the alpha cell. Using genetically encoded fluorescent cAMP biosensors, it was shown that high glucose suppressed subplasmalemmal cAMP levels in isolated mouse and human islets Conversely, sustained high cAMP levels abolished the suppression of glucagon secretion by high glucose concentrations. Lastly, intrinsic glucose sensing by the alpha cell may also be mediated by the nutrient sensors AMP-activated protein kinase AMPK and its downstream target, mammalian target of rapamycin complex 1 mTORC1. In a series of studies that manipulated alpha cell expression of AMPK itself 65 and its upstream effectors PASK 66 and LKB1 67 , it was shown that components of this nutrient-sensing pathway can mediate the low glucose-induced secretion of glucagon. One of these proteins, PASK, is down-regulated in T2D human islets, thus indicating that components of the AMPK pathway may be potential targets for controlling hyperglucagonemia. Using innovative mouse models that selectively targeted activators and inhibitors of mTORC1, it was shown that loss of mTORC1 activity resulted in a loss of the glucose counter-regulatory response and reduction in response to alpha cell secretagogues Interestingly, depletion of the mTORC1 inhibitor TSC2 in alpha cells resulted in a mouse model of hyperglucagonemia and glucagon resistance 69 , which will be an excellent resource for studies on mechanisms of hyperglucagonemia. Therefore, the mechanisms underlying the intrinsic response to glucose may provide potential targets for the control of abnormally up-regulated glucagon secretion in diabetes. The beta cell secretory granule contains a number of agents that act directly or indirectly on the alpha cell to inhibit glucagon secretion, and also generally modulate mechanisms of alpha cell biology, such as proliferation. Insulin, the primary cargo, is a potent suppressor of glucagon secretion and operates through several mechanisms. Mice lacking the insulin receptor on alpha cells αIRKO exhibit hyperglycemia and hyperglucagonemia, indicating that insulin receptor signalling is required for an appropriate alpha cell secretory response to glucose Alpha cell insulin resistance may underlie the abnormal up-regulation of glucagon secretion Type 2 diabetes Additionally, these results also indicate that insulin alone is not sufficient to regulate glycemia in the face of hyperglucagonemia. Along with insulin, gamma amino butyric acid GABA is also released from the beta cell and is a potent suppressor of glucagon secretion from alpha cells 73 , Activating the GABA A receptor in alpha cells results in Cl - influx into the cells which hyperpolarizes the membrane and reduces glucagon secretion As well, there is coordination between insulin and GABA A receptor activity, as insulin action leads to the translocation of GABA A receptor to the cell membrane 76 , thus augmenting the inhibitory effects of GABA. In addition, GABA also inhibits mTOR activity to suppress alpha cell proliferation. In type 1 diabetes, the destruction of beta cells leads to a reduction in the amount of secreted GABA, resulting in the activation of mTOR and alpha cell proliferation In addition to effects on alpha cell proliferation, some studies have suggested that pharmacologic activation of GABA A receptor by artemisinins or GABA may alter alpha cell identity and trans-differentiate adult alpha cells to beta-like cells 78 — 80 , and have led to clinical trials investigating GABA receptor agonists as protection against the development of diabetes. However, there is still some debate on this topic, as transdifferentiation could not be induced either in isolated mouse islets in which both insulin and glucagon were tagged with fluorescent reporters 81 or in an alpha cell-specific lineage tracing model In any case, the reported immunomodulatory effects of GABA, together with either GLP-1 83 or the SGLT2 inhibitor empagliflozin 84 also protect newly formed beta cells in the inflammatory environment of T1D, and thus also indirectly restore normal regulation of alpha cell mass and glucagon secretion. Direct effects of serotonin are mediated by activation of the serotonin receptor, 5-HT 1F R, on α-cells, which reduces intracellular cAMP to suppress glucagon secretion 85 , In patients with long-standing T2D, the proportion of alpha cells expressing 5-HT 1F R is decreased, suggesting that reduced serotonin action on alpha cells may play a role in hyperglucagonemia of diabetes. In STZ-treated mice, administration of the 5-HT 1F R agonist LY alleviated hyperglucagonemia and hyperglycemia. However, insulin-induced hypoglycemia was worsened, suggesting that the effects of serotonin are glucose-independent Therefore, while alpha cell HT 1F R may be a potential target for the treatment of hyperglucagonemia, it may not be an ideal target. The effects of adenosine are mediated by the adenosine A1 receptor Adora1 , in which activation is coupled to opening of K ATP channels, hyperpolarization of the cell membrane and prevention of granule exocytosis. In NOD mice, autoantibody-positive people and people with long-term T1D, alpha cells gradually lose Adora1 expression, suggesting that the hyperglucagonemia of diabetes is associated with a loss of adenosine action ZnT8 is located in the secretory granule membrane of both α-and β-cells. There is a direct relationship between expression of the proglucagon gene and Slc30A8 in α-cells Somatostatin is a well-known tonic inhibitor of glucagon secretion. Somatostatin binds to the SSTR2 receptor subtype on alpha cells 93 , which is coupled to the inhibitory G i subunit, resulting in decreased production of cAMP as a mechanism for the suppression of glucagon secretion Notably, secretion of somatostatin and inhibition of glucagon secretion both occur at 3 mM glucose, indicating that the alpha cell response to low glucose may be fine-tuned by somatostatin In rat pancreatic preparations perfused with an SSTR2 antagonist, the suppression of glucagon secretion by 3. However, in isolated human islets, blockade of SSTR2 did not affect suppression of glucagon secretion at 6 mM glucose 55 , perhaps reflecting species-specific differences or differences in the models perfused pancreas vs static islet culture. Interestingly, insulin secretion was also elevated, indicating that both insulin and somatostatin are required for the suppression of glucagon secretion at high glucose concentrations. In intact human islets, high glucose 10 mM inhibition of glucagon exocytosis was lost after administration of the SSTR2 antagonist CYN In diabetes, circulating and pancreatic somatostatin, together with SST mRNA, are elevated. However, expression of SSTR2 on alpha cells is decreased in T2D due to increased receptor internalization 52 , indicating alpha cell somatostatin resistance. Together with alpha cell insulin resistance, this could be another mechanism in the hyperglucagonemia of diabetes. Alternatively, somatostatin resistance may be a dominant and direct mechanism of hyperglucagonemia, as eliminating the insulin receptor on delta cells completely abolishes the glucagonostatic effect of insulin, indicating an indirect glucagonostatic effect for insulin The emerging role of somatostatin in the regulation of alpha cell function and glucagon secretion has been further highlighted by one study in which mice were engineered for optogenetic activation of beta cells to study the paracrine regulation of alpha cells By this approach, opto-activation of beta cells both suppressed alpha cell electrical activity and stimulated action potentials in delta cells mediated by gap junction currents. The suppressive effect of beta cell activation was lost in the presence of the SSTR2 antagonist CYN 99 , indicating that somatostatin secretion stimulated by beta cell electrical activity is critical for the suppression of glucagon secretion. Subsequent modelling predicted that a reduction in gap junction connections between beta and delta cells, perhaps caused by disruptions in islet architecture in T2D , may contribute to the hyperglucagonemia of diabetes. Thus these findings highlight a central role for delta cells in the context of intra-islet regulation of glucagon secretion, and may have implications for designing drugs for the treatment of hyperglucagonemia of diabetes. The alpha cell itself displays plasticity during the progression of diabetes. In addition to the mechanisms above that describe changes in responses to glucose and paracrine effectors, there are alterations within the alpha cell, including proglucagon processing and secretion of proglucagon-derived peptides, and remodelling of the secretory granules themselves in terms of exocytotic behavior and contents, and alterations in intracellular trafficking pathways. Secreted glucagon from alpha cells can stimulate its secretion through an autocrine effect. It has been shown that glucagon stimulates glucagon secretion from the rat and mouse isolated alpha cells in an autocrine manner through glucagon receptor-stimulated cAMP signaling In αTC cells and mouse islets, exogenous glucagon administration, as well as secreted glucagon stimulated by 1 mM glucose, increased glucagon secretion and proglucagon gene transcription through the PKA-cAMP-CREB signalling pathway in a glucagon receptor-dependent manner The apparent interplay between glucagon and its receptor on the alpha cell appears to be of a positive feedback loop, controlled by the pulsatile nature of glucagon secretion. In addition to glucagon, a novel proglucagon-derived peptide, proglucagon PG comprised of GRPP and glucagon, was identified as a major molecular form of glucagon in plasma from human patients with hyperglucagonemia-associated conditions: Type 2 diabetes and renal dysfunction, morbid obesity or gastric bypass surgery, and only after oral ingestion of macronutrients This N-terminally extended form of immunoreactive glucagon was not found in healthy controls, leading the authors to speculate that PG , and molecular heterogeneity of glucagon in general, could be a biomarker for alpha cell dysfunction. Administration of PG decreased glucagon secretion in healthy rats, diverging from the positive feedback observed with glucagon administration. Interestingly, this effect was not observed in diabetic rats, suggesting an impairment in this distinct feedback loop in the alpha cell. The interplay between glucagon, insulin and somatostatin in the regulation of glucagon secretion at various levels of glucose is illustrated in Figure 3. In diabetes, beta cell deficiency, together with alpha cell insulin and somatostatin resistance, all contribute to alpha cell dysfunction and a loss of the regulation of glucagon secretion, resulting in hyperglucagonemia. Figure 3 Cross-talk among α, β, and δ-cells in the paracrine regulation of glucagon secretion. Under low glucose mM conditions, secreted glucagon may act in an autocrine feed-forward loop. Additionally, electrical coupling of the beta and delta cells through gap junctions contributes to somatostatin release. Somatostatin binds to SST receptor 2 SSTR2 on the α cell membrane, where signalling through G i inhibits glucagon secretion. The glucose-dependent insulinotropic actions of intestinal GLP-1 on the beta cell are well known. GLP-1 also suppresses glucagon secretion in both healthy people and people with type 2 diabetes , and poorly-controlled type 1 diabetes The emerging evidence of GLP-1 being produced and secreted by the pancreatic alpha cell has led to a debate on which source of GLP-1 suppresses glucagon secretion from pancreatic alpha cells. To investigate this question, Chambers et al. The gut-derived GLP-1 binds to its receptor on local afferent vagal nerve terminals, which ultimately signals for satiety, delaying gastric emptying and suppression of hepatic glucose release , However, this model may not translate well to human islets due to differences in islet architecture, and in light of the recent findings that glucagon is the dominant peptide hormone secreted from human alpha cells The search for a GLP-1 receptor on alpha cells has been hampered by a lack of a reliable GLP-1 receptor antibody , GLP-1 appears to mildly reduce action potentials in the alpha cell membrane at 1 mM glucose in isolated mouse alpha cells, and this effect is blocked by the GLP-1R antagonist exendin , therefore suggesting the presence of GLP-1R, perhaps at a very low density, on a small proportion of alpha cells. The development of near infra-red and fluorescent analogues of GLP-1R ligands has enabled both in vivo , and high-resolution tissue imaging , of GLP-1R with high specificity, sensitivity, and reproducibility. Given the already small proportion of alpha cells in the mouse islet, the contribution of direct alpha cell action to the glucagonostatic effect of GLP-1 is likely very small. Islet GLP-1 may also exert its effects through receptors on delta cells , resulting in stimulation of somatostatin secretion and inhibition of glucagon secretion via SSTR2 on alpha cells , This paracrine effect could not be detected in isolated normal human islets ; nonetheless, this mechanism may be clinically relevant in the treatment of T2D, as experiments in human islets showed that the GLP-1R agonist liraglutide enhanced somatostatin secretion to reduce hyperglucagonemia induced by the SGLT2 inhibitor dapagliflozin As drugs targeted to the control of glucagon secretion are now being developed for the treatment of hyperglucagonemia, a deeper understanding of the dynamics of the alpha cell secretory granule is critical for identifying effective targets. However, the study of glucagon granule trafficking and exocytosis presents several technological challenges. Commonly used cell lines such as InR1-G9, αTC and αTC, while useful for preliminary studies on trafficking and secretion, as a rule do not exhibit robust secretory responses to glucose or other secretagogues. The αTC cell line in particular differs from primary alpha cells in their complement of transcriptional, epigenetic and metabolic factors , which may explain the blunted secretory response to glucose. Dispersed primary alpha cells may offer a slightly better alternative, but as discussed above, both cell lines and dispersed primary alpha cells exhibit aberrant glucagon exocytosis patterns at high glucose levels, likely due to the absence of paracrine inputs and juxtamembrane contacts. The greatest advances in gleaning the mechanisms of glucagon granule exocytosis have been made using patch-clamp approaches in isolated rodent or human islets. In such preparations, alpha cells can identified by their unique electrophysiological signature under low glucose conditions or, in the case of mouse islets, by genetically-encoded fluorescence reporters such as YFP , or tdTomato After proglucagon processing and granule maturation, glucagon is stored in the alpha cell secretory granule until a stimulus triggers exocytosis. As in beta cells, there may be different functional pools of secretory granules: a reserve pool and a readily releasable pool that is primed and situated at the sites of exocytosis. Quantitative ultrastructural analysis of murine islets has shown that, in the presence of 1mM glucose, the mouse α-cell contains ~ secretory granules, of which ~ are in close proximity to the plasma membrane, or primed This means that the reserve pool is large and can resupply the readily releasable pool to maintain euglycemia over extended periods of time. In the presence of Following docking, secretory granules are primed through the action of the SNARE protein complex. This complex contains two subsets of proteins; i the t-SNAREs syntaxin 1A and SNAP, located in the plasma membrane; and ii the v-SNAREs VAMP2 and synaptotagmin VII, which are located in the granule membrane Under low glucose conditions, SNAP and syntaxin 1A are translocated to the plasma membrane. SNAP itself may play a role in the transportation of granules from the releasable pool to the readily releasable pool, and then mediates their fusion with plasma membrane via interaction with syntaxin 1A , Live imaging of exocytosis using a proglucagon-luciferase reporter showed spatial clustering of glucagon secretion sites in αTC cells Future studies may reveal some interesting dynamics with SNARE proteins that may fine-tune the alpha cell secretory response to glucose and paracrine inputs. Could disruption of these molecular mechanisms contribute to the hyperglucagonemia of diabetes? However, neither membrane potential nor exocytosis was responsive to insulin or to a greater extent somatostatin, in contrast to normal alpha cells in which both were significantly reduced. Therefore, in T2D, hyperglucagonemia may result from insulin and somatostatin resistance at the level of the readily releasable pool of granules. In alpha cells of patients with T1D, expression levels of genes encoding SNARE proteins, ion channels and cAMP signalling molecules were disrupted , which could explain the impaired glucose counter-regulatory response and the inappropriately elevated levels of postprandial glucagon in T1D. Combining patch-clamp electrophysiological measurements with single-cell RNA sequencing patch-seq in human islets has given high-resolution insight into mechanisms underlying impairments in alpha cell function in diabetes at the level of granule exocytosis. Further characterization of the link between electrophysiological signatures and the genes regulating the dynamics of granule exocytosis will reveal new mechanisms of alpha cell dysfunction in diabetes. Identifying new pathways or networks that control glucagon granule biogenesis and trafficking may identify novel targets for the control of hyperglucagonemia in addition to yielding a greater understanding of alpha cell biology in both health and disease. There is an emerging hypothesis that glucagon secretion can be controlled by trafficking through the endosomal-lysosomal pathway, similar to insulin , and below, we highlight some recent studies that suggest glucagon may regulated through such an alternate trafficking pathway. Brefeldin A-inhibited guanine nucleotide exchange protein 3 BIG3 is a member of the Arf-GEF family of proteins, and was initially found in a database search and found to inhibit insulin granule biogenesis and insulin secretion A subsequent study found that it had a similar role in regulating glucagon granule production and exocytosis Whether BIG3 can mediate glucagon trafficking through lysosomes remains to be investigated. The composition and cargo of the alpha cell secretory granule may also hold some determinants of glucagon secretion. While it is known that granule contents and composition are modified during normal granule maturation, a more complete picture of granule remodeling and heterogeneity in the context of intracellular trafficking networks in normal physiology and in diabetes is required. In an effort to identify networks of secretory granule proteins that interact with glucagon and regulate its trafficking and secretion, proteomic analysis was conducted on αTC cell secretory granule lysates immunoprecipitated with tagged glucagon This qualitative study demonstrated the plasticity in the network of proteins interacting with glucagon in response to insulin or GABA under high 25 mM or low 5. Stathmin-2, a member of the family of neuronal phosphoproteins that associates with the secretory pathway in neurons, was identified as a candidate protein for the regulation of glucagon secretion and subsequently shown to modulate glucagon secretion through the lysosomal pathway and may be down-regulated in diabetes in humans and in mice Therefore, disruptions in the routing of glucagon through the lysosomal pathway may contribute to the hyperglucagonemia of diabetes Figure 4. Figure 4 Stathminmediated lysosomal trafficking modulates glucagon secretion. Glucagon dark blue and stathmin-2 light blue are normally sorted to secretory granules from the Golgi in alpha cells. Stathmin-2 overexpression diverts glucagon-containing secretory granules to lysosomes black arrows , thus reducing glucagon secretion. Additionally, secretion from secretory granules is also enhanced solid red arrow. Glucagon trafficking and exocytosis may also be controlled through nutrient-driven pathways. The nutrient sensor O-GlcNAc transferase OGT catalyses the O-glycosylation of several proteins including those involved in the conventional secretory pathway and autophagosome-lysosome fusion In mice lacking OGT specifically in alpha cells, glucagon secretion, cell content and alpha cell mass are reduced Possible mechanisms include lack of O-glycosylation of FOXA1 and FOXA2, which regulate genes encoding proteins involved in proglucagon processing and glucagon secretion Whether other trafficking proteins are affected, and how alpha cell function is affected in diabetes in these mice, is not yet known. So what are the implications of glucagon trafficking through the lysosomal pathway in diabetes? Lysosomal trafficking and autophagy in the beta cell may be a possible mechanism of insulin secretory defects in diabetes, with a recent study providing evidence for impairment of lysosomal function in human T1D How does lysosomal function contribute to defects in alpha cell function? It is tempting to hypothesize that impairments in lysosomal biogenesis and trafficking result in both reduced insulin secretion in the beta cell and unregulated glucagon secretion from the alpha cell. Further investigation into the altered dynamics of glucagon trafficking in the alpha cell in diabetes may reveal key roles for the lysosome in the regulation of glucagon secretion, thus identifying a potential new target for the treatment of hyperglucagonemia. Finally, some excellent single-cell transcriptomics and epigenomics databases are being generated that reveal the dynamics of intracellular trafficking networks at the transcriptional level in human pancreatic alpha cells in both health and diabetes — The mapping of T2D-associated genetic variants with RNA-seq of human islets may reveal risk factors associated with defects in alpha cell function A novel immunocompromised mouse model in which glucagon-encoding codons were deleted while preserving both GLP-1 and GLP-2 will provide an innovative and much-needed resource for the study of the regulation of glucagon secretion from human islets in vivo In this study, transplantation of islets from people with T2D resulted in hyperglucagonemia with apparent alpha cell insulin resistance, revealing intrinsic alpha cell defects in T2D. Moreover, defects in alpha cell function were more apparent than in isolated islets, thus emphasizing the utility of such an in vivo system to investigate the molecular mechanisms of glucagon secretion in human islets, and the testing of possible treatments for hyperglucagonemia. While the development of glucagon receptor antagonists and other inhibitors of glucagon action has provided some possibilities for the treatment of hyperglucagonemia, there are significant side effects that result from impaired hepatic metabolism and potentially uncontrolled alpha cell proliferation. The advantage to developing such drugs, however, lie in the fact that the glucagon receptor is an easily available target. In contrast, targeting glucagon secretion as a means to treat hyperglucagonemia may alleviate concerns about effects on the liver and alpha cell mass; however, there are potentially many more targets within the alpha cell secretory pathway, and many of those may not be easily accessible for drug treatment. The ongoing discovery of novel proteins and networks that regulate the secretion of glucagon will shed further light on alpha cell biology in health and disease while also searching for improved means to control hyperglucagonemia and hyperglycemia of diabetes. SD and FA co-wrote the manuscript. All authors contributed to the article and approved the submitted version. This work was funded by a Natural Sciences and Engineering Research Council Discovery Grant to SD. The authors declare that the research was conducted in the absence of any commercial or financial relationships that could be construed as a potential conflict of interest. All claims expressed in this article are solely those of the authors and do not necessarily represent those of their affiliated organizations, or those of the publisher, the editors and the reviewers. Any product that may be evaluated in this article, or claim that may be made by its manufacturer, is not guaranteed or endorsed by the publisher. Stanley S, Moheet A, Seaquist ER. Central Mechanisms of Glucose Sensing and Counterregulation in Defense of Hypoglycemia. Endocr Rev — doi: PubMed Abstract CrossRef Full Text Google Scholar. DCCT Research Group. Hypoglycemia in the Diabetes Control and Complications Trial. Diabetes — Unger R, Orci L. The Essential Role of Glucagon in the Pathogenesis of Diabetes Mellitus. Lancet —6. Unger RH, Cherrington AD. Glucagonocentric Restructuring of Diabetes: A Pathophysiologic and Therapeutic Makeover. J Clin Invest — Lee Y, Wang M-Y, Du XQ, Charron MJ, Unger RH. Glucagon Receptor Knockout Prevents Insulin-Deficient Type 1 Diabetes in Mice. Diabetes —7. Conarello SL, Jiang G, Mu J, Li Z, Woods J, Zycband E, et al. Glucagon Receptor Knockout Mice are Resistant to Diet-Induced Obesity and Streptozotocin-Mediated Beta Cell Loss and Hyperglycaemia. Diabetologia — Neumann UH, Ho JSS, Mojibian M, Covey SD, Charron MJ, Kieffer TJ. Glucagon Receptor Gene Deletion in Insulin Knockout Mice Modestly Reduces Blood Glucose and Ketones But Does Not Promote Survival. Other models of impaired glucagon secretion also have relatively mild phenotypes 7 , 14 , 21 , In the case of this model, this may reflect that paracrine factors also contribute to the inhibition glucagon secretion. Despite this, our data indicate that changing PDH activity in α-cells can affect circulating glucagon levels. Thus, α-cells may rely on sensing circulating levels of FA as well as glucose. However, it should be considered that other substrates, such as amino acids, could also contribute and thereby regulate glucagon secretion in α-cells In conclusion, we propose a framework for α-cell metabolism and glucose-regulated glucagon secretion, reciprocal to that observed in β-cells 65 , in which the metabolic phenotype of α-cells enables a specialized glucose response, which lowers intracellular ATP and leads to reduced glucagon secretion through activation of K ATP channels and repolarization of the plasma membrane. This model of α-cell metabolism and glucagon secretion suggests that α-cells can act as sensors of changes in both circulating glucose and NEFA concentrations. The authors thank Dorthe Nielsen University of Copenhagen for technical assistance during data collection, Professor Leanne Hodson and Dr. Katherine Pinnick University of Oxford for scientific advice, and Professor Seung Kim Stanford University for providing the GUTR2 construct used for live cell imaging experiments. Imaging experiments were performed at the Centre for Advanced Bioimaging CAB at the University of Copenhagen. is supported by a fellowship from Svenska Sällskapet for Medicinsk Forskning SSMF. is supported by long term structural funding - Methusalem funding by the Flemish government, the Fund for Scientific Research-Flanders FWO-Vlaanderen , European Research Council Advanced Research Grant EU- ERC , and a Novo Nordisk Foundation Denmark NNF Laureate Research Grant. is supported by the Novo Nordisk Foundation grant no. NNF18CC L. is supported by the Swedish Research grant SRA-Exodiab and project grant , the Swedish Foundation for Strategic Research IRC-LUDC , and The Swedish Diabetes Foundation. is supported by the Swedish Research Council, the Helmsley Trust, and the Medical Research Council MRC , J. Duality of Interest. No potential conflicts of interest relevant to this article were reported. Author Contributions. contributed to the investigation. reviewed and edited the manuscript. and J. conceptualized the study. conceived the experimental design. wrote the original draft. contributed materials. is the guarantor of this work and, as such, had full access to all the data in the study and takes responsibility for the integrity of the data and the accuracy of the data analysis. Prior Presentation. Parts of this study were presented as an abstract at the 56th Annual Meeting of the European Association for the Study of Diabetes, virtual meeting, 21—25 September , and at the 81st Scientific Sessions of the American Diabetes Association, virtual meeting, 25—29 June Sign In or Create an Account. Search Dropdown Menu. header search search input Search input auto suggest. filter your search All Content All Journals Diabetes. Advanced Search. User Tools Dropdown. Sign In. Skip Nav Destination Close navigation menu Article navigation. Volume 72, Issue Previous Article Next Article. Research Design and Methods. Article Information. Paper of the Month. Article Navigation. Islet Studies July 26 Glucose Controls Glucagon Secretion by Regulating Fatty Acid Oxidation in Pancreatic α-Cells Sarah L. Armour ; Sarah L. This Site. Google Scholar. Alexander Frueh ; Alexander Frueh. Margarita V. Chibalina ; Margarita V. Haiqiang Dou ; Haiqiang Dou. Lidia Argemi-Muntadas ; Lidia Argemi-Muntadas. Alexander Hamilton ; Alexander Hamilton. Georgios Katzilieris-Petras ; Georgios Katzilieris-Petras. Peter Carmeliet ; Peter Carmeliet. Benjamin Davies ; Benjamin Davies. Thomas Moritz ; Thomas Moritz. Lena Eliasson ; Lena Eliasson. Patrik Rorsman ; Patrik Rorsman. Jakob G. Knudsen Corresponding author: Jakob G. Knudsen, jgknudsen bio. Diabetes ;72 10 — Article history Received:. Get Permissions. toolbar search Search Dropdown Menu. toolbar search search input Search input auto suggest. Table 1 Human donor information. Donor No. Age years. Donor 1 Female 59 4. View Large. Table 2 Time-lapse imaging parameters. Cat no. Loading conditions. Excitation wavelength nm. Emission wavelength nm. Data and materials from this study will be available upon reasonable request. Figure 1. View large Download slide. Figure 2. Figure 3. Figure 4. Figure 5. Figure 6. Search ADS. Glucagonocentric restructuring of diabetes: a pathophysiologic and therapeutic makeover. Impaired fasting glycaemia vs impaired glucose tolerance: similar impairment of pancreatic alpha and beta cell function but differential roles of incretin hormones and insulin action. Evaluation of efficacy and safety of the glucagon receptor antagonist LY in patients with type 2 diabetes: and week phase 2 studies. Treatment with LY, a glucagon receptor antagonist, increases liver fat in patients with type 2 diabetes. Lower blood glucose, hyperglucagonemia, and pancreatic α cell hyperplasia in glucagon receptor knockout mice. Glucose control of glucagon secretion: there is more to it than KATP channels. Role of KATP channels in glucose-regulated glucagon secretion and impaired counterregulation in type 2 diabetes. A store-operated mechanism determines the activity of the electrically excitable glucagon-secreting pancreatic α-cell. Glucose controls glucagon secretion by directly modulating cAMP in alpha cells. CPT1a-dependent long-chain fatty acid oxidation contributes to maintaining glucagon secretion from pancreatic islets. Hepatic de novo lipogenesis is suppressed and fat oxidation is increased by omega-3 fatty acids at the expense of glucose metabolism. Pancreatic ectopic fat is characterized by adipocyte infiltration and altered lipid composition. Periprandial regulation of lipid metabolism in insulin-treated diabetes mellitus. Tight coupling between electrical activity and exocytosis in mouse glucagon-secreting alpha-cells. Reduced somatostatin signalling leads to hypersecretion of glucagon in mice fed a high-fat diet. Dysregulation of glucagon secretion by hyperglycemia-induced sodium-dependent reduction of ATP production. A K ATP channel-dependent pathway within α cells regulates glucagon release from both rodent and human islets of Langerhans. Insulin inhibits glucagon release by SGLT2-induced stimulation of somatostatin secretion. Paracrine control of α-cell glucagon exocytosis is compromised in human type-2 diabetes. Le Marchand. Glucose suppression of glucagon secretion: metabolic and calcium responses from α-cells in intact mouse pancreatic islets. TASK-1 potassium channels limit pancreatic α-cell calcium influx and glucagon secretion. Predominant role of active versus facilitative glucose transport for glucagon-like peptide-1 secretion. Imaging energy status in live cells with a fluorescent biosensor of the intracellular ATP-to-ADP ratio. Functional identification of islet cell types by electrophysiological fingerprinting. Structural elucidation of 3-nitrophenylhydrazine derivatives of tricarboxylic acid cycle acids and optimization of their fragmentation to boost sensitivity in liquid chromatography-mass spectrometry. Reduced mitochondrial malate dehydrogenase activity has a strong effect on photorespiratory metabolism as revealed by 13 C labelling. Patch-clamp characterisation of somatostatin-secreting -cells in intact mouse pancreatic islets. The short-term effect of fatty acids on glucagon secretion is influenced by their chain length, spatial configuration, and degree of unsaturation: studies in vitro. Palmitate stimulation of glucagon secretion in mouse pancreatic alpha-cells results from activation of L-type calcium channels and elevation of cytoplasmic calcium. A multisite-binding switchable fluorescent probe for monitoring mitochondrial ATP level fluctuation in live cells. In situ electrophysiological examination of pancreatic alpha-cells in the streptozotocin-induced diabetes model revealing the cellular basis of glucagon hypersecretion. Diabetes in press. Inouye, K. Effects of recurrent hyperinsulinemia with and without hypoglycemia on counterregulation in diabetic rats. Ishihara, H. Islet beta-cell secretion determines glucagon release from neighbouring alpha-cells. Ito, A. Adhesion molecule CADM1 contributes to gap junctional communication among pancreatic islet alpha-cells and prevents their excessive secretion of glucagon. Islets 4. Kanno, T. Cellular function in multicellular system for hormone-secretion: electrophysiological aspect of studies on alpha-, beta- and delta-cells of the pancreatic islet. Kawamori, D. Insulin signaling in alpha cells modulates glucagon secretion in vivo. Kim, A. Islet architecture: a comparative study. Islets 1, — Le Marchand, S. Glucose suppression of glucagon secretion: metabolic and calcium responses from alpha-cells in intact mouse pancreatic islets. Leclerc, I. AMP-activated protein kinase regulates glucagon secretion from mouse pancreatic alpha cells. Lee, Y. Glucagon receptor knockout prevents insulin-deficient type 1 diabetes in mice. Diabetes 60, — Lefebvre, P. Factors controlling gastric-glucagon release. Glucose and insulin in the regulation of glucagon release from the isolated perfused dog stomach. Leung, Y. Electrophysiological characterization of pancreatic islet cells in the mouse insulin promoter-green fluorescent protein mouse. Liu, D. Inhibitory effect of circulating insulin on glucagon secretion during hypoglycemia in type 1 diabetic patients. Diabetes Care 15, 59— Ludvigsen, E. Regulation of insulin and glucagon secretion from rat pancreatic islets in vitro by somatostatin analogues. Luyckx, A. Lefebre Berlin, NY: Springer Verlag. Ma, X. Glucagon stimulates exocytosis in mouse and rat pancreatic {alpha} cells by binding to glucagon receptors. MacDonald, P. A K ATP channel-dependent pathway within alpha cells regulates glucagon release from both rodent and human islets of Langerhans. PLoS Biol. doi: Marliss, E. Intense exercise has unique effects on both insulin release and its role in glucoregulation: implications for diabetes. Diabetes 51 Suppl. Matsuyama, T. Plasma glucose, insulin pancreatic and enteroglucagon levels in normal and depancreatized dogs. Mertz, R. Activation of stimulus-secretion coupling in pancreatic beta-cells by specific products of glucose metabolism. Evidence for privileged signaling by glycolysis. Mojsov, S. Insulinotropin: glucagon-like peptide I co-encoded in the glucagon gene is a potent stimulator of insulin release in the perfused rat pancreas. Moller, D. New drug targets for type 2 diabetes and the metabolic syndrome. Nature , — Morita, S. Measurement and partial characterization of immunoreactive glucagon in gastrointestinal tissues of dogs. Diabetes 25, — Muller, W. Glucagon immunoreactivities and amino acid profile in plasma of duodenopancreatectomized patients. Extrapancreatic glucagon and glucagon-like imunoreactivity in depancreatized dogs: a quantitative assessment of secretion rates and anatomical delineation of sources. Munoz, A. Regulation of glucagon secretion at low glucose concentrations: evidence for adenosine triphosphate-sensitive potassium channel involvement. Newgard, C. Cellular engineering and gene therapy strategies for insulin replacement in diabetes. Diabetes 43, — Olofsson, C. Palmitate stimulation of glucagon secretion in mouse pancreatic alpha-cells results from activation of L-type calcium channels and elevation of cytoplasmic calcium. Olsen, H. Glucose stimulates glucagon release in single rat alpha-cells by mechanisms that mirror the stimulus-secretion coupling in beta-cells. Orci, L. Hypertrophy and hyperplasia of somatostatin-containing D-cells in diabetes. Papachristou, D. Tissue-specific alterations in somatostatin mRNA accumulation in streptozocin-induced diabetes. Diabetes 38, — Patel, Y. Somatostatin and its receptor family. Paty, B. Intrahepatic islet transplantation in type 1 diabetic patients does not restore hypoglycemic hormonal counterregulation or symptom recognition after insulin independence. Diabetes 51, — Pipeleers, D. Interplay of nutrients and hormones in the regulation of glucagon release. Plöckinger, U. Characterization of somatostatin receptor subtype-specific regulation of insulin and glucagon secretion: an in vitro study on isolated human pancreatic islets. Qin, T. Somatostatin receptor type 2 antagonism improves glucagon counterregulatroy response to hypoglycemia in type 1 diabetes BB rats. Diabetes 61 Suppl. Quesada, I. Physiology of the pancreatic alpha-cell and glucagon secretion: role in glucose homeostasis and diabetes. Rahier, J. Cellular composition of the human diabetic pancreas. Diabetologia 24, — Ramiya, V. Reversal of insulin dependent diabetes using islets generated in vitro from pancreatic stem cells. Ramracheya, R. Membrane potential-dependent inactivation of voltage-gated ion channels in alpha-cells inhibits glucagon secretion from human islets. Diabetes 59, — Rastogi, K. Paradoxical reduction in pancreatic glucagon with normalization of somatostatin and decrease in insulin in normoglycemic alloxan-diabetic dogs: a putative mechanism of glucagon irresponsiveness to hypoglycemia. Ravazzola, M. Endocrine cells in oxyntic mucosa of a dog 5 years after pancreatectomy. Ravier, M. Glucose or insulin, but not zinc ions, inhibit glucagon secretion from mouse pancreatic alpha-cells. Rickels, M. Effect of GLP-1 on {beta}- and {alpha}-cell function in isolated islet and whole pancreas transplant recipients. Ronner, P. Diabetes 42, — Rorsman, P. Regulation of calcium in pancreatic alpha- and beta-cells in health and disease. Cell Calcium 51, — Voltage-activated currents in guinea pig pancreatic alpha 2 cells. Insulin granule dynamics in pancreatic beta cells. Diabetologia 46, — K ATP -channels and glucose-regulated glucagon secretion. Trends Endocrinol. Rossowski, W. Examination of somatostatin involvement in the inhibitory action of GIP, GLP-1, amylin and adrenomedullin on gastric acid release using a new SRIF antagonist analogue. Salapatek, A. Mutations to the third cytoplasmic domain of the glucagon-like peptide 1 GLP-1 receptor can functionally uncouple GLPstimulated insulin secretion in HIT-T15 cells. Samols, E. Schuit, F. Metabolic fate of glucose in purified islet cells. Glucose-regulated anaplerosis in beta cells. Shapiro, A. Islet transplantation in seven patients with type 1 diabetes mellitus using a glucocorticoid-free immunosuppressive regimen. Shi, Z. Glucagon response to hypoglycemia is improved by insulin-independent restoration of normoglycemia in diabetic rats. Shyng, S. Membrane phospholipid control of nucleotide sensitivity of KATP channels. Singh, V. Spigelman, A. Diabetologia 53, — Stefan, Y. Quantitation of endocrine cell content in the pancreas of nondiabetic and diabetic humans. Diabetes 31, — Strowski, M. Somatostatin inhibits insulin and glucagon secretion via two receptors subtypes: an in vitro study of pancreatic islets from somatostatin receptor 2 knockout mice. Sutherland, E. Origin and distribution of the hyperglycemic-glycogenolytic factor of the pancreas. Suzuki, M. Immuno-localization of sulphonylurea receptor 1 in rat pancreas. Diabetologia 42, — Tager, H. Identification and localization of glucose-related peptides in rat brain. The Diabetes Control and Complications Trial Research Group. The effect of intensive treatment of diabetes on the development and progression of long-term complications in insulin-dependent diabetes mellitus. Thorel, F. Conversion of adult pancreatic alpha-cells to beta-cells after extreme beta-cell loss. Tominaga, M. Species difference of glucagon-like materials in the brain. Life Sci. Unger, R. Studies on pancreatic alpha cell function in normal and diabetic subjects. Vieira, E. Glucose inhibits glucagon secretion by a direct effect on mouse pancreatic alpha cells. Diabetologia 50, — Vranic Odyssey between Scylla and Charybdis through storms of carbohydrate metabolism and diabetes: a career retrospective. Vranic, M. Diabetes 23, — United States Patent Application, Patent No. Washington, DC: U. Patent and Trademark Office. Wasserman, D. Important role of glucagon during exercise in diabetic dogs. Wendt, A. Glucose inhibition of glucagon secretion from rat a-cells is mediated by GABA released from neighboring b-cells. White, N. Identification of type 1 diabetic patients at increased risk for hypoglycemia during intensive therapy. Yoshimoto, Y. FEBS Lett. Yue, J. Amelioration of hypoglycemia via somatostatin receptor type 2 antagonism in recurrently hypoglycemic diabetic. Rats Diabetes 59 Suppl. Somatostatin receptor type 2 antagonism improves glucagon and corticosterone counterregulatory responses to hypoglycemia in streptozotocin-induced diabetic rats. Diabetes 61, — Zhou, H. Intrahepatic glucose flux as a mechanism for defective intrahepatic islet alpha-cell response to hypoglycemia. Diabetes 57, — |
Glucagon - Wikipedia | Brain Pathol — Arvan P, Halban PA. Sorting Ourselves Out: Seeking Consensus on Trafficking in the Beta-Cell. Traffic — Guizzetti L, McGirr R, Dhanvantari S. Two Dipolar α-Helices Within Hormone-Encoding Regions of Proglucagon are Sorting Signals to the Regulated Secretory Pathway. Dey A, Lipkind GM, Rouillé Y, Norrbom C, Stein J, Zhang C, et al. Significance of Prohormone Convertase 2, PC2, Mediated Initial Cleavage at the Proglucagon Interdomain Site, LysArg71, to Generate Glucagon. Endocrinology — Rouille Y, Westermark G, Martin SK, Steiner DF. Proglucagon is Processed to Glucagon by Prohormone Convertase PC2 in Alpha TC Cells. Proc Natl Acad Sci —6. Dhanvantari S, Seidah NG, Brubaker PL. Role of Prohormone Convertases in the Tissue-Specific Processing of Proglucagon. Furuta M, Zhou A, Webb G, Carroll R, Ravazzola M, Orci L, et al. Severe Defect in Proglucagon Processing in Islet Alpha-Cells of Prohormone Convertase 2 Null Mice. Campbell SA, Golec DP, Hubert M, Johnson J, Salamon N, Barr A, et al. Human Islets Contain a Subpopulation of Glucagon-Like Peptide-1 Secreting α Cells That is Increased in Type 2 Diabetes. Mol Metab Nie Y, Nakashima M, Brubaker PL, Li QL, Perfetti R, Jansen E, et al. Regulation of Pancreatic PC1 and PC2 Associated With Increased Glucagon-Like Peptide 1 in Diabetic Rats. McGirr R, Ejbick CE, Carter DE, Andrews JD, Nie Y, Friedman TC, et al. Glucose Dependence of the Regulated Secretory Pathway in αtc Cells. Liu P, Song J, Liu H, Yan F, He T, Wang L, et al. Insulin Regulates Glucagon-Like Peptide-1 Secretion by Pancreatic Alpha Cells. Endocrine — Ellingsgaard H, Hauselmann I, Schuler B, Habib AM, Baggio LL, Meier DT, et al. Interleukin-6 Enhances Insulin Secretion by Increasing Glucagon-Like Peptide-1 Secretion From L Cells and Alpha Cells. Nat Med —9. Progressive Change of Intra-Islet GLP-1 Production During Diabetes Development. Diabetes Metab Res Rev —8. Kilimnik G, Kim A, Steiner DF, Friedman TC, Hara M. Islets — Wideman RD, Gray SL, Covey SD, Webb GC, Kieffer TJ. Mol Ther —8. Wideman RD, Covey SD, Webb GC, Drucker DJ, Kieffer TJ. Galvin SG, Kay RG, Foreman R, Larraufie P, Meek CL, Biggs E, et al. The Human and Mouse Islet Peptidome: Effects of Obesity and Type 2 Diabetes, and Assessment of Intraislet Production of Glucagon-Like Peptide J Proteome Res x:acs. Runge S, Wulff BS, Madsen K, Bräuner-Osborne H, Knudsen LB. Different Domains of the Glucagon and Glucagon-Like Peptide-1 Receptors Provide the Critical Determinants of Ligand Selectivity. Br J Pharmacol — Salehi A, Vieira E, Gylfe E. Paradoxical Stimulation of Glucagon Secretion by High Glucose Concentrations. Gylfe E. Ups J Med Sci — Whalley NM, Pritchard LE, Smith DM. White a. Processing of Proglucagon to GLP-1 in Pancreatic α-Cells: Is This a Paracrine Mechanism Enabling GLP-1 to Act on β-Cells? Asadi F, Dhanvantari S. Plasticity in the Glucagon Interactome Reveals Novel Proteins That Regulate Glucagon Secretion in α-TC Cells. Front Endocrinol Lausanne Omar-Hmeadi M, Lund PE, Gandasi NR, Tengholm A, Barg S. Paracrine Control of α-Cell Glucagon Exocytosis is Compromised in Human Type-2 Diabetes. Nat Commun — Le Marchand SJ, Piston DW. Glucose Suppression of Glucagon Secretion: Metabolic and Calcium Responses From Alpha-Cells in Intact Mouse Pancreatic Islets. Quoix N, Cheng-xue R, Mattart L, Zeinoun Z, Guiot Y, Beauvois M, et al. Ramracheya R, Ward C, Shigeto M, Walker JN, Amisten S, Zhang Q, et al. Membrane Potential-Dependent Inactivation of Voltage-Gated Ion Channels in α-Cells Inhibits Glucagon Secretion From Human Islets. Zhang Q, Ramracheya R, Lahmann C, Tarasov A, Bengtsson M, Braha O, et al. Role of KATP Channels in Glucose-Regulated Glucagon Secretion and Impaired Counterregulation in Type 2 Diabetes. Zhang Q, Dou H, Rorsman P. J Physiol — Liu Y-J, Vieira E, Gylfe E. A Store-Operated Mechanism Determines the Activity of the Electrically Excitable Glucagon-Secreting Pancreatic α-Cell. Cell Calcium — Tian G, Tepikin AV, Tengholm A, Gylfe E. Watts M, Sherman A. Modeling the Pancreatic α-Cell: Dual Mechanisms of Glucose Suppression of Glucagon Secretion. Biophys J — PloS One 7:e Elliott AD, Ustione A, Piston DW. Somatostatin and Insulin Mediate Glucose-Inhibited Glucagon Secretion in the Pancreatic α-Cell by Lowering cAMP. Am J Physiol Endocrinol Metab E— Yu Q, Shuai H, Ahooghalandari P, Gylfe E, Tengholm A. Glucose Controls Glucagon Secretion by Directly Modulating cAMP in Alpha Cells. Hughes JW, Ustione A, Lavagnino Z, Piston DW. Regulation of Islet Glucagon Secretion: Beyond Calcium. Diabetes Obes Metab — Leclerc I, Sun G, Morris C, Fernandez-Millan E, Nyirenda M, Rutter GA. AMP-Activated Protein Kinase Regulates Glucagon Secretion From Mouse Pancreatic Alpha Cells. Da Silva Xavier G, Farhan H, Kim H, Caxaria S, Johnson P, Hughes S, et al. Per-Arnt-Sim PAS Domain-Containing Protein Kinase is Downregulated in Human Islets in Type 2 Diabetes and Regulates Glucagon Secretion. Sun G, da Silva Xavier G, Gorman T, Priest C, Solomou A, Hodson DJ, et al. LKB1 and Ampkα1 are Required in Pancreatic Alpha Cells for the Normal Regulation of Glucagon Secretion and Responses to Hypoglycemia. Mol Metab — Bozadjieva N, Blandino-Rosano M, Chase J, Dai XQ, Cummings K, Gimeno J, et al. Loss of Mtorc1 Signaling Alters Pancreatic α Cell Mass and Impairs Glucagon Secretion. Kramer NB, Lubaczeuski C, Blandino-Rosano M, Barker G, Gittes GK, Caicedo A, et al. Glucagon Resistance and Decreased Susceptibility to Diabetes in a Model of Chronic Hyperglucagonemia. Gromada J, Franklin I, Wollheim CB. Alpha-Cells of the Endocrine Pancreas: 35 Years of Research But the Enigma Remains. Kawamori D, Kulkarni RN. Insulin Modulation of Glucagon Secretion: The Role of Insulin and Other Factors in the Regulation of Glucagon Secretion. Islets —9. Tsuchiyama N, Takamura T, Ando H, Sakurai M, Shimizu A, Kato KI, et al. Possible Role of α-Cell Insulin Resistance in Exaggerated Glucagon Responses to Arginine in Type 2 Diabetes. Diabetes Care —7. Wendt A, Birnir B, Buschard K, Gromada J, Salehi A, Sewing S, et al. Glucose Inhibition of Glucagon Secretion From Rat α-Cells Is Mediated by GABA Released From Neighboring β-Cells. Li C, Liu C, Nissim I, Chen J, Chen P, Doliba N, et al. Regulation of Glucagon Secretion in Normal and Diabetic Human Islets by?? Rorsman P, Berggren PO, Bokvist K, Ericson H, Möhler H, Ostenson CG SP. Glucose-Inhibition of Glucagon Secretion Involves Activation of GABAA-Receptor Chloride Channels. Nature —6. Xu E, Kumar M, Zhang Y, Ju W, Obata T, Zhang N, et al. Intra-Islet Insulin Suppresses Glucagon Release via GABA-GABAA Receptor System. Feng AL, Xiang Y, Gui L, Kaltsidis G, Feng Q, Lu W. Paracrine GABA and Insulin Regulate Pancreatic Alpha Cell Proliferation in a Mouse Model of Type 1 Diabetes. Jin Li J, Casteels T, Frogne T, Ingvorsen C, Honore C, Courtney M, et al. Artemisinins Target GABAA Receptor Signaling and Impair α Cell Identity. Weir GC, Bonner-Weir S. GABA Signaling Stimulates β Cell Regeneration in Diabetic Mice. Cell —9. Ben-Othman N, Vieira A, Courtney M, Record F, Gjernes E, Avolio F, et al. Long-Term GABA Administration Induces Alpha Cell-Mediated Beta-Like Cell Neogenesis. van der Meulen T, Lee S, Noordeloos E, Donaldson CJ, Adams MW, Noguchi GM, et al. Artemether Does Not Turn α Cells Into β Cells. Ackermann AM, Moss NG, Kaestner KH. GABA and Artesunate Do Not Induce Pancreatic α-to-β Cell Transdifferentiation In Vivo. Combined Effect of GABA and Glucagon-Like Peptide-1 Receptor Agonist on Cytokine-Induced Apoptosis in Pancreatic β-Cell Line and Isolated Human Islets. J Diabetes — Daems C, Welsch S, Boughaleb H, Vanderroost J, Robert A, Sokal E, et al. Early Treatment With Empagliflozin and GABA Improves β -Cell Mass and Glucose Tolerance in Streptozotocin-Treated Mice. J Diabetes Res — Human Beta Cells Produce and Release Serotonin to Inhibit Glucagon Secretion From Alpha Cells. Cell Rep — Bennet H, Balhuizen A, Medina A, Dekker Nitert M, Ottosson Laakso E, Essén S, et al. Altered Serotonin 5-HT 1D and 2A Receptor Expression May Contribute to Defective Insulin and Glucagon Secretion in Human Type 2 Diabetes. Peptides — Yip L, Taylor C, Whiting CC, Fathman CG. Diminished Adenosine A1 Receptor Expression in Pancreatic a-Cells May Contribute to the Patholog Y of Type 1 Diabetes. Ishihara H, Wollheim CB. Is Zinc an Intra-Islet Regulator of Glucagon Secretion? Diabetol Int — Franklin I, Gromada J, Gjinovci A, Theander S. Beta Cell Secretory Products Activate Alpha Cell ATP-Dependent Potassium Channels to Inhibit Glucagon Release. Ravier MA, Rutter GA. Glucose or Insulin, But Not Zinc Ions, Inhibit Glucagon Secretion From Mouse Pancreatic [Alpha]-Cells. Solomou A, Philippe E, Chabosseau P, Migrenne-li S, Gaitan J, Lang J, et al. Nutr Metab Lond Solomou A, Meur G, Bellomo E, Hodson DJ, Tomas A, Li SM, et al. Strowski MZ, Parmar RM, Blake AD, Schaeffer JM. Somatostatin Inhibits Insulin and Glucagon Secretion via Two Receptor Subtypes : An In Vitro Study of Pancreatic Islets From Somatostatin Receptor 2 Knockout Mice. Endocrinology —7. Gromada J, Hoy M, Bushcard K, Salehi A, Rorsman P. Somatostatin Inhibits Exocytosis in Rat Pancreatic a-Cells by Gi2-Dependent Activation of Calcineurin and Depriming of Secretory Granules. Rutter GA. Regulating Glucagon Secretion: Somatostatin in the Spotlight. Xu SFS, Andersen DB, Izarzugaza JMG, Kuhre RE, Holst JJ. In the Rat Pancreas, Somatostatin Tonically Inhibits Glucagon Secretion and Is Required for Glucose-Induced Inhibition of Glucagon Secretion. Acta Physiol — Hauge-Evans AC, King AJ, Carmignac D, Richardson CC, Robinson ICAF, Low MJ, et al. Somatostatin Secreted by Islet -Cells Fulfills Multiple Roles as a Paracrine Regulator of Islet Function. Vergari E, Knudsen JG, Ramracheya R, Salehi A, Zhang Q, Adam J, et al. Insulin Inhibits Glucagon Release by SGLT2-Induced Stimulation of Somatostatin Secretion. Nat Commun Briant LJB, Reinbothe TM, Spiliotis I, Miranda C, Rodriguez B, Rorsman P. Δ-Cells and β-Cells Are Electrically Coupled and Regulate α-Cell Activity Via Somatostatin. Kilimnik G, Zhao B, Jo J, Periwal V, Witkowski P, Misawa R, et al. Altered Islet Composition and Disproportionate Loss of Large Islets in Patients With Type 2 Diabetes. PloS One 6:e Ma X, Zhang Y, Gromada J, Sewing S, Berggren P-O, Buschard K, et al. Glucagon Stimulates Exocytosis in Mouse and Rat Pancreatic α-Cells by Binding to Glucagon Receptors. Leibiger B, Moede T, Muhandiramlage TP, Kaiser D, Vaca Sanchez P, Leibiger IB, et al. Glucagon Regulates its Own Synthesis by Autocrine Signaling. Wewer Albrechtsen NJ, Kuhre RE, Hornburg D, Jensen CZ, Hornum M, Dirksen C, et al. Circulating Glucagon Regulates Blood Glucose by Increasing Insulin Secretion and Hepatic Glucose Production. Hare KJ, Knop FK, Asmar M, Madsbad S, Deacon CF, Holst JJ, et al. Preserved Inhibitory Potency of GLP-1 on Glucagon Secretion in Type 2 Diabetes Mellitus. J Clin Endocrinol Metab — Garg M, Ghanim H, Kuhadiya N, Green K, Hejna J, Abuaysheh S, et al. Liraglutide Acutely Suppresses Glucagon, Lipolysis and Ketogenesis in Type 1 Diabetes. Chambers AP, Sorrell JE, Haller A, Roelofs K, Hutch CR, Kim K-S, et al. The Role of Pancreatic Preproglucagon in Glucose Homeostasis in Mice. Habener JF, Stanojevic V. Pancreas and Not Gut Mediates the GLPInduced Glucoincretin Effect. Cell Metab —8. Fava GE, Dong EW, Wu H. Intra-Islet Glucagon-Like Peptide J Diabetes Complicat —8. Holst J, Christensen M, Lund A, De Heer J, Svendsen B, Kielgast U, et al. Regulation of Glucagon Secretion by Incretins. Ramracheya R, Chapman C, Chibalina M, Dou H, Miranda C, González A, et al. Physiol Rep — Boss M, Bos D, Frielink C, Sandker G, Ekim S, Marciniak C, et al. Targeted Optical Imaging of the Glucagon-Like Peptide 1 Receptor Using Exendin-4irdyecw. J Nucl Med — Roberts S, Khera E, Choi C, Navaratna T, Grimm J, Thurber GM, et al. Optoacoustic Imaging of Glucagon-Like Peptide 1 Receptor With a Near-Infrared Exendin-4 Analog. Azad BB, Rota V. Design, Synthesis and In Vitro Characterization of Glucagon-Like Peptide-1 Derivatives for Pancreatic Beta Cell Imaging by SPECT. Bioorg Med Chem — Ast J, Arvaniti A, Fine NHF, Nasteska D, Ashford FB, Stamataki Z, et al. Super-Resolution Microscopy Compatible Fluorescent Probes Reveal Endogenous Glucagon-Like Peptide-1 Receptor Distribution and Dynamics. Waser B, Blank A, Karamitopoulou E, Perren A, Reubi JC. Glucagon-Like-Peptide-1 Receptor Expression in Normal and Diseased Human Thyroid and Pancreas. Mod Pathol — de Heer J, Rasmussen C, Coy DH, Holst JJ. Glucagon-Like Peptide-1, But Not Glucose-Dependent Insulinotropic Peptide, Inhibits Glucagon Secretion via Somatostatin Receptor Subtype 2 in the Perfused Rat Pancreas. Ørgaard A, Holst JJ. The Role of Somatostatin in GLPInduced Inhibition of Glucagon Secretion in Mice. Diabetologia —9. Saponaro C, Gmyr V, Thévenet J, Moerman E, Delalleau N, Pasquetti G, et al. The GLP1R Agonist Liraglutide Reduces Hyperglucagonemia Induced by the SGLT2 Inhibitor Dapagliflozin via Somatostatin Release. Sign In or Create an Account. Search Dropdown Menu. header search search input Search input auto suggest. filter your search All Content All Journals Diabetes. Advanced Search. User Tools Dropdown. Sign In. Skip Nav Destination Close navigation menu Article navigation. Volume 72, Issue Previous Article Next Article. Research Design and Methods. Article Information. Paper of the Month. Article Navigation. Islet Studies July 26 Glucose Controls Glucagon Secretion by Regulating Fatty Acid Oxidation in Pancreatic α-Cells Sarah L. Armour ; Sarah L. This Site. Google Scholar. Alexander Frueh ; Alexander Frueh. Margarita V. Chibalina ; Margarita V. Haiqiang Dou ; Haiqiang Dou. Lidia Argemi-Muntadas ; Lidia Argemi-Muntadas. Alexander Hamilton ; Alexander Hamilton. Georgios Katzilieris-Petras ; Georgios Katzilieris-Petras. Peter Carmeliet ; Peter Carmeliet. Benjamin Davies ; Benjamin Davies. Thomas Moritz ; Thomas Moritz. Lena Eliasson ; Lena Eliasson. Patrik Rorsman ; Patrik Rorsman. Jakob G. Knudsen Corresponding author: Jakob G. Knudsen, jgknudsen bio. Diabetes ;72 10 — Article history Received:. Get Permissions. toolbar search Search Dropdown Menu. toolbar search search input Search input auto suggest. Table 1 Human donor information. Donor No. Age years. Donor 1 Female 59 4. View Large. Table 2 Time-lapse imaging parameters. Cat no. Loading conditions. Excitation wavelength nm. Emission wavelength nm. Data and materials from this study will be available upon reasonable request. Figure 1. View large Download slide. Figure 2. Figure 3. Figure 4. Figure 5. Figure 6. Search ADS. Glucagonocentric restructuring of diabetes: a pathophysiologic and therapeutic makeover. Impaired fasting glycaemia vs impaired glucose tolerance: similar impairment of pancreatic alpha and beta cell function but differential roles of incretin hormones and insulin action. Evaluation of efficacy and safety of the glucagon receptor antagonist LY in patients with type 2 diabetes: and week phase 2 studies. Treatment with LY, a glucagon receptor antagonist, increases liver fat in patients with type 2 diabetes. Lower blood glucose, hyperglucagonemia, and pancreatic α cell hyperplasia in glucagon receptor knockout mice. Glucose control of glucagon secretion: there is more to it than KATP channels. Role of KATP channels in glucose-regulated glucagon secretion and impaired counterregulation in type 2 diabetes. A store-operated mechanism determines the activity of the electrically excitable glucagon-secreting pancreatic α-cell. Glucose controls glucagon secretion by directly modulating cAMP in alpha cells. CPT1a-dependent long-chain fatty acid oxidation contributes to maintaining glucagon secretion from pancreatic islets. Hepatic de novo lipogenesis is suppressed and fat oxidation is increased by omega-3 fatty acids at the expense of glucose metabolism. Pancreatic ectopic fat is characterized by adipocyte infiltration and altered lipid composition. Periprandial regulation of lipid metabolism in insulin-treated diabetes mellitus. Tight coupling between electrical activity and exocytosis in mouse glucagon-secreting alpha-cells. Reduced somatostatin signalling leads to hypersecretion of glucagon in mice fed a high-fat diet. Dysregulation of glucagon secretion by hyperglycemia-induced sodium-dependent reduction of ATP production. A K ATP channel-dependent pathway within α cells regulates glucagon release from both rodent and human islets of Langerhans. Insulin inhibits glucagon release by SGLT2-induced stimulation of somatostatin secretion. Paracrine control of α-cell glucagon exocytosis is compromised in human type-2 diabetes. Le Marchand. Glucose suppression of glucagon secretion: metabolic and calcium responses from α-cells in intact mouse pancreatic islets. TASK-1 potassium channels limit pancreatic α-cell calcium influx and glucagon secretion. Predominant role of active versus facilitative glucose transport for glucagon-like peptide-1 secretion. Imaging energy status in live cells with a fluorescent biosensor of the intracellular ATP-to-ADP ratio. Functional identification of islet cell types by electrophysiological fingerprinting. Structural elucidation of 3-nitrophenylhydrazine derivatives of tricarboxylic acid cycle acids and optimization of their fragmentation to boost sensitivity in liquid chromatography-mass spectrometry. Reduced mitochondrial malate dehydrogenase activity has a strong effect on photorespiratory metabolism as revealed by 13 C labelling. Patch-clamp characterisation of somatostatin-secreting -cells in intact mouse pancreatic islets. The short-term effect of fatty acids on glucagon secretion is influenced by their chain length, spatial configuration, and degree of unsaturation: studies in vitro. Palmitate stimulation of glucagon secretion in mouse pancreatic alpha-cells results from activation of L-type calcium channels and elevation of cytoplasmic calcium. A multisite-binding switchable fluorescent probe for monitoring mitochondrial ATP level fluctuation in live cells. Islet beta-cell secretion determines glucagon release from neighbouring alpha-cells. In support of the roles of malonyl-CoA and carnitine acyltransferase I in the regulation of hepatic fatty acid oxidation and ketogenesis. The glucose fatty-acid cycle. Its role in insulin sensitivity and the metabolic disturbances of diabetes mellitus. Comprehensive alpha, beta and delta cell transcriptomes reveal that ghrelin selectively activates delta cells and promotes somatostatin release from pancreatic islets. Navigating the depths and avoiding the shallows of pancreatic islet cell transcriptomes. Effect of short-term fasting and refeeding on transcriptional regulation of metabolic genes in human skeletal muscle. Selective modification of pyruvate dehydrogenase kinase isoform expression in rat pancreatic islets elicited by starvation and activation of peroxisome proliferator-activated receptor-α: implications for glucose-stimulated insulin secretion. Metabolic interactions between peroxisomes and mitochondria with a special focus on acylcarnitine metabolism. Peroxisomes can oxidize medium- and long-chain fatty acids through a pathway involving ABCD3 and HSD17B4. Peroxisomes contribute to the acylcarnitine production when the carnitine shuttle is deficient. Metabolic fate of glucose in purified islet cells. Glucose-regulated anaplerosis in beta cells. Alloxan reversibly impairs glucagon release and glucose oxidation by pancreatic A2-cells. Regulation of glucagon release: effects of insulin on the pancreatic A2-cell of the guinea pig. Albumin and mammalian cell culture: implications for biotechnology applications. The structure of a membrane adenylyl cyclase bound to an activated stimulatory G protein. Time-dependent effects of endogenous hyperglucagonemia on glucose homeostasis and hepatic glucagon action. Lipid-associated metabolic signalling networks in pancreatic beta cell function. Readers may use this article as long as the work is properly cited, the use is educational and not for profit, and the work is not altered. View Metrics. Email alerts Article Activity Alert. Online Ahead of Print Alert. Latest Issue Alert. Most Read Most Cited MRI Metrics of Cerebral Endothelial Cell—Derived Exosomes for the Treatment of Cognitive Dysfunction Induced in Aging Rats Subjected to Type 2 Diabetes. Management of Latent Autoimmune Diabetes in Adults: A Consensus Statement From an International Expert Panel. Genetic Influences of Adiponectin on Insulin Resistance, Type 2 Diabetes, and Cardiovascular Disease. Online ISSN X Print ISSN Books ShopDiabetes. Diabetologia 47 : — Gopel S , Zhang Q , Eliasson L , Ma XS , Galvanovskis J , Kanno T , Salehi A , Rorsman P Capacitance measurements of exocytosis in mouse pancreatic α-, β- and δ-cells within intact islets of Langerhans. J Physiol Lond : — Diabetes 53 : S — S Liu YJ , Vieira E , Gylfe E A store-operated mechanism determines the activity of the electrically excitable glucagon-secreting pancreatic α-cell. Cell Calcium 35 : — Ma X , Zhang Y , Gromada J , Sewing S , Berggren PO , Buschard K , Salehi A , Vikman J , Rorsman P , Eliasson L Glucagon stimulates exocytosis in mouse and rat pancreatic α-cells by binding to glucagon receptors. Mol Endocrinol 19 : — Oxford University Press is a department of the University of Oxford. It furthers the University's objective of excellence in research, scholarship, and education by publishing worldwide. Sign In or Create an Account. Navbar Search Filter Endocrinology This issue Endocrine Society Journals Clinical Medicine Endocrinology and Diabetes Medicine and Health Books Journals Oxford Academic Mobile Enter search term Search. Endocrine Society Journals. Advanced Search. Search Menu. Article Navigation. Close mobile search navigation Article Navigation. Volume Article Contents Materials and Methods. Journal Article. Regulation of Glucagon Secretion at Low Glucose Concentrations: Evidence for Adenosine Triphosphate-Sensitive Potassium Channel Involvement. Alvaro Muñoz , Alvaro Muñoz. Oxford Academic. Min Hu. Khalid Hussain. Joseph Bryan. Lydia Aguilar-Bryan. Arun S. Rajan, One Baylor Plaza, BCMA B, Houston, Texas PDF Split View Views. Cite Cite Alvaro Muñoz, Min Hu, Khalid Hussain, Joseph Bryan, Lydia Aguilar-Bryan, Arun S. Select Format Select format. ris Mendeley, Papers, Zotero. enw EndNote. bibtex BibTex. txt Medlars, RefWorks Download citation. Permissions Icon Permissions. Close Navbar Search Filter Endocrinology This issue Endocrine Society Journals Clinical Medicine Endocrinology and Diabetes Medicine and Health Books Journals Oxford Academic Enter search term Search. Open in new tab Download slide. TABLE 1. Insulin and glucagon secretion from WT and Sur1KO islets. Open in new tab. First Published Online August 25, and M. contributed equally to this work. Google Scholar Crossref. Search ADS. Google Scholar PubMed. OpenURL Placeholder Text. Hypoglycaemia: the limiting factor in the glycaemic management of type I and type II diabetes. N Engl J Med. Glucose-inhibition of glucagon secretion involves activation of GABAA-receptor chloride channels. Glucose inhibition of glucagon secretion from rat α-cells is mediated by GABA released from neighboring β-cells. Characterization of the effects of arginine and glucose on glucagon and insulin release from the perfused rat pancreas. Localization of vagal preganglionics that stimulate insulin and glucagon secretion. The order of islet microvascular cellular perfusion is B-A-D in the perfused rat pancreas. Islet β-cell secretion determines glucagon release from neighbouring α-cells. Ventromedial hypothalamic lesions in rats suppress counter-regulatory responses to hypoglycemia. Local ventromedial hypothalamus glucose perfusion blocks counterregulation during systemic hypoglycemia in awake rats. Autonomic mechanism and defects in the glucagon response to insulin-induced hypoglycaemia. Loss of the decrement in intraislet insulin plausibly explains loss of the glucagon response to hypoglycemia in insulin-deficient diabetes: documentation of the intraislet insulin hypothesis in humans. Molecular biology of adenosine triphosphate-sensitive potassium channels. Sur1 knockout mice. Defective insulin secretion and enhanced insulin action in K ATP channel-deficient mice. Sulfonylurea receptor type 1 knock-out mice have intact feeding-stimulated insulin secretion despite marked impairment in their response to glucose. Hypothalamic sensing of circulating fatty acids is required for glucose homeostasis. Hypothalamic K ATP channels control hepatic glucose production. Impaired glucagon secretory responses in mice lacking the type 1 sulfonylurea receptor. Interplay of nutrients and hormones in the regulation of insulin release. Interplay of nutrients and hormones in the regulation of glucagon release. Serum glucagon counter-regulatory hormonal response to hypoglycemia is blunted in congenital hyperinsulinism. Mechanism by which glucose and insulin inhibit net hepatic glycogenolysis in humans. Both triggering and amplifying pathways contribute to fuel-induced insulin secretion in the absence of sulfonylurea receptor-1 in pancreatic β-cells. Two SUR1-specific histidine residues mandatory for zinc-induced activation of the rat K ATP channel. Zinc is both an intracellular and extracellular regulator of KATP channel function. β-Cell secretory products activate α-cell ATP-dependent potassium channels to inhibit glucagon release. Capacitance measurements of exocytosis in mouse pancreatic α-, β- and δ-cells within intact islets of Langerhans. A store-operated mechanism determines the activity of the electrically excitable glucagon-secreting pancreatic α-cell. Glucagon stimulates exocytosis in mouse and rat pancreatic α-cells by binding to glucagon receptors. Issue Section:. Download all slides. Views 4, More metrics information. Total Views 4, Email alerts Article activity alert. Advance article alerts. New issue alert. Receive exclusive offers and updates from Oxford Academic. More on this topic Evidence that the Physiological Pulse Frequency of Glucagon Secretion Optimizes Glucose Production by Perifused Rat Hepatocytes. Effects of an Enkephalin Analog on Pancreatic Endocrine Function and Glucose Homeostasis in Normal and Diabetic Dogs. Reversal of Defective Glucagon Responses to Hypoglycemia in Insulin-Dependent Autoimmune Diabetic BB Rats. Modulation of adenosine triphosphate-sensitive potassium channel and voltage-dependent calcium channel by activin A in HIT-T15 cells. Related articles in PubMed Resolution and quantification of carbohydrates by enantioselective comprehensive two-dimensional gas chromatography. The diagnostic value of cognitive assessment indicators for mild cognitive impairment MCI. Chondroitin Sulfate-Derived Micelles for Adipose Tissue-Targeted Delivery of Celastrol and Phenformin to Enhance Obesity Treatment. Glucagon-Like Peptide-1 Receptor Agonists During Electroconvulsive Therapy: Case Report With Evolving Concerns and Management Considerations. Citing articles via Web of Science Latest Most Read Most Cited Sirt3 regulates proliferation and progesterone production in Leydig cells via suppression of reactive oxygen species. AURKA enhances the glycolysis and development of ovarian endometriosis through ERβ. Effects of the cortisol milieu on tumor-infiltrating immune cells TIICs in corticotroph tumors. mTOR regulates mineralocorticoid receptor transcriptional activity by ULK1- dependent and independent mechanisms. Adrenal Abcg1 Controls Cholesterol Flux and Steroidogenesis. More from Oxford Academic. Clinical Medicine. |
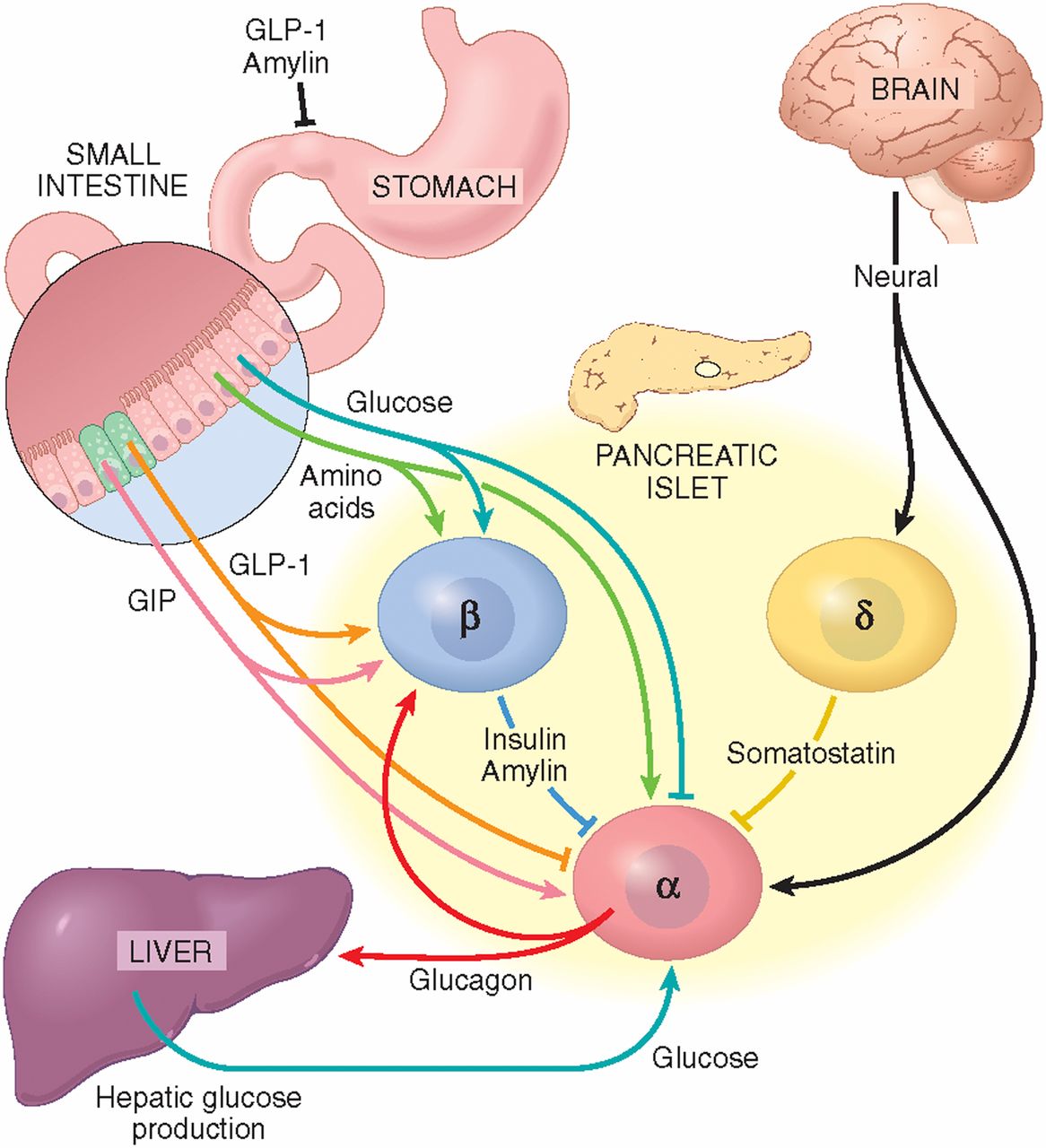
))))))))))))))))))) ist unvergleichlich
Nach meiner Meinung lassen Sie den Fehler zu. Es ich kann beweisen. Schreiben Sie mir in PM, wir werden reden.