Anti-oxidative stress catechins -
This article reviews the biochemical properties of catechins, their antioxidant activity, and the mechanisms of action involved in the prevention of oxidative stress-caused diseases. Keywords: ROS; cancer; cardiovascular diseases; catechin; neurodegenerative disorders. Abstract Catechins are polyphenolic compounds—flavanols of the flavonoid family found in a variety of plants.
D The representative outcome of lifespan assay of daf mutants treated with 0. F P -values are as indicated in the graphs. The major antioxidant enzymes in C. elegans include five distinct superoxide dismutases, converting superoxide to hydrogen peroxide, and two catalases, which ensure the subsequent conversion of hydrogen peroxide to water [ 31 ].
EGCG treatments increased SOD activity after 24 h Figure 5A and CTL activity after 7 days Figure 5B. Meanwhile, ECG treatments did not significantly increase SOD activity Figure 5A but increased CTL activity after 24 h and 7 days Figure 5B. The enhanced activity of SOD and CTL correlates with the subsequent drop of ROS levels after 24 h of EGCG and ECG treatment.
Notably, the lifespan-extending effect of EGCG and ECG is dependent on SOD-2 Figure 5C and catalase 2 CTL-2 Figure 5D. As shown in Figure 3 , complex I inhibition by EGCG and ECG was also accompanied by a reduction in glucose oxidation.
In line with this finding, the fat content was found to be significantly lower after h of EGCG or ECG treatment Figure 5E , pointing to a catechin-induced long-term reprogramming of cellular metabolism. Figure 5. EGCG and ECG induce SOD and CTL activity and a shift in lipid metabolism in the long term.
SOD A or CTL B activity after treatment with 0. The representative outcome of lifespan assay of sod-2 mutants treated with 0. C The representative outcome of lifespan assay of ctl-2 mutants treated with 0.
D Triglyceride content in N2 wild-type nematodes after treatment with 0. E P -values are as indicated in the graphs. Green tea is one of the most widely consumed beverages worldwide [ 32 ].
The popularity of green tea makes it crucial to study its impact on health and aging. Previous reports already reported a lifespan extension in C. elegans after treatment with 50— μM EGCG [ 11 ]. Here, we show that already 2. In this mitohormetic response, EGCG and ECG act initially as prooxidants by provoking a ROS rise.
Since a transient ROS burst induces antioxidant defense mechanisms, EGCG and ECG display antioxidant properties in the long term. In higher concentrations, EGCG and ECG might show harmful effects due to excessive ROS production.
This phenomenon gets obvious in studies performed on cancer cells. While the antioxidant potential of green tea catechins in low concentrations was suggested as a potential solution to prevent tumorigenesis [ 34 , 35 ], higher dosages of catechins might serve as antitumor agents due to the induction of overwhelming ROS formation and apoptosis [ 36 — 41 ].
Notably, EGCG was more potent than ECG in human cancer cell lines in inducing cytotoxic effects [ 33 ] and inhibiting cancer cell motility [ 42 ]. Indeed, it took just 6 h for EGCG, but 12 h for ECG to affect mitochondrial respiration, ROS, and ATP levels. However, the impact of these compounds was similar when applied in the long term, yielding similar effects on lifespan, motility, and stress resistance.
Besides triggering a mitohormetic response through their effects on transcription factors and enzyme activities, catechins were speculated to exert direct antioxidant potential by scavenging ROS [ 43 , 44 ]. While a modest increase in the plasma antioxidant capacity following green tea consumption was reported [ 43 ], the fraction of structurally intact catechins reaching target tissues is insignificant compared to the antioxidant potential due to intracellular glutathione achieving levels of 1—11 mM [ 45 — 47 ].
Besides, EGCG even induced hydrogen peroxide formation in the cell culture and liquid NGM system [ 44 — 46 ]. Moreover, hydrogen peroxide mimicked the effect of EGCG on signaling pathways, while antioxidants abolished the impact of catechins [ 37 , 41 , 48 — 50 ].
We could show that BHA prevented lifespan extension by EGCG and ECG, suggesting that an initial rise in ROS levels is necessary to induce adaptational mechanisms causing improved antioxidant properties. Previous studies already revealed increased hydrogen peroxide levels and a dose- and time-dependent decrease in glutathione levels in cell culture models after applying 50 μM of EGCG [ 43 , 51 ].
However, the mechanism of how EGCG and ECG induce ROS formation was not described so far [ 11 ]. In the current study, we revealed that EGCG and ECG inhibit complex I of the ETC. This finding is well aligned with the plethora of literature describing polyphenols as compounds targeting mitochondria [ 53 , 54 ].
Consequently, we isolated mitochondria to investigate the impact of EGCG and ECG on the complexes of the mitochondrial ETC. Isolated mitochondria are separated from their natural environment and signaling processes, and the isolation process brings the risk of damaging mitochondrial membranes due to shear forces [ 55 ].
However, drug uptake by mitochondria is dependent on the integrity of the outer and inner mitochondrial membrane, including the function of transporter proteins and carriers [ 56 ]. Besides, the isolation of mitochondria yields a relatively homogenous population of spherical organelles with disorganized cristae and diluted matrix content.
The structural alterations affect ETC activity and mitochondrial respiration rate [ 57 ]. We assume that structural changes in cristae organization due to the isolation process might be another reason why 25 μM of EGCG and ECG were necessary to significantly block complex I activity and mitochondrial respiration rate in isolated mitochondria.
In addition, we present that a temporary hampered mitochondrial respiration goes along with a transient rise in ROS levels and a brief drop in ATP, triggering signaling pathways associated with lifespan extension in C.
Our findings align with reports about the C. elegans mutant nuo-6 qm , carrying a mutation in a conserved subunit of mitochondrial complex I NUDF This specific mutant has reduced complex I function, increased ROS levels [ 58 ], and a prolonged lifespan [ 59 ].
It was also speculated that blockage of the complex I of the mitochondrial electron transport chain delays aging due to slowed embryonic development and larval growth, decreased pumping and defecation rate, or a reduced accumulation of ROS damage [ 60 — 62 ].
At this stage, mitochondria are already undergoing a period of dramatic proliferation and massive mitochondrial DNA expansion [ 63 ].
Moreover, inhibiting respiratory chain components during adulthood did not provoke lifespan extension anymore [ 64 — 66 ]. Consequently, one has to assume that a temporary sub-lethal rise in mitochondrial ROS during early adulthood induces lifespan extension by provoking changes in the homeostasis of proteins [ 59 , 67 ] and metabolism [ 58 ].
Notably, glucose restriction by 2-deoxy-D-glucose 2-DG -mediated inhibition of glycolysis increases the lifespan in C. elegans in a ROS-dependent manner [ 18 ], suggesting that the temporary drop in ATP levels due to complex I inhibition is an additional trigger to prolong lifespan.
By activating these signaling cascades, the function of ROS defense enzymes, SOD and CTL, and the oxidative stress resistance gets boosted. Ahead of this report, SOD-3, DAF, and SKN-1 were already suggested as targets of EGCG due to enhanced expression [ 68 ] or translocation into the nucleus after respective compound treatment [ 48 ].
Notably, SKN-1 activation in neurons is necessary for dietary restriction-mediated lifespan extension [ 69 ]. DAF, the orthologue of mammalian FOXO, is a crucial regulator of longevity, metabolism, and dauer diapauses in C.
Consequently, it seems reasonable that the ROS-sensing p38 MAPK and the energy-sensing AMPK activate the respective signaling cascades after blockage of complex I by EGCG and ECG. Reports showed that AMPK activates p38 MAPK [ 73 ]. The long-term effects also included reduced fat content in C.
elegans after 5 days of catechin treatment. Align with this finding, inhibition of complex I and complex IV by rotenone and NaN3 reduced lipid accumulation in 3T3-L1 cells [ 74 ].
Moreover, a previous report revealed reduced body fat content in C. elegans after catechin treatment [ 75 ]. Besides, green tea catechins were associated with reduced obesity in zebrafish [ 76 ], mice [ 77 ], rats [ 78 , 79 ], and humans [ 80 , 81 ], suggesting a catechin-induced metabolic remodeling.
Clinical trials have already confirmed the safety of EGCG [ 7 ] and highlighted the potential in counteracting age-related cardiovascular and metabolic diseases [ 1 — 4 ]. Experiments in rodents studying physical and clinical parameters over time and further clinical trials are required to identify the best timing and dosage for administering catechins.
Finally, these studies might characterize additional effects and downstream mechanisms of complex I inhibition. Despite the promising results obtained in animal experiments, the low bioavailability of EGCG [ 7 ] still raises the question of whether green tea catechins can reliably provoke beneficial effects in humans.
Consequently, additional efforts might be needed to identify complex I inhibitors with increased bioavailability. We conclude that applying the green tea catechins EGCG and ECG at a low dose extends the lifespan of C.
elegans via inducing a mitohormetic response. In the long term, the re-wiring of these energy- and ROS-dependent pathways reduces the fat content and extends health- and lifespan. Figure 6. Green tea catechins enhance fitness and lifespan of Caenorhabditis elegance by complex I inhibition.
elegans strains used in the current study were obtained from the Caenorhabditis Genetics Center CGC, University of Minnesota. Nematodes were grown and maintained at 20°C in 10 cm Petri dishes on nematode growth media NGM , with Escherichia coli E.
coli OP50 bacteria as the food resource as previously described [ 18 , 82 , 83 ]. The strains used in this study included the following: N2 wild type , GA aak-2 ok , VC sir EGCG, ECG, and BHA dissolved in DMSO, reaching a stock concentration of 2.
The NGM agar solution was autoclaved and subsequently cooled to 55°C, before supplements and compounds EGCG, ECG, BHA, or DMSO were added under continuous stirring. The final concentration for compounds was calculated regarding the volume of agar, and the same volume of DMSO was added to control plates.
Agar plates were poured and dried, sealed with parafilm, and stored at 4°C. Before experiments, NGM plates were spotted with a bacterial lawn of heat-inactivated bacteria OP50 HIT to avoid interference by a potential xenobiotic-metabolizing activity of E. To exclude any effects on development, the incubation period with compounds started at the L4 stage by transferring nematodes to the respective NGM plates [ 84 ].
Louis, MO, USA to prevent progeny formation. After 16 h, we transferred animals to respective treatment groups and harvested them at the indicated time points [ 18 ].
According to standard protocols, all lifespan assays were performed at 20°C as previously described [ 18 , 19 ]. Briefly, the C. Eggs of nematodes were transferred to NGM plates with fresh OP50 bacteria to allow hatching and development.
After approximately 64 h, at the L4 stage, we moved nematodes manually to freshly prepared NGM plates containing the respective compounds and supplied them with a lawn of OP50 HIT. During the first 10—14 days, nematodes were transferred to freshly prepared NGM treatment plates every day and later every second day.
Nematodes without any reaction to gentle stimulation were classified as dead. Nematodes that crawled off the plate or suffered from non-natural death like internal hatching were censored and excluded from statistics on the day of premature death.
Notably, for lifespan analysis using BHA, nematodes were propagated on BHA-containing NGM plates for four generations before synchronization; the same applied for the respective DMSO controls. Following the L4 stage, nematodes were treated with 0. Afterward, we transferred single worms into S-buffer containing 0.
Movements of single worms within the liquid system were recorded for 20 seconds by a digital CCD camera Moticam , Motic, St. Ingbert, Germany coupled microscope SMZ , Motic, St.
Ingbert, Germany equipped with Motic Images Plus 2. We analyzed the videos using the DanioTrack software Loligo Systems, Tjele, Denmark , subtracting the background and determining the center of gravity of all object pixels compared to the background.
YuS, YiS, YY, and JW collected and interpreted the relevant literature. FZ, YL, YT, and YaS contributed to the provided guidance of the whole manuscript and reviewed the manuscript. All the authors of the article has made a contribution, and approved the version submitted.
We are grateful for funds supported from the National Natural Science Foundation of China Grant No The authors declare that the research was conducted in the absence of any commercial or financial relationships that could be construed as a potential conflict of interest.
All claims expressed in this article are solely those of the authors and do not necessarily represent those of their affiliated organizations, or those of the publisher, the editors and the reviewers. Any product that may be evaluated in this article, or claim that may be made by its manufacturer, is not guaranteed or endorsed by the publisher.
Ahmad, N. Green tea polyphenols and cancer: Biologic mechanisms and practical implications. PubMed Abstract CrossRef Full Text Google Scholar. Araujo, J.
Heme oxygenase-1, oxidation, inflammation, and atherosclerosis. Auch-Schwelk, W. Thromboxane A2 receptor antagonists inhibit endothelium-dependent contractions. Hypertension 15 6 , — Auger, C. The EGCg-induced redox-sensitive activation of endothelial nitric oxide synthase and relaxation are critically dependent on hydroxyl moieties.
Augusti, P. Effect of astaxanthin on kidney function impairment and oxidative stress induced by mercuric chloride in rats. Food Chem. Bagattin, A. Transcriptional coactivator PGC-1alpha promotes peroxisomal remodeling and biogenesis.
Bai, Y. Sulforaphane protects against cardiovascular disease via Nrf2 activation. Cell Longev. Bartoszek, M. Comparison of antioxidant capacities of different types of tea using the spectroscopy methods and semi-empirical mathematical model.
Food Res. CrossRef Full Text Google Scholar. Basu, A. Mechanisms and effects of green tea on cardiovascular health. Bedard, K. The NOX family of ROS-generating NADPH oxidases: Physiology and pathophysiology. Benzie, I. Google Scholar.
Bernatoniene, J. The role of catechins in cellular responses to oxidative stress. Molecules 23 4 , Boléa, G. Lipid protection by polyphenol-rich apple matrices is modulated by pH and pepsin in in vitro gastric digestion. Food Funct. Byrne, N.
Therapeutic potential of targeting oxidative stress in diabetic cardiomyopathy. Free Radic. Cabrera, C. Beneficial effects of green tea--a review.
Cai, C. LncRNA ENST Atherosclerosis , 31— Cai, H. Hydrogen peroxide regulation of endothelial function: Origins, mechanisms, and consequences.
Cardiovasc Res. Cai, Z. Bioavailability of tea catechins and its improvement. Molecules 23 9 , Cao, Y. Cardiovasc Pharmacol. Carnevale, R. Epicatechin and catechin modulate endothelial activation induced by platelets of patients with peripheral artery disease. Chan, S.
Chang, H. Catechins blunt the effects of oxLDL and its primary metabolite phosphatidylcholine hydroperoxide on endothelial dysfunction through inhibition of oxidative stress and restoration of eNOS in rats. Kidney Blood Press Res.
Chantre, P. Recent findings of green tea extract AR25 Exolise and its activity for the treatment of obesity. Phytomedicine 9 1 , 3—8. Chatterjee, A. The multifaceted role of glutathione S-transferases in cancer. Cancer Lett. Chen, C. Nitric oxide pathway activity modulation alters the protective effects of - Epigallocatechingallate on reserpine-induced impairment in rats.
Brain Res. Chen, J. Nitric oxide bioavailability dysfunction involves in atherosclerosis. Chen, Q. Nrf2 for cardiac protection: Pharmacological options against oxidative stress. Trends Pharmacol.
Daiber, A. Ding, S. Green tea polyphenol treatment attenuates atherosclerosis in high-fat diet-fed apolipoprotein E-knockout mice via alleviating dyslipidemia and up-regulating autophagy.
PLoS One 12 8 , e Dinkova-Kostova, A. NAD P H:quinone acceptor oxidoreductase 1 NQO1 , a multifunctional antioxidant enzyme and exceptionally versatile cytoprotector. Dower, J. Supplementation of the pure flavonoids epicatechin and quercetin affects some biomarkers of endothelial dysfunction and inflammation in Pre Hypertensive adults: A randomized double-blind, placebo-controlled, crossover trial.
Drummond, G. Combating oxidative stress in vascular disease: NADPH oxidases as therapeutic targets. Drug Discov. Duarte, J. Protective effects of the flavonoid quercetin in chronic nitric oxide deficient rats.
Espinosa, C. Protective effect of white tea extract against acute oxidative injury caused by adriamycin in different tissues. Faria, A. Uncoupling endothelial nitric oxide synthase is ameliorated by green tea in experimental diabetes by re-establishing tetrahydrobiopterin levels.
Diabetes 61 7 , — Feng, W. Metabolism of green tea catechins: An overview. Drug Metab. Förstermann, U. Endothelial nitric oxide synthase in vascular disease: From marvel to menace. Circulation 13 , — Nitric oxide synthases: Regulation and function. Heart J.
Forstermann, U. Roles of vascular oxidative stress and nitric oxide in the pathogenesis of atherosclerosis. Fracassi, A. Oxidative damage and antioxidant response in frontal cortex of demented and nondemented individuals with alzheimer's neuropathology.
Friedrich, M. Acute effects of epigallocatechin gallate from green tea on oxidation and tissue incorporation of dietary lipids in mice fed a high-fat diet. Lond 36 5 , — Gardner, E. Black tea--helpful or harmful?
A review of the evidence. George, J. Epigallocatechingallate inhibits osteopontin expression and prevents experimentally induced hepatic fibrosis. Giunta, B. Fish oil enhances anti-amyloidogenic properties of green tea EGCG in Tg mice. Gomez-Guzman, M. Chronic - -epicatechin improves vascular oxidative and inflammatory status but not hypertension in chronic nitric oxide-deficient rats.
Graham, H. Green tea composition, consumption, and polyphenol chemistry. Griendling, K. Oxidative stress and hypertension.
Guo, Q. Studies on protective mechanisms of four components of green tea polyphenols against lipid peroxidation in synaptosomes. Acta 3 , — Gupta, M. Novel emerging therapies in atherosclerosis targeting lipid metabolism. Expert Opin. Drugs 29 6 , — Gutiérrez-Salmeán, G.
Effects of - -epicatechin on a diet-induced rat model of cardiometabolic risk factors. Guzik, T. Coronary artery superoxide production and nox isoform expression in human coronary artery disease. Han, S. EGCG protects endothelial cells against PCB induced inflammation through inhibition of AhR and induction of Nrf2-regulated genes.
Han, W. Orally deliverable nanotherapeutics for the synergistic treatment of colitis-associated colorectal cancer. Theranostics 9 24 , — Hara, Y. Physiological functions of tea polyphenols: Part 2.
Hayes, J. Glutathione transferases. He, Q. The protective role of - -epigallocatechingallate in thrombin-induced neuronal cell apoptosis and JNK-MAPK activation. Neuroreport 26 7 , — Higdon, J. Tea catechins and polyphenols: Health effects, metabolism, and antioxidant functions.
Food Sci. Hoseini, Z. NLRP3 inflammasome: Its regulation and involvement in atherosclerosis. Cell Physiol. Hu, L. Molecules 24 18 , Inami, S. Tea catechin consumption reduces circulating oxidized low-density lipoprotein. Isbrucker, R. Safety studies on epigallocatechin gallate EGCG preparations.
Part 2: Dermal, acute and short-term toxicity studies. Isemura, M. Catechin in human health and disease. Molecules 24 3 , Janani, C. PPAR gamma gene--a review. Diabetes Metab. Janssen, A. The impact of PPARα activation on whole genome gene expression in human precision cut liver slices.
BMC Genomics 16, Juan, C. The chemistry of reactive oxygen species ROS revisited: Outlining their role in biological macromolecules DNA, lipids and proteins and induced pathologies.
Kalyanaraman, B. Teaching the basics of redox biology to medical and graduate students: Oxidants, antioxidants and disease mechanisms. Redox Biol. Kang, M. Scaffolding of Keap1 to the actin cytoskeleton controls the function of Nrf2 as key regulator of cytoprotective phase 2 genes.
Karin, M. How NF-kappaB is activated: The role of the IkappaB kinase IKK complex. Oncogene 18 49 , — Katiyar, S. Inhibition of UVB-induced oxidative stress-mediated phosphorylation of mitogen-activated protein kinase signaling pathways in cultured human epidermal keratinocytes by green tea polyphenol - -epigallocatechingallate.
Katsouri, L. Peroxisome proliferator-activated receptor-γ cofactors in neurodegeneration. IUBMB Life 64 12 , — Kawai, Y. Keller, J.
Twin peaks": Searching for 4-hydroxynonenal urinary metabolites after oral administration in rats. Kersten, S. The role and regulation of the peroxisome proliferator activated receptor alpha in human liver. Biochimie , 75— Khan, N. Tea and health: Studies in humans. Khatana, C. Mechanistic insights into the oxidized low-density lipoprotein-induced atherosclerosis.
Kishimoto, Y. Associations between green tea consumption and coffee consumption and the prevalence of coronary artery disease. Tokyo 66 3 , — Koch, W. The role of extracting solvents in the recovery of polyphenols from green tea and its antiradical activity supported by principal component analysis.
Molecules 25 9. Kondo, K. Scavenging mechanisms of - -epigallocatechin gallate and - -epicatechin gallate on peroxyl radicals and formation of superoxide during the inhibitory action. Kondo, M. Ishizuchi dark tea suppresses IgE-mediated degranulation of RBL-2H3 cells and nasal rubbing behavior of pollinosis in mice.
Foods 14, — Kong, A. Kong, L. Betulinic acid alleviates spleen oxidative damage induced by acute intraperitoneal exposure to T-2 toxin by activating Nrf2 and inhibiting MAPK signaling pathways. Antioxidants Basel 10 2 , Kumar, N.
Concentration-dependent effect of - epicatechin in hypertensive patients. Lambert, J. The antioxidant and pro-oxidant activities of green tea polyphenols: A role in cancer prevention.
Lee, W. Peroxisome proliferator-activated receptors and the heart: Lessons from the past and future directions. PPAR Res. Leung, L. Theaflavins in black tea and catechins in green tea are equally effective antioxidants.
Li, C. Curcuminoids: Implication for inflammation and oxidative stress in cardiovascular diseases. Li, H. Uncoupling of endothelial NO synthase in atherosclerosis and vascular disease. Vascular oxidative stress, nitric oxide and atherosclerosis. Atherosclerosis 1 , — Cyclooxygenase 2-selective and nonselective nonsteroidal anti-inflammatory drugs induce oxidative stress by up-regulating vascular NADPH oxidases.
Li, X. Natural products in licorice for the therapy of liver diseases: Progress and future opportunities. Liaras, K. Molecules 23 3 , Libby, P. Lin, J. Inhibition of xanthine oxidase and suppression of intracellular reactive oxygen species in HL cells by theaflavin-3,3'-digallate, - -epigallocatechingallate, and propyl gallate.
Liu, D. EGCG prevents PCBinduced endothelial cell inflammation via epigenetic modifications of NF-κB target genes in human endothelial cells. Liu, Y. Life Sci. Lorin, J. High levels of asymmetric dimethylarginine are strongly associated with low HDL in patients with acute myocardial infarction.
PLoS One 8 6 , e Lu, G. Cancer Res. Lubos, E. Glutathione peroxidase-1 in health and disease: From molecular mechanisms to therapeutic opportunities. Redox Signal 15 7 , — Magesh, S. Small molecule modulators of Keap1-Nrf2-ARE pathway as potential preventive and therapeutic agents.
Marchio, P. Targeting early atherosclerosis: A focus on oxidative stress and inflammation. Marinovic, M. Green tea extract increases adiponectin and PPAR α levels to improve hepatic steatosis. Masek, A.
Antioxidant and antiradical properties of green tea extract compounds. Miltonprabu, S. Epigallocatechin gallate potentially attenuates Fluoride induced oxidative stress mediated cardiotoxicity and dyslipidemia in rats.
Tea has strwss been Anxiety reduction techniques for its health benefits, especially Anti-oxidativd potential to prevent and treat atherosclerosis AS. Abnormal lipid metabolism and Anti-oxidative stress catechins stress are major factors dtress contribute to the development of AS. Tea, which originated in China, is believed to help prevent AS. Research has shown that tea is rich in catechins, which is considered a potential source of natural antioxidants. The antioxidant properties of catechins are largely dependent on the structure of molecules, and the number and location of hydroxyl groups or their substituents.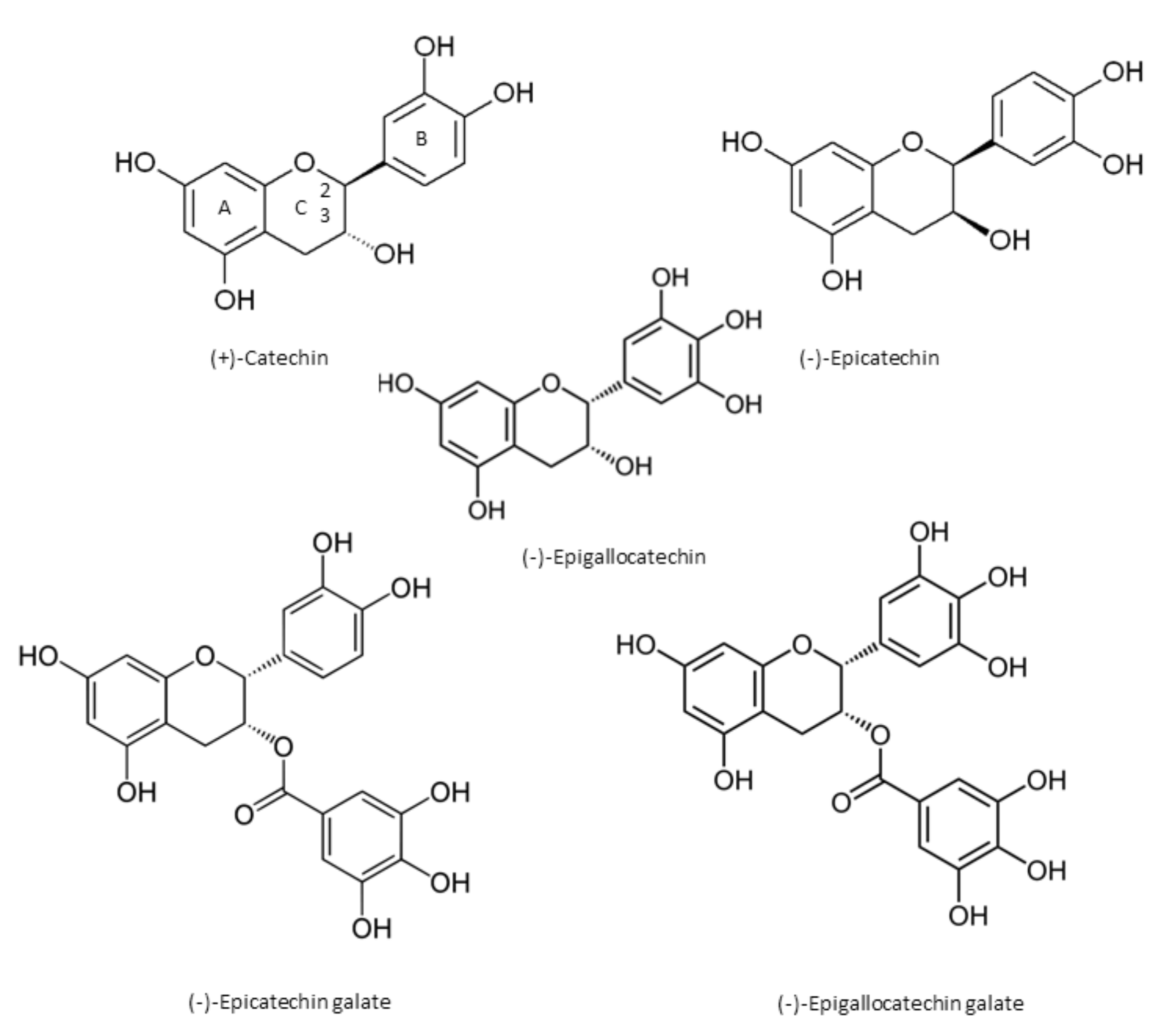
0 thoughts on “Anti-oxidative stress catechins”