Video
Regulation of Insulin Release and Insulin Action Domenico Accili; Insulin Insulin mechanism of action Research mecyanism the Future of Diabetes Treatment: The Banting Medal Team-building exercises Scientific Achievement Lecture. IInsulin 1 September ; 67 Insulin mechanism of action : Insulon Diabetes is caused by combined abnormalities actionn insulin production Insulin mechanism of action action. The Insulin mechanism of action Sports nutrition choices these mechajism has been studied mechanixm and is BCAA and muscle metabolism well understood. Their causes are elusive and their manifestations pleiotropic, likely reflecting the triple threat of genes, environment, and lifestyle. Treatment, once restricted to monotherapy with secretagogues or insulin, now involves complex combinations of expensive regimens that stem the progression but do not fundamentally alter the underlying causes of the disease. As advances in our understanding of insulin action and β-cell failure reach a critical stage, here I draw on lessons learned from our research on insulin regulation of gene expression and pancreatic β-cell dedifferentiation to address the question of how we can translate this exciting biology into mechanism-based interventions to reverse the course of diabetes.Insulin mechanism of action -
In the vasculature, Foxo regulates nitric oxide production and inflammatory responses 25 — In addition, it carries out seemingly distinct functions in tissue differentiation and lineage stability that are especially evident in pancreatic and enteroendocrine cells 15 , 28 — It does so through an elegant mechanism of phosphorylation-induced nuclear exclusion.
Thus, in the postabsorptive state, Foxo is located in the nucleus and regulates gene expression. In response to meals, it undergoes insulin-dependent phosphorylation and is transferred from the nucleus to the cytoplasm, where it lies quiescent until nutrient levels fall again 7. The key conceptual advance of this work is the extent to which the actions of insulin can be accounted for by changes in gene transcription.
Experimental animal studies show that blocking insulin action by incapacitating any of its key upstream components insulin receptor and its substrates, PIkinase, or Akt leads to Foxo activation, with ensuing insulin resistance and diabetes.
This effect can be reversed by concurrent inactivation of Foxo, reestablishing metabolic control 10 — Similar epistasis can be demonstrated to regulate fiber type composition and mass in skeletal muscle 32 , 33 , as well as β-cell mass These phenotypes are surprising to the extent that it had long been assumed that the primary actions of insulin occurred by regulating enzyme activities rather than gene expression The data should not be construed to indicate that this signaling module is redundant but simply that its local actions in any given tissue can be compensated by insulin acting indirectly through substrate fluxes from other organs Insulin integrates hepatic glucose and lipid metabolism, directing nutrients to glycolysis, glycogen storage, and lipogenesis.
The diabetic liver overflows with glucose and atherogenic lipoproteins such VLDL triglycerides and small dense LDL, resulting in lower HDL. Thus, hepatic insulin resistance can be viewed as a causal factor in both microvascular hyperglycemia-driven and macrovascular lipoprotein-driven complications of diabetes Decreasing hepatic glucose production has long been viewed as a therapeutic target in diabetes.
There appear to be at least three different modalities to regulate hepatic glucose production: 1 indirect regulation of nutrient flow from other tissues; 2 direct, Foxo-dependent actions in the hepatocyte, in part by its regulation of glucogenic enzymes glucosephosphatase G6pc and glucokinase Gck and in part through yet undefined mechanisms; and 3 direct, Foxo-independent actions I will not attempt to summarize, let alone reconcile, the many different opinions on these phenomena.
No animal model can be said to fully recapitulate the human pathophysiology, in part because the latter is likely comprised of several distinct subphenotypes whose relative pathophysiologic contribution changes over the course of the disease.
Which experimental lessons can be generalized and contribute to new approaches to treatment? First, suppression of adipocyte lipolysis by insulin is required to suppress hepatic glucose production in humans as well as rodents. Whether central nervous system—derived signals are also important in this process remains unclear because of species and methodologic differences Somewhat overlooked, but worthy of consideration, is the notion that paracrine mechanisms modulate glucose production through nitric oxide generated in hepatic endothelial cells The Foxo pathway provides evidence that regulation of glucose and lipid production in the liver are mechanistically intertwined.
Specifically, Foxo is the key factor controlling the coordinate induction of G6pc and suppression of Gck in the postabsorptive state Fig. In normal physiology, the ratio of G6pc to Gck is key to maintaining plasma glucose levels within a narrow range. The tight linkage between the ability of insulin to suppress G6pc and activate Gck means that in insulin resistance, as insulin levels rise to maintain normal glucose production by inhibiting Foxo-dependent G6pc, they also promote Gck expression, predisposing to hepatic triglyceride accumulation and secretion.
Incidentally, this explains why G6pc levels are normal in patients with diabetes receiving treatment. Thus, an atherogenic lipoprotein profile can be viewed as the price to be paid to suppress glucose production during the euglycemic phase of insulin resistance, as well as the early stages of diabetes.
At a more fundamental level, this observation allowed us to propose that Foxo has arisen in evolution as a mechanism to control metabolic flexibility 16 , Foxo as the single checkpoint for G6pc and Gck. Insulin activates Gck and inhibits G6pc through the Akt pathways.
Surprisingly, it does so through a single mediator, Foxo. The implication of this discovery is that suppression of glucose release through inhibition of G6pc is bound to increase flux through Gck, with the potential to increase lipogenesis and glucose oxidation and storage.
TG, triglyceride. Another discovery from our studies is that insulin regulates bile acid pool composition through Cyp8b1, the 12α-hydroxylase responsible for the generation of cholic acid and deoxycholic acid. Bile acids play important roles in triglyceride and cholesterol homeostasis and affect glucose levels.
They act through different receptors, including the nuclear receptor Fxr, the cell surface receptor Tgr5, and nonreceptor-mediated mechanisms Although there has been much interest in assessing total bile acid levels, less attention has been paid to specific types of bile acids made in normal and disease conditions.
Initially synthesized as chenodeoxycholic acid and its derivative lithocholic acid, bile acids undergo conversion by gut microbes to yield cholic acid and deoxycholic acid. We discovered that insulin controls the 12α-hydroxylase enzyme necessary for the synthesis of chenodeoxycholic versus lithocholic acid, encoded by the Cyp8b1 gene, through Foxo1 The consequences of this control are important to insulin sensitivity because 12α-hydroxylated bile acids differentially influence intestinal lipid absorption and the activity of bile acid receptors.
Thus, one would predict that insulin resistance would increase 12α-hydroxylase activity and promote the formation of bile acids that increase triglyceride and cholesterol levels. This idea found support in studies of a cohort of euglycemic individuals stratified according to their insulin sensitivity, showing that levels of 12α-hydroxylated bile acids increase as a function of the quartile of insulin resistance.
In addition, principal component analyses showed a strong correlation between 12α-hydroxylated bile acids and insulin, triglyceride, and LDL cholesterol, consistent with the idea that this constellation of bile acids is proatherogenic Cyp8b1 remains an attractive target for drug development.
The ability of Foxo to coordinate the dynamic relationship of G6pc and Gck during the fasting-to-feeding transition recapitulates the challenges underlying treatments for insulin resistance. In this respect, augmentation of insulin sensitivity would inhibit glucose production lowering G6pc , potentially benefiting fasting hyperglycemia, but as hepatic glucose utilization increases, so does lipid synthesis, potentially predisposing to steatosis and release of atherogenic lipoproteins.
This is a daily conundrum in clinical practice. Our approach to this problem was to further dissect the Foxo regulation, i. We discovered the transcriptional coregulator Sin3a as the elusive Foxo-dependent Gck corepressor Fig. This discovery raised the possibility that the two functions of Foxo1—as an activator and as a repressor of genes—could be independently modulated.
Thus, we reckoned that if we selectively blocked the activating function of Foxo and left the repressor function intact, we could reduce the excessive hepatic glucose production characteristic of diabetes without increasing triglyceride synthesis.
Screening of a library of small molecules led us to identify two series of Foxo inhibitors that were pared down through a combination of biochemical, computational, and toxicology analyses to a small number that could be tested in primary hepatocytes for their ability to differentially modulate G6pc and Gck Activating and repressing function of Foxo1.
Many transcription factors have dual effects on gene expression, turning some genes on and others off. The repressor mechanism is based on the recruitment of Sin3a, a large component of the gene repressor complex, as the Foxo corepressor of Gck.
This opens up the possibility of identifying drugs that act selectively on a specific function. The expectation was that full inhibitors of Foxo1 would decrease G6pc and increase Gck , as indeed they did.
In contrast, selective Foxo1 inhibitors would lower G6pc without increasing Gck. These results were borne out by functional assays of glucose production and de novo lipogenesis in hepatocytes This work provided proof of principle that it is possible to modulate critical nodes of insulin signaling to dial up or down individual biologic responses to the hormone.
Moreover, it showed that unliganded transcription factors, long the bane of drug development, can be targeted pharmacologically, leading to selective reversal of insulin resistance. Although fraught with uncertainty, the road ahead is ripe for transformative drug discovery.
And yet, we know from clinical experience that β-cell failure can be reversed, albeit partially, for years on end, even after the onset of hyperglycemia There are at least three abnormalities of islet cell function in diabetes: impaired insulin response to stimulus, reduced number of β-cells, and inappropriate glucagon response Our interest in this area was triggered by the striking regulation of Foxo in β-cells: present but inactive in healthy β-cells, Foxo is activated in early diabetes by moving into the nucleus; however, in advanced diabetes, it disappears from β-cells even as the latter lose their insulin content 29 , 30 , Our research showed that these three features correspond to three stages in the development of β-cell failure: 1 healthy basal state, 2 impaired coupling of cellular metabolism with insulin secretion metabolic inflexibility , and 3 loss of features as a mature, hormone-laden cell dedifferentiation with conversion to other endocrine cell types Fig.
In response to the stress associated with the growing metabolic demand for insulin, β-cells respond by activating Foxo This activation boosts a network of homeostatic genes, including the maturity-onset diabetes of the young genes Hnf4α, Hnf1α, and Pdx1, to stave off failure 17 as long as the inciting causes are removed.
Otherwise, there is loss of β-cell features, appearance of progenitor-like features, and acquisition of α-cell properties. Model of the progression of β-cell failure. The graph shows a model of changes in gene expression during three phases of progression of β-cell dysfunction, indicated at the top.
Metabolic inflexibility is a state in which the pairing of ATP generation with insulin secretion is impaired. Dedifferentiation is a state in which features of mature β-cells are lost. Shown at the bottom is representative immunohistochemistry of Foxo1 translocation from the cytoplasm to the nucleus of the β-cell in response to changes in glucose, lipid, and cytokine levels.
Foxo is detected by red fluorescence, insulin by green fluorescence. Nuclear translocation is associated with the activation of a stress response that aims to prevent loss of β-cell features through the genes indicated on the right-hand column.
Nuclear Foxo1 is degraded if hyperglycemia is not reversed, paving the way for the activation of progenitor-like features and conversion into α-like—cells. This model can best be understood in the physiologic context of insulin secretion Fig. The first phase is dependent on glucose phosphorylation, closure of ATP-dependent K channels, and secretion of stored, readily releasable insulin granules, whereas the second phase requires, in addition to energy generated through mitochondrial oxidative phosphorylation, various second messengers, including glutamate, NADPH, and acyl-CoA The main source of substrate for mitochondrial oxidative phosphorylation is glucose, but amino acids and lipids also provide acetyl-CoA.
We discovered that Foxo activation in response to metabolic challenges curtails fatty acid oxidation Fig. However, activated Foxo is rapidly degraded, leading to its depletion if the metabolic challenge does not subside Rather, the constitutive rise in lipid oxidation leads to the generation of toxic intermediates, with impaired ATP production, calcium mobilization, and insulin secretion Fig.
Metabolic inflexibility and β-cell failure. A : In response to factors requiring increased β-cell insulin secretion transient hyperglycemia, increased lipids, inflammation, cytokines in the early phases of diabetes, Foxo1 translocates to the nucleus to enforce a gene expression network that includes several maturity-onset diabetes of the young genes e.
B : As Foxo1 becomes functionally exhausted, expression of maturity-onset diabetes of the young genes decreases and Pparα increases. β-Cells become transcriptionally blindsided to the effects of glucose, increasing lipid oxidation.
Interestingly, Pparγ is also activated with Pparα. We interpret this as an attempt by the β-cell to divert carbons into lipid synthesis rather than oxidation. These data provide a potential explanation for the direct beneficial effects of thiazolidinediones on β-cell function.
Cpt1, carnitine palmitoyl-transferase; GDH, glutamate dehydrogenase; Lc-FA, long chain fatty acids; PC, pyruvate carboxylase. As a long-term consequence of metabolic inflexibility, β-cells gradually lose their terminally differentiated features.
When we lineage-traced β-cells during diabetes progression, we obtained evidence that they do not die at least, not immediately but reverse their developmental pathway and come to resemble progenitor cells.
Moreover, we learned that some of these former β-cells convert to glucagon-producing, α-like—cells, providing a potential explanation for the hyperglucagonemia of diabetes We have now identified a subset of candidate genes that mediate the transition from a metabolically inflexible to a dedifferentiated β-cell The β subunits have tyrosine kinase enzyme activity which is triggered by the insulin binding.
This activity provokes the autophosphorylation of the β subunits and subsequently the phosphorylation of proteins inside the cell known as insulin receptor substrates IRS. The phosphorylation of the IRS activates a signal transduction cascade that leads to the activation of other kinases as well as transcription factors that mediate the intracellular effects of insulin.
The cascade that leads to the insertion of GLUT4 glucose transporters into the cell membranes of muscle and fat cells, and to the synthesis of glycogen in liver and muscle tissue, as well as the conversion of glucose into triglycerides in liver, adipose, and lactating mammary gland tissue, operates via the activation, by IRS-1, of phosphoinositol 3 kinase PI3K.
This enzyme converts a phospholipid in the cell membrane by the name of phosphatidylinositol 4,5-bisphosphate PIP2 , into phosphatidylinositol 3,4,5-triphosphate PIP3 , which, in turn, activates protein kinase B PKB. Activated PKB facilitates the fusion of GLUT4 containing endosomes with the cell membrane, resulting in an increase in GLUT4 transporters in the plasma membrane.
The active enzyme, glycogen synthase GS , catalyzes the rate limiting step in the synthesis of glycogen from glucose. Similar dephosphorylations affect the enzymes controlling the rate of glycolysis leading to the synthesis of fats via malonyl-CoA in the tissues that can generate triglycerides , and also the enzymes that control the rate of gluconeogenesis in the liver.
The overall effect of these final enzyme dephosphorylations is that, in the tissues that can carry out these reactions, glycogen and fat synthesis from glucose are stimulated, and glucose production by the liver through glycogenolysis and gluconeogenesis are inhibited. After the intracellular signal that resulted from the binding of insulin to its receptor has been produced, termination of signaling is then needed.
As mentioned below in the section on degradation, endocytosis and degradation of the receptor bound to insulin is a main mechanism to end signaling.
The structure of the insulin— insulin receptor complex has been determined using the techniques of X-ray crystallography. Insulin also influences other body functions, such as vascular compliance and cognition.
Once insulin enters the human brain, it enhances learning and memory and benefits verbal memory in particular. Once an insulin molecule has docked onto the receptor and effected its action, it may be released back into the extracellular environment, or it may be degraded by the cell.
The two primary sites for insulin clearance are the liver and the kidney. The liver clears most insulin during first-pass transit, whereas the kidney clears most of the insulin in systemic circulation.
Degradation normally involves endocytosis of the insulin-receptor complex, followed by the action of insulin-degrading enzyme. An insulin molecule produced endogenously by the beta cells is estimated to be degraded within about one hour after its initial release into circulation insulin half-life ~ 4—6 minutes.
Insulin is a major regulator of endocannabinoid EC metabolism and insulin treatment has been shown to reduce intracellular ECs, the 2-arachidonoylglycerol 2-AG and anandamide AEA , which correspond with insulin-sensitive expression changes in enzymes of EC metabolism. In insulin-resistant adipocytes , patterns of insulin-induced enzyme expression is disturbed in a manner consistent with elevated EC synthesis and reduced EC degradation.
Findings suggest that insulin-resistant adipocytes fail to regulate EC metabolism and decrease intracellular EC levels in response to insulin stimulation, whereby obese insulin-resistant individuals exhibit increased concentrations of ECs.
Hypoglycemia , also known as "low blood sugar", is when blood sugar decreases to below normal levels. The most common cause of hypoglycemia is medications used to treat diabetes mellitus such as insulin and sulfonylureas.
Biosynthetic human insulin insulin human rDNA, INN for clinical use is manufactured by recombinant DNA technology. Researchers have succeeded in introducing the gene for human insulin into plants as another method of producing insulin "biopharming" in safflower.
Several analogs of human insulin are available. These insulin analogs are closely related to the human insulin structure, and were developed for specific aspects of glycemic control in terms of fast action prandial insulins and long action basal insulins.
Other rapid-acting analogues are NovoRapid and Apidra , with similar profiles. Fast acting insulins do not require the injection-to-meal interval previously recommended for human insulin and animal insulins. The other type is long acting insulin; the first of these was Lantus insulin glargine.
These have a steady effect for an extended period from 18 to 24 hours. Likewise, another protracted insulin analogue Levemir is based on a fatty acid acylation approach. A myristic acid molecule is attached to this analogue, which associates the insulin molecule to the abundant serum albumin, which in turn extends the effect and reduces the risk of hypoglycemia.
Both protracted analogues need to be taken only once daily, and are used for type 1 diabetics as the basal insulin. A combination of a rapid acting and a protracted insulin is also available, making it more likely for patients to achieve an insulin profile that mimics that of the body's own insulin release.
Insulin is usually taken as subcutaneous injections by single-use syringes with needles , via an insulin pump , or by repeated-use insulin pens with disposable needles. Inhaled insulin is also available in the U. Featuring extra-thin walls and a multi-bevel tapered point, these pen needles prioritise patient comfort by minimising pain and ensuring seamless medication delivery.
The product aims to provide affordable Pen Needles to the developing part of the country through its wide distribution channel. Additionally, the universal design of these needles guarantees compatibility with all insulin pens. Unlike many medicines, insulin cannot be taken by mouth because, like nearly all other proteins introduced into the gastrointestinal tract , it is reduced to fragments, whereupon all activity is lost.
There has been some research into ways to protect insulin from the digestive tract, so that it can be administered orally or sublingually. In , the World Health Organization added insulin to its model list of essential medicines. Insulin, and all other medications, are supplied free of charge to people with diabetes by the National Health Service in the countries of the United Kingdom.
In , while studying the structure of the pancreas under a microscope , Paul Langerhans , a medical student in Berlin , identified some previously unnoticed tissue clumps scattered throughout the bulk of the pancreas. In , the physician Oskar Minkowski , in collaboration with Joseph von Mering , removed the pancreas from a healthy dog to test its assumed role in digestion.
On testing the urine, they found sugar, establishing for the first time a relationship between the pancreas and diabetes. In , another major step was taken by the American physician and scientist Eugene Lindsay Opie , when he isolated the role of the pancreas to the islets of Langerhans: "Diabetes mellitus when the result of a lesion of the pancreas is caused by destruction of the islands of Langerhans and occurs only when these bodies are in part or wholly destroyed".
Over the next two decades researchers made several attempts to isolate the islets' secretions. In George Ludwig Zuelzer achieved partial success in treating dogs with pancreatic extract, but he was unable to continue his work. Between and , E. Scott at the University of Chicago tried aqueous pancreatic extracts and noted "a slight diminution of glycosuria", but was unable to convince his director of his work's value; it was shut down.
Israel Kleiner demonstrated similar effects at Rockefeller University in , but World War I interrupted his work and he did not return to it. In , Nicolae Paulescu developed an aqueous pancreatic extract which, when injected into a diabetic dog, had a normalizing effect on blood sugar levels.
He had to interrupt his experiments because of World War I , and in he wrote four papers about his work carried out in Bucharest and his tests on a diabetic dog. Later that year, he published "Research on the Role of the Pancreas in Food Assimilation". The name "insulin" was coined by Edward Albert Sharpey-Schafer in for a hypothetical molecule produced by pancreatic islets of Langerhans Latin insula for islet or island that controls glucose metabolism.
Unbeknown to Sharpey-Schafer, Jean de Meyer had introduced the very similar word "insuline" in for the same molecule.
In October , Canadian Frederick Banting concluded that the digestive secretions that Minkowski had originally studied were breaking down the islet secretion, thereby making it impossible to extract successfully.
A surgeon by training, Banting knew that blockages of the pancreatic duct would lead most of the pancreas to atrophy, while leaving the islets of Langerhans intact. He reasoned that a relatively pure extract could be made from the islets once most of the rest of the pancreas was gone.
He jotted a note to himself: "Ligate pancreatic ducts of dog. Keep dogs alive till acini degenerate leaving Islets. In the spring of , Banting traveled to Toronto to explain his idea to John Macleod , Professor of Physiology at the University of Toronto.
Macleod was initially skeptical, since Banting had no background in research and was not familiar with the latest literature, but he agreed to provide lab space for Banting to test out his ideas. Macleod also arranged for two undergraduates to be Banting's lab assistants that summer, but Banting required only one lab assistant.
Charles Best and Clark Noble flipped a coin; Best won the coin toss and took the first shift. This proved unfortunate for Noble, as Banting kept Best for the entire summer and eventually shared half his Nobel Prize money and credit for the discovery with Best.
Banting and Best presented their results to Macleod on his return to Toronto in the fall of , but Macleod pointed out flaws with the experimental design, and suggested the experiments be repeated with more dogs and better equipment.
He moved Banting and Best into a better laboratory and began paying Banting a salary from his research grants. Several weeks later, the second round of experiments was also a success, and Macleod helped publish their results privately in Toronto that November.
Bottlenecked by the time-consuming task of duct-tying dogs and waiting several weeks to extract insulin, Banting hit upon the idea of extracting insulin from the fetal calf pancreas, which had not yet developed digestive glands.
By December, they had also succeeded in extracting insulin from the adult cow pancreas. Macleod discontinued all other research in his laboratory to concentrate on the purification of insulin. He invited biochemist James Collip to help with this task, and the team felt ready for a clinical test within a month.
On January 11, , Leonard Thompson , a year-old diabetic who lay dying at the Toronto General Hospital , was given the first injection of insulin. Over the next 12 days, Collip worked day and night to improve the ox-pancreas extract. A second dose was injected on January 23, eliminating the glycosuria that was typical of diabetes without causing any obvious side-effects.
The first American patient was Elizabeth Hughes , the daughter of U. Secretary of State Charles Evans Hughes. was future woodcut artist James D. Havens ; [] John Ralston Williams imported insulin from Toronto to Rochester, New York , to treat Havens.
Banting and Best never worked well with Collip, regarding him as something of an interloper, [ citation needed ] and Collip left the project soon after. Over the spring of , Best managed to improve his techniques to the point where large quantities of insulin could be extracted on demand, but the preparation remained impure.
The drug firm Eli Lilly and Company had offered assistance not long after the first publications in , and they took Lilly up on the offer in April. In November, Lilly's head chemist, George B. Walden discovered isoelectric precipitation and was able to produce large quantities of highly refined insulin.
Shortly thereafter, insulin was offered for sale to the general public. Toward the end of January , tensions mounted between the four "co-discoverers" of insulin and Collip briefly threatened to separately patent his purification process.
John G. FitzGerald , director of the non-commercial public health institution Connaught Laboratories , therefore stepped in as peacemaker.
The resulting agreement of 25 January established two key conditions: 1 that the collaborators would sign a contract agreeing not to take out a patent with a commercial pharmaceutical firm during an initial working period with Connaught; and 2 that no changes in research policy would be allowed unless first discussed among FitzGerald and the four collaborators.
Initially, Macleod and Banting were particularly reluctant to patent their process for insulin on grounds of medical ethics. However, concerns remained that a private third-party would hijack and monopolize the research as Eli Lilly and Company had hinted [] , and that safe distribution would be difficult to guarantee without capacity for quality control.
To this end, Edward Calvin Kendall gave valuable advice. He had isolated thyroxin at the Mayo Clinic in and patented the process through an arrangement between himself, the brothers Mayo, and the University of Minnesota , transferring the patent to the public university.
The patent would not be used for any other purpose than to prevent the taking out of a patent by other persons. When the details of the method of preparation are published anyone would be free to prepare the extract, but no one could secure a profitable monopoly.
Following further concern regarding Eli Lilly's attempts to separately patent parts of the manufacturing process, Connaught's Assistant Director and Head of the Insulin Division Robert Defries established a patent pooling policy which would require producers to freely share any improvements to the manufacturing process without compromising affordability.
Purified animal-sourced insulin was initially the only type of insulin available for experiments and diabetics. John Jacob Abel was the first to produce the crystallised form in Doisy , and Philip A.
Shaffer in Evans Jr. isolated the amino acids phenylalanine and proline in The amino acid structure of insulin was first characterized in by Frederick Sanger , [18] [] and the first synthetic insulin was produced simultaneously in the labs of Panayotis Katsoyannis at the University of Pittsburgh and Helmut Zahn at RWTH Aachen University in the mids.
Hans E. Weber discovered preproinsulin while working as a research fellow at the University of California Los Angeles in In —, Weber learned the techniques of how to isolate, purify, and translate messenger RNA. To further investigate insulin, he obtained pancreatic tissues from a slaughterhouse in Los Angeles and then later from animal stock at UCLA.
He isolated and purified total messenger RNA from pancreatic islet cells which was then translated in oocytes from Xenopus laevis and precipitated using anti-insulin antibodies. When total translated protein was run on an SDS-polyacrylamide gel electrophoresis and sucrose gradient, peaks corresponding to insulin and proinsulin were isolated.
However, to the surprise of Weber a third peak was isolated corresponding to a molecule larger than proinsulin. After reproducing the experiment several times, he consistently noted this large peak prior to proinsulin that he determined must be a larger precursor molecule upstream of proinsulin.
In May , at the American Diabetes Association meeting in New York, Weber gave an oral presentation of his work [] where he was the first to name this precursor molecule "preproinsulin". Following this oral presentation, Weber was invited to dinner to discuss his paper and findings by Donald Steiner , a researcher who contributed to the characterization of proinsulin.
A year later in April , this molecule was further characterized and sequenced by Steiner, referencing the work and discovery of Hans Weber. The first genetically engineered, synthetic "human" insulin was produced using E. coli in by Arthur Riggs and Keiichi Itakura at the Beckman Research Institute of the City of Hope in collaboration with Herbert Boyer at Genentech.
Recombinant insulin is produced either in yeast usually Saccharomyces cerevisiae or E. A chemically synthesized C-terminal tail is then grafted onto insulin by reverse proteolysis using the inexpensive protease trypsin; typically the lysine on the C-terminal tail is protected with a chemical protecting group to prevent proteolysis.
The ease of modular synthesis and the relative safety of modifications in that region accounts for common insulin analogs with C-terminal modifications e. lispro, aspart, glulisine. The Genentech synthesis and completely chemical synthesis such as that by Bruce Merrifield are not preferred because the efficiency of recombining the two insulin chains is low, primarily due to competition with the precipitation of insulin B chain.
The Nobel Prize committee in credited the practical extraction of insulin to a team at the University of Toronto and awarded the Nobel Prize to two men: Frederick Banting and John Macleod. Banting, incensed that Best was not mentioned, [] shared his prize with him, and Macleod immediately shared his with James Collip.
The patent for insulin was sold to the University of Toronto for one dollar. Two other Nobel Prizes have been awarded for work on insulin.
British molecular biologist Frederick Sanger , who determined the primary structure of insulin in , was awarded the Nobel Prize in Chemistry. Several Nobel Prizes also have an indirect connection with insulin. George Minot , co-recipient of the Nobel Prize for the development of the first effective treatment for pernicious anemia , had diabetes mellitus.
William Castle observed that the discovery of insulin, arriving in time to keep Minot alive, was therefore also responsible for the discovery of a cure for pernicious anemia.
The work published by Banting, Best, Collip and Macleod represented the preparation of purified insulin extract suitable for use on human patients.
Ian Murray was particularly active in working to correct "the historical wrong" against Nicolae Paulescu. Murray was a professor of physiology at the Anderson College of Medicine in Glasgow , Scotland , the head of the department of Metabolic Diseases at a leading Glasgow hospital, vice-president of the British Association of Diabetes, and a founding member of the International Diabetes Federation.
Murray wrote:. Insufficient recognition has been given to Paulescu, the distinguished Romanian scientist, who at the time when the Toronto team were commencing their research had already succeeded in extracting the antidiabetic hormone of the pancreas and proving its efficacy in reducing the hyperglycaemia in diabetic dogs.
In a private communication, Arne Tiselius , former head of the Nobel Institute, expressed his personal opinion that Paulescu was equally worthy of the award in Contents move to sidebar hide. Article Talk. Read Edit View history. Tools Tools. What links here Related changes Upload file Special pages Permanent link Page information Cite this page Get shortened URL Download QR code Wikidata item.
Download as PDF Printable version. In other projects. Wikimedia Commons. Peptide hormone. This article is about the naturally occurring protein.
For uses of insulin in treating diabetes, see Insulin medication. Not to be confused with Inulin. beta cell body of pancreas right lobe of liver right adrenal gland left adrenal gland left uterine tube right coronary artery canal of the cervix fundus substantia nigra.
islet of Langerhans pyloric antrum yolk sac retinal pigment epithelium secondary oocyte quadriceps femoris muscle ankle sexually immature organism neuron spermatid. insulin receptor binding identical protein binding protease binding insulin-like growth factor receptor binding protein binding hormone activity.
endoplasmic reticulum lumen transport vesicle Golgi membrane secretory granule lumen Golgi lumen endoplasmic reticulum-Golgi intermediate compartment membrane endosome lumen extracellular region extracellular space. See also: Blood glucose regulation.
Main article: Insulin oscillations. Further information: Insulin index. Main article: Hypoglycemia. Main article: Insulin medication. Richardson diagram of a porcine insulin monomer, showing its characteristic secondary structure.
This is the biologically active form of insulin. Richardson diagram of a porcine insulin hexamer. The sphere at the center is a stabilizing zinc atom, surrounded by coordinating histidine residues. This is the form in which insulin is stored in beta cells. National Center for Biotechnology Information, U.
National Library of Medicine. Lexico Dictionaries English. Archived from the original on August 1, com Dictionary of English". Biochemistry 4th ed. New York: Wiley. Biochemistry Fourth ed. New York: W. Freeman and Company. As a general rule, assume that these insulins have duration of action of 4 hours.
The larger the dose of regular the faster the onset of action, but the longer the time to peak effect and the longer the duration of the effect.
NPH Human Insulin which has an onset of insulin effect of 1 to 2 hours, a peak effect of 4 to 6 hours, and duration of action of more than 12 hours. Very small doses will have an earlier peak effect and shorter duration of action, while higher doses will have a longer time to peak effect and prolonged duration.
Pre-Mixed Insulin which is NPH pre-mixed with either regular human insulin or a rapid- acting insulin analog. The insulin action profile is a combination of the short and intermediate acting insulins. The insulin effect plateaus over the next few hours and is followed by a relatively flat duration of action that lasts hours for insulin detemir and 24 hours for insulin glargine.
Self assessment quizzes are available for topics covered in this website. To find out how much you have learned about Insulin Therapy , take our self assessment quiz when you have completed this section.
The quiz is multiple choice. Please choose the single best answer to each question. At the end of the quiz, your score will display. All rights reserved.
Human Insulin and Insulin Analogs Balancing restrictions and goals available for insulin replacement therapy. Insulins mevhanism are classified by the timing of Insu,in action Insulin mechanism of action your body — acction, how quickly they start to fo, when they have a maximal effect and how long they act. Insulin analogs have been developed because human insulins have limitations when injected under the skin. In high concentrations, such as in a vial or cartridge, human and also animal insulin clumps together. This clumping causes slow and unpredictable absorption from the subcutaneous tissue and a dose-dependent duration of action i.Insulin mechanism of action -
Contrary to conventional linear RNA, circRNAs are noncoding RNAs that generated from precursor mRNAs by back-splicing circularization, which is derived from exonic circRNAs, intronic circRNAs, exonic-intronic circRNAs and ntergenic circRNAs.
Recent studies have suggested that newly identified circRNAs are novel factors in the initiation and development of IR. CircHIPK3 is one of the most abundant circRNAs in β-cells and regulates hyperglycemia and IR by sequestering miRp and miRp, thereby increasing mRNA expression of key β-cell genes e.
Similar to the miRNAs and lncRNAs, several circRNAs also contribute to the the regulation of glucose and lipid homeostasis. Deep sequencing analysis of adipose circRNA revealed that circArhgap is highly upregulated during differentiation of human white adipocytes.
Thus, circRNAs likely serve as important regulators of adipocyte differentiation and lipid metabolism. Another circRNA deep sequencing analysis of sera from patients with metabolic syndrome MetS identified the presence of a novel circRNA, circRNF, involved in MetS progression.
AMPK is a critical factor in energy homeostasis including glycolysis, lipolysis, and fatty acid oxidation FAO. CircACC1 is a circRNA derived from the human acetyl-CoA carboxylase 1 ACC1 gene and directly binds to the β and γ subunits of AMPK, facilitating its activity, and promoting glycolysis and fatty acid β-oxidation during metabolic stress.
circMAP3K4 is another potentially important circRNA involved in glucose metabolism that is highly expressed in the placentas of patients with gestational diabetes mellitus GDM and the IR model.
Nevertheless, the exact roles and regulatory mechanisms of circRNAs in IR require additional clarity. The microbes living in the human gut are key contributors to host metabolism and immune function through mediating the interaction between the host and environment, or releasing metabolites and cytokines.
Different factors influencing these alterations of gut microbiome composition have been explored including diet, exercise, circadian disruption, antibiotics treatments, and genetics.
The gut microbial communities of the groups significantly diverged over time, with participants on animal diets experiencing proliferation of bile-tolerant microorganisms e. For example, microbiome genome-wide association studies mGWAS have identified that variants of different genes for example, VDR , LCT , NOD2 , FUT2 , and APOA5 that are associated with distinct gut microbiome compositions.
Growing evidence in the last two decades has suggested that gut microbial dysbiosis contributes to increased risks of metabolic defects like obesity, IR, and diabetes. LPS circulation then contributes to the chronic inflammation of liver and adipose tissue that is associated with the development of IR, in addition to other conditions associated with metabolic syndromes.
As we all know, IR is a state in which higher than normal concentrations of insulin are needed for a normal response, leading directly to hyperinsulinaemia and impaired glucose tolerance. Non-alcoholic fatty liver disease NAFLD is one of the most common liver diseases worldwide.
Adipose tissue is a physiologic reservoir of fatty acids, when the storage capacity is exceeded, the accumulation of heterotopic lipids leads to lipotoxicity, thereby promoting low-grade inflammation and IR in the liver.
Lipotoxic injury appears to occur in response to excessive levels of serum free fatty acids FFAs in hepatocytes. At present, the molecular mechanism of insulin in PCOS has been well described.
Such modifications then activate NF-κB that is involved in the expression of proinflammatory mediators such as TNF and IL-6, , and that induces key steroidogenic molecules, like CYP11A1, CYP17A1 and StAR, leading to further aggravation of hyperandrogenemia.
Cardiovascular diseases CVDs are the leading causes of death globally. The World Health Organization estimates that Moreover, over 23 million people are estimated to die from CVDs each year by However, the most common types of CVDs include high blood pressure, coronary artery disease CAD , stroke, cerebrovascular disease and rheumatic heart disease RHD.
Identifying new therapies to reduce IR may contribute to the reduced prevalence of CVDs. Insulin primarily enters the brain via selective, saturable transport across the blood-brain barrier BBB , , Peripherally produced insulin can also be actively transported into the brain via an endocytic-exocytic mechanism.
Current researches have demonstrated that the mechanisms of systemic IR and brain-specific IR have close links with AD pathogenesis. Pioglitazone acts similarly as Rosiglitazone by reducing tau and Aβ deposits in the hippocampus, and improving neuronal plasticity and learning in AD.
Moreover, overlapping pathological features exist for diabetes, IR, and AD. Chronic kidney disease CKD involves a gradual loss of kidney function and inability to filter blood , and is a major risk factor for end-stage kidney failure ESKF and CVDs.
Numerous recent epidemiological studies have suggested that IR increases the risks for different cancers including colon, liver, pancreas, breast, endometrium, thyroid and gastric cancer. Further, a growing body of evidence suggests that increased insulin, in addition to IGF1 and IGF2 levels critically influence tumor initiation and progression in IR patients.
As we all know, IR is related to several metabolic abnormalities including obesity, glucose tolerance, dyslipidemia, type 2 diabetes and other metabolic syndrome.
Actually, IR precedes the occurrence of T2DM, so how to increase the accurate assessment of insulin sensitivity is very important to predict the risk and evaluate the management of impaired insulin sensitivity and metabolic syndrome in research and clinical practice.
HOMA2 updated HOMA model which took account of variations in hepatic and peripheral glucose resistance , homeostatic Model Assessment for IR HOMA-IR , the oral glucose insulin sensitivity index OGSI , fasting Insulin FINS , and fasting plasma glucose FPG based on fasting glucose and insulin levels , , , , are widely utilized IR measurements in clinical research.
Other indices based on fasting insulin include the glucose to insulin ratio GIR , the quantitative insulin sensitivity check index QUICKI , , , triglycerides McAuley Index alone or in accordance with HDL cholesterol HDL-C , whole-body insulin sensitivity index WBISI , Matsuda Index to evaluate whole body physiological insulin sensitivity by the above methods.
Indeed, the early symptoms of IR in different individuals are not obvious, and the related symptoms are very complex, combining with screening indicators may provide more precise diagnosis for IR in the general population.
No medications exist currently that are specifically approved to treat IR, but IR management 91 , , is possible through lifestyle changes like dietary, increased exercise, and disease prevention in addition to alternative medications Fig. Among these treatments, lifestyle changes should be the main focus for IR treatment, with nutritional intervention to decrease calories, avoidance of carbohydrates, and focusing on aliments with low glycemic index including vegetables, fruits, whole-grain products, nuts, lean meats or beans to provide higher fiber, vitamins, healthy fats and protein are particularly helpful for people trying to improve insulin sensitivity.
Table 1. Metformin is a first-line medication and the most widely-prescribed insulin-sensitizing agent in T2DM and PCOS patients.
For example, 1 Glucagon-like peptide 1 GLP1 is an intestinal hormone that can enhance insulin secretion in a glucose-dependent manner by activating the GLP-1 receptor GLP-1R that is highly expressed on islet β cells.
are now world-wide therapy of T2DM since and could improve insulin sensitivity. In clinical research, scientists and physicians have explored different strategies to prevent and treat diabetes mellitus and IR. gov to reduce IR and summarized them mainly include: 1 Diet intervention, such as Low-fat vegetarian Food, high-protein food, calorie restriction, vitamin D supplementation to reduce the IR in human obesity.
We present some clinical trials of IR intervention in Table 2. Over the past years, our knowledge of the pathogenesis of IR and T2DM has improved, the development of new treatments of IR and metabolic syndrome have gained certain success, while the complexity of IR and the presence of multiple feedback loops make a challenge to the specific intervention.
In recent years, accumulating preclinical studies on the intervention of IR have been reported, which have important reference significance for the development of new drugs. We present the related studies on IR reported in recent years in Table 3 , including animal models, treatment methods and results.
Pre-clinical IR intervention mainly includes drug intervention, probiotic therapy and exercise supplement. Drug therapy to improve IR is the main research direction at present.
Researchers found that Valdecoxib VAL can inhibit inflammation and endoplasmic reticulum ER stress through AMPK-regulated HSPB1 pathway, thus improving skeletal muscle IR under hyperlipidemia. The researchers found that the mixed nasal administration of GLP-1 receptor agonist and L-form of peneracin can effectively alleviate the cognitive dysfunction of SAMP8 mice.
Natividad et al. Regular exercise is an alternative intervention measure to maintain the blood sugar level in the normal range and reduce the risk factors.
Hsu and colleagues found that exercise combined with probiotics intervention can have a positive effect on blood sugar and increase insulin sensitivity in mice. The above results show that drug intervention, probiotic supplementation and intensive exercise can improve IR but more clinical data are still needed.
Overall, the increased incidence of IR and the key roles of IR plays in many diseases, urgently require a better understanding of IR pathogenesis in addition to how IR interacts with genetics and different environments.
A deeper understanding of IR can be achieved with a more systematic approach involving large-scale omics to study the molecular landscape is of major importance in addition to exploring new intervention strategies to prevent abnormal IR syndrome.
Banting, F. The internal secretion of the pancreas. Indian J. CAS PubMed Google Scholar. SANGER, F. The amino-acid sequence in the glycyl chain of insulin. The identification of lower peptides from partial hydrolysates.
Biochem J. Article CAS PubMed PubMed Central Google Scholar. The investigation of peptides from enzymic hydrolysates. Kung, Y. Total synthesis of crystalline bovine insulin.
Goeddel, D. et al. Expression in Escherichia coli of chemically synthesized genes for human insulin. USA 76 , — Vecchio, I. The discovery of insulin: an important milestone in the history of medicine. Front Endocrinol. Article Google Scholar. Cheatham, B. Insulin action and the insulin signaling network.
Root, H. Insulin resistance and bronze diabetes. Laakso, M. Insulin resistance and hyperglycaemia in cardiovascular disease development.
Article CAS PubMed Google Scholar. Bugianesi, E. Insulin resistance in nonalcoholic fatty liver disease. Saklayen, M. The global epidemic of the metabolic syndrome.
Diamanti-Kandarakis, E. Insulin resistance and the polycystic ovary syndrome revisited: an update on mechanisms and implications. Stenvers, D. Circadian clocks and insulin resistance.
Article PubMed CAS Google Scholar. Freeman AM, Pennings N. Insulin Resistance. In: StatPearls Internet. Treasure Island FL : StatPearls Publishing. PMID: American Diabetes Association. Prevention or delay of type 2 diabetes: standards of medical care in diabetes Diabetes Care 44 , S34—S39 Pharmacologic approaches to glycemic treatment: Standards of Medical Care in Diabetes Diabetes Care 44 , S—S Weiss, M.
Insulin biosynthesis, secretion, structure, and structure-activity relationships. In: Feingold KR, Anawalt B, Boyce A, et al. South Dartmouth MA : MDText. com, Inc.
Sanger, F. Chemistry of insulin. Science , — Katsoyannis, P. Synthesis of insulin. Lee, J. The insulin receptor: structure, function, and signaling. Pessin, J.
Signaling pathways in insulin action: molecular targets of insulin resistance. Invest , — Haeusler, R. Biochemical and cellular properties of insulin receptor signalling. Cell Biol. White, M. Mechanisms of insulin action. In Atlas of diabetes pp. Springer, Boston, MA Newgard, C.
Organizing glucose disposal: emerging roles of the glycogen targeting subunits of protein phosphatase Diabetes 49 , — Beurel, E. Glycogen synthase kinase-3 GSK3 : regulation, actions, and diseases.
Article CAS Google Scholar. Dong, X. Inactivation of hepatic Foxo1 by insulin signaling is required for adaptive nutrient homeostasis and endocrine growth regulation.
Cell Metab. Puigserver, P. Insulin-regulated hepatic gluconeogenesis through FOXO1—PGC-1α interaction. Nature , — Vander Haar, E. Garami, A. Cell 11 , — Laplante, M. mTORC1 activates SREBP-1c and uncouples lipogenesis from gluconeogenesis. USA , — Han, Y. Post-translational regulation of lipogenesis via AMPK-dependent phosphorylation of insulin-induced gene.
Calejman, C. mTORC2-AKT signaling to ATP-citrate lyase drives brown adipogenesis and de novo lipogenesis. Xia, W. Loss of ABHD15 impairs the anti-lipolytic action of insulin by altering PDE3B stability and contributes to insulin resistance.
Cell Rep. James, D. The aetiology and molecular landscape of insulin resistance. Tam, C. Defining insulin resistance from hyperinsulinemic-euglycemic clamps. Diabetes care 35 , — Samuel, V. Mechanisms for insulin resistance: common threads and missing links.
Cell , — Ye, J. Mechanisms of insulin resistance in obesity. Front Med. Article PubMed PubMed Central Google Scholar. Yaribeygi, H. Insulin resistance: Review of the underlying molecular mechanisms. Cell Physiol.
De Meyts, P. The insulin receptor: a prototype for dimeric, allosteric membrane receptors? Trends Biochem Sci.
Caro, J. Insulin receptor kinase in human skeletal muscle from obese subjects with and without noninsulin dependent diabetes. Invest 79 , — Fröjdö, S. Alterations of insulin signaling in type 2 diabetes: a review of the current evidence from humans.
Biochim Biophys. Acta , 83—92 Fisher, S. Michael, M. Loss of insulin signaling in hepatocytes leads to severe insulin resistance and progressive hepatic dysfunction.
Cell 6 , 87—97 Davis, R. The c-Jun NH2-terminal kinase promotes insulin resistance during association with insulin receptor substrate-1 and phosphorylation of Ser Article PubMed Google Scholar. Carvalho-Filho, M. Diabetes 54 , — Taniguchi, C.
Critical nodes in signalling pathways: insights into insulin action. Brachmann, S. Phosphoinositide 3-kinase catalytic subunit deletion and regulatory subunit deletion have opposite effects on insulin sensitivity in mice.
Phosphatidylinositol 3-kinase activation is required for insulin stimulation of pp70 S6 kinase, DNA synthesis, and glucose transporter translocation. CAS PubMed PubMed Central Google Scholar.
Czech, M. Signaling mechanisms that regulate glucose transport. Luo, J. Loss of class IA PI3K signaling in muscle leads to impaired muscle growth, insulin response, and hyperlipidemia. Cong, L. Physiological role of Akt in insulin-stimulated translocation of GLUT4 in transfected rat adipose cells.
Xia, J. Targeted induction of ceramide degradation leads to improved systemic metabolism and reduced hepatic steatosis. Le Marchand-Brustel, Y.
Insulin receptor tyrosine kinase is defective in skeletal muscle of insulin-resistant obese mice. Brozinick, J. Defective signaling through Akt-2 and-3 but not Akt-1 in insulin-resistant human skeletal muscle: potential role in insulin resistance.
Diabetes 52 , — Kruszynska, Y. Fatty acid-induced insulin resistance: decreased muscle PI3K activation but unchanged Akt phosphorylation. Choi, K. Molecular mechanism of insulin resistance in obesity and type 2 diabetes.
Korean J. Intern Med. Kahn, B. Glucose transport: pivotal step in insulin action. Diabetes 45 , — Dimitriadis, G. Insulin effects in muscle and adipose tissue. Diabetes Res Clin. Shepherd, P. Glucose transporters and insulin action-implications for insulin resistance and diabetes mellitus.
Li, J. Reduced glucose uptake precedes insulin signaling defects in adipocytes from heterozygous GLUT4 knockout mice. FASEB J. Klip, A. Recruitment of GLUT-4 glucose transporters by insulin in diabetic rat skeletal muscle.
Biochem Biophys. Res Commun. Etgen, G. Jr et al. Exercise training reverses insulin resistance in muscle by enhanced recruitment of GLUT-4 to the cell surface. Ryder, J. Use of a novel impermeable biotinylated photolabeling reagent to assess insulin-and hypoxia-stimulated cell surface GLUT4 content in skeletal muscle from type 2 diabetic patients.
Garvey, W. Multiple defects in the adipocyte glucose transport system cause cellular insulin resistance in gestational diabetes: heterogeneity in the number and a novel abnormality in subcellular localization of GLUT4 glucose transporters. Diabetes 42 , — Chadt, A.
Deletion of both Rab-GTPase—activating proteins TBC14KO and TBC1D4 in mice eliminates insulin-and AICAR-stimulated glucose transport. Diabetes 64 , — Chen, S. Tramunt, B. Sex differences in metabolic regulation and diabetes susceptibility.
Diabetologia 63 , — Greenhill, C. Sex differences in insulin resistance. Qiu, J. Estradiol protects proopiomelanocortin neurons against insulin resistance. Endocrinology , — Zidon, T. Effects of ERβ and ERα on OVX-induced changes in adiposity and insulin resistance.
Ikeda, K. Functions of estrogen and estrogen receptor signaling on skeletal muscle. Steroid Biochem Mol. Gerdts, E. Sex differences in cardiometabolic disorders. Chia, C. Age-related changes in glucose metabolism, hyperglycemia, and cardiovascular risk. Shi, H. Sex differences in obesity-related glucose intolerance and insulin resistance.
Glucose Tolerance 4 , 37—66 Google Scholar. Geer, E. Gender differences in insulin resistance, body composition, and energy balance. Christen, T. Sex differences in body fat distribution are related to sex differences in serum leptin and adiponectin.
Peptides , 25—31 Palmisano, B. Sex differences in lipid and lipoprotein metabolism. Kodama, K. Ethnic differences in the relationship between insulin sensitivity and insulin response: a systematic review and meta-analysis.
Diabetes Care 36 , — Raygor, V. Diab Vasc. Sumner, A. Ethnic differences in the ability of triglyceride levels to identify insulin resistance.
Atherosclerosis , — Tan, V. Ethnic differences in insulin sensitivity and beta-cell function among Asian men. Diabetes 5 , e—e Ministry of Health Singapore MOHS. Potts, J. Sex and ethnic group differences in fat distribution in young United Kingdom South Asians and Europids.
Ehtisham, S. Ethnic differences in insulin resistance and body composition in United Kingdom adolescents. Lear, S. Ethnic variation in fat and lean body mass and the association with insulin resistance. Mason, C. Dietary weight loss and exercise effects on insulin resistance in postmenopausal women.
Med 41 , — Mikusova, V. Insulin resistance and need for a lifestyle change to eliminate it. Listy , — orpeleijn, E. Metabolic flexibility in the development of insulin resistance and type 2 diabetes: effects of lifestyle. Shigeta, H. Lifestyle, obesity, and insulin resistance.
Diabetes Care 24 , Oosterman, J. The circadian clock, shift work, and tissue-specific insulin resistance. Endocrinology , bqaa McAuley, K.
Intensive lifestyle changes are necessary to improve insulin sensitivity: a randomized controlled trial. Diabetes care 25 , — Bergman, B. Novel and reversible mechanisms of smoking-induced insulin resistance in humans. Diabetes 61 , — Kan, C. A systematic review and meta-analysis of the association between depression and insulin resistance.
Diabetes care 36 , — Sung, C. Role of vitamin D in insulin resistance. Ardabili, H. Vitamin D supplementation has no effect on insulin resistance assessment in women with polycystic ovary syndrome and vitamin D deficiency. Pasieka, A. Impact of glucocorticoid excess on glucose tolerance: clinical and preclinical evidence.
Metabolites 6 , 24 Article PubMed Central CAS Google Scholar. Rizza, R. Cortisol-induced insulin resistance in man: impaired suppression of glucose production and stimulation of glucose utilization due to a postreceptor defect of insulin action.
Effects of growth hormone on insulin action in man: mechanisms of insulin resistance, impaired suppression of glucose production, and impaired stimulation of glucose utilization.
Diabetes 31 , — Barbour, L. A Cellular mechanisms for insulin resistance in normal pregnancy and gestational diabetes. Diabetes Care 30 , S—S Parichatikanond, W. Prolonged stimulation of β2-adrenergic receptor with β2-agonists impairs insulin actions in H9c2 cells.
Walli, R. Treatment with protease inhibitors associated with peripheral insulin resistance and impaired oral glucose tolerance in HIVinfected patients.
AIDS 12 , F—F Murata, H. The mechanism of insulin resistance caused by HIV protease inhibitor therapy. Teff, K. Antipsychotic-induced insulin resistance and postprandial hormonal dysregulation independent of weight gain or psychiatric disease. Diabetes 62 , — Bittencourt, M.
Insulin therapy in insulin resistance: could it be part of a lethal pathway? Elbein, S. Heritability of pancreatic beta-cell function among nondiabetic members of Caucasian familial type 2 diabetic kindreds.
Shulman, G. Cellular mechanisms of insulin resistance. Knauf, C. Brain glucagon-like peptide-1 increases insulin secretion and muscle insulin resistance to favor hepatic glycogen storage.
Petersen, M. Regulation of hepatic glucose metabolism in health and disease. Matsumoto, M. Dual role of transcription factor FoxO1 in controlling hepatic insulin sensitivity and lipid metabolism. Shimomura, I. Cell 6 , 77—86 Petersen, K.
Mechanism by which glucose and insulin inhibit net hepatic glycogenolysis in humans. Henriksen, E. Role of glycogen synthase kinase-3 in insulin resistance and type 2 diabetes. Drug Targets 7 , — Karim, S. Hepatic expression and cellular distribution of the glucose transporter family.
World J. Rencurel, F. Requirement of glucose metabolism for regulation of glucose transporter type 2 GLUT2 gene expression in liver. Thorens, B. Diabetologia 58 , — Eberlé, D. SREBP transcription factors: master regulators of lipid homeostasis.
Biochimie 86 , — Horton, J. SREBPs: activators of the complete program of cholesterol and fatty acid synthesis in the liver. Ferré, P. Hepatic steatosis: a role for de novo lipogenesis and the transcription factor SREBP-1c.
Diabetes Obes. Tobe, K. Dentin, R. Carbohydrate responsive element binding protein ChREBP and sterol regulatory element binding protein-1c SREBP-1c : two key regulators of glucose metabolism and lipid synthesis in liver. Biochimie 87 , 81—86 Hepatic glucokinase is required for the synergistic action of ChREBP and SREBP-1c on glycolytic and lipogenic gene expression.
Herman, M. A novel ChREBP isoform in adipose tissue regulates systemic glucose metabolism. Iizuka, K. Deficiency of carbohydrate response element-binding protein ChREBP reduces lipogenesis as well as glycolysis.
Natl Acad. Jaworski, K. Regulation of triglyceride metabolism. Hormonal regulation of lipolysis in adipose tissue. Liver Physiol. Vaughan, M. Hormone-sensitive lipase and monoglyceride lipase activities in adipose tissue. Zmuda-Trzebiatowska, E. Role of PDE3B in insulin-induced glucose uptake, GLUT-4 translocation and lipogenesis in primary rat adipocytes.
Cell Signal 18 , — Choi, Y. Alterations in regulation of energy homeostasis in cyclic nucleotide phosphodiesterase 3B—null mice. Martinez-Botas, J. Genet 26 , — Tansey, J. Perilipin ablation results in a lean mouse with aberrant adipocyte lipolysis, enhanced leptin production, and resistance to diet-induced obesity.
USA 98 , — Mechanisms of Insulin Action and Insulin Resistance. Kimball, S. Regulation of protein synthesis by insulin. Pösö, A. Multifunctional control of amino acids of deprivation-induced proteolysis in liver. Role of leucine.
Marshall, S. New insights into the metabolic regulation of insulin action and insulin resistance: role of glucose and amino acids. Rudrappa, S. Human skeletal muscle disuse atrophy: effects on muscle protein synthesis, breakdown, and insulin resistance-a qualitative review.
Front Physiol. Medeiros, C. Antuna-Puente, B. Adipokines: the missing link between insulin resistance and obesity. Diabetes Metab. Rabe, K. Adipokines and insulin resistance. Adipokines mediate inflammation and insulin resistance. Lausanne 4 , 71 Li, S. Adiponectin levels and risk of type 2 diabetes: a systematic review and meta-analysis.
JAMA , — Hotta, K. Circulating concentrations of the adipocyte protein adiponectin are decreased in parallel with reduced insulin sensitivity during the progression to type 2 diabetes in rhesus monkeys. Diabetes 50 , — Takahashi, M. Chemerin enhances insulin signaling and potentiates insulin-stimulated glucose uptake in 3T3-L1 adipocytes.
FEBS Lett. Yamauchi, T. Targeted disruption of AdipoR1 and AdipoR2 causes abrogation of adiponectin binding and metabolic actions. Li, L. Changes and relations of circulating visfatin, apelin, and resistin levels in normal, impaired glucose tolerance, and type 2 diabetic subjects.
Diabetes , — Soriguer, F. Apelin levels are increased in morbidly obese subjects with type 2 diabetes mellitus. Yue, P. Apelin is necessary for the maintenance of insulin sensitivity. American journal of physiology. Apelin decreases lipolysis via G q , G i , and AMPK-dependent mechanisms.
Endocrinology , 59—68 Segal, K. Relationship between insulin sensitivity and plasma leptin concentration in lean and obese men. Amitani, M. The role of leptin in the control of insulin-glucose axis.
Front Neurosci. Article PubMed PubMed Central CAS Google Scholar. Ceddia, R. Analysis of paradoxical observations on the association between leptin and insulin resistance. Covey, S. The pancreatic beta cell is a key site for mediating the effects of leptin on glucose homeostasis.
Seufert, J. Leptin effects on pancreatic beta-cell gene expression and function. Diabetes 53 , S—S Myers, M. Mechanisms of leptin action and leptin resistance. Burguera, B. Obesity is associated with a decreased leptin transport across the blood-brain barrier in rats. Gainsford, T. Leptin can induce proliferation, differentiation, and functional activation of hemopoietic cells.
USA 93 , — Scherer, P. Adipose tissue: from lipid storage compartment to endocrine organ. Diabetes 55 , — Saponaro, C. The subtle balance between lipolysis and lipogenesis: a critical point in metabolic homeostasis.
Nutrients 7 , — Frayn, K. Adipose tissue and the insulin resistance syndrome. Roden, M. Mechanism of free fatty acid-induced insulin resistance in humans. Invest 97 , — Goossens, G.
The role of adipose tissue dysfunction in the pathogenesis of obesity-related insulin resistance. RANDLE, P. The glucose fatty-acid cycle. Its role in insulin sensitivity and the metabolic disturbances of diabetes mellitus.
Lancet 1 , — Randle, P. The glucose fatty acid cycle in obesity and maturity onset diabetes mellitus. Y Acad. Brechtel, K. Fast elevation of the intramyocellular lipid content in the presence of circulating free fatty acids and hyperinsulinemia: a dynamic 1H-MRS study.
Reson Med 45 , — Boden, G. Role of fatty acids in the pathogenesis of insulin resistance and NIDDM. Diabetes 46 , 3—10 Rothman, D. USA 92 , — Cline, G. Impaired glucose transport as a cause of decreased insulin-stimulated muscle glycogen synthesis in type 2 diabetes. Dresner, A. Effects of free fatty acids on glucose transport and IRSassociated phosphatidylinositol 3-kinase activity.
Yu, C. Mechanism by which fatty acids inhibit insulin activation of insulin receptor substrate-1 IRS-1 -associated phosphatidylinositol 3-kinase activity in muscle.
Erion, D. Diacylglycerol-mediated insulin resistance. Kim, J. PKC-theta knockout mice are protected from fat-induced insulin resistance. Nagai, Y. The role of peroxisome proliferator-activated receptor gamma coactivator-1 beta in the pathogenesis of fructose-induced insulin resistance.
Yen, C. The triacylglycerol synthesis enzyme DGAT1 also catalyzes the synthesis of diacylglycerols, waxes, and retinyl esters.
Lipid Res. Timmers, S. Muscular diacylglycerol metabolism and insulin resistance. I will not attempt to summarize, let alone reconcile, the many different opinions on these phenomena. No animal model can be said to fully recapitulate the human pathophysiology, in part because the latter is likely comprised of several distinct subphenotypes whose relative pathophysiologic contribution changes over the course of the disease.
Which experimental lessons can be generalized and contribute to new approaches to treatment? First, suppression of adipocyte lipolysis by insulin is required to suppress hepatic glucose production in humans as well as rodents.
Whether central nervous system—derived signals are also important in this process remains unclear because of species and methodologic differences Somewhat overlooked, but worthy of consideration, is the notion that paracrine mechanisms modulate glucose production through nitric oxide generated in hepatic endothelial cells The Foxo pathway provides evidence that regulation of glucose and lipid production in the liver are mechanistically intertwined.
Specifically, Foxo is the key factor controlling the coordinate induction of G6pc and suppression of Gck in the postabsorptive state Fig. In normal physiology, the ratio of G6pc to Gck is key to maintaining plasma glucose levels within a narrow range.
The tight linkage between the ability of insulin to suppress G6pc and activate Gck means that in insulin resistance, as insulin levels rise to maintain normal glucose production by inhibiting Foxo-dependent G6pc, they also promote Gck expression, predisposing to hepatic triglyceride accumulation and secretion.
Incidentally, this explains why G6pc levels are normal in patients with diabetes receiving treatment. Thus, an atherogenic lipoprotein profile can be viewed as the price to be paid to suppress glucose production during the euglycemic phase of insulin resistance, as well as the early stages of diabetes.
At a more fundamental level, this observation allowed us to propose that Foxo has arisen in evolution as a mechanism to control metabolic flexibility 16 , Foxo as the single checkpoint for G6pc and Gck. Insulin activates Gck and inhibits G6pc through the Akt pathways.
Surprisingly, it does so through a single mediator, Foxo. The implication of this discovery is that suppression of glucose release through inhibition of G6pc is bound to increase flux through Gck, with the potential to increase lipogenesis and glucose oxidation and storage.
TG, triglyceride. Another discovery from our studies is that insulin regulates bile acid pool composition through Cyp8b1, the 12α-hydroxylase responsible for the generation of cholic acid and deoxycholic acid.
Bile acids play important roles in triglyceride and cholesterol homeostasis and affect glucose levels. They act through different receptors, including the nuclear receptor Fxr, the cell surface receptor Tgr5, and nonreceptor-mediated mechanisms Although there has been much interest in assessing total bile acid levels, less attention has been paid to specific types of bile acids made in normal and disease conditions.
Initially synthesized as chenodeoxycholic acid and its derivative lithocholic acid, bile acids undergo conversion by gut microbes to yield cholic acid and deoxycholic acid.
We discovered that insulin controls the 12α-hydroxylase enzyme necessary for the synthesis of chenodeoxycholic versus lithocholic acid, encoded by the Cyp8b1 gene, through Foxo1 The consequences of this control are important to insulin sensitivity because 12α-hydroxylated bile acids differentially influence intestinal lipid absorption and the activity of bile acid receptors.
Thus, one would predict that insulin resistance would increase 12α-hydroxylase activity and promote the formation of bile acids that increase triglyceride and cholesterol levels.
This idea found support in studies of a cohort of euglycemic individuals stratified according to their insulin sensitivity, showing that levels of 12α-hydroxylated bile acids increase as a function of the quartile of insulin resistance.
In addition, principal component analyses showed a strong correlation between 12α-hydroxylated bile acids and insulin, triglyceride, and LDL cholesterol, consistent with the idea that this constellation of bile acids is proatherogenic Cyp8b1 remains an attractive target for drug development.
The ability of Foxo to coordinate the dynamic relationship of G6pc and Gck during the fasting-to-feeding transition recapitulates the challenges underlying treatments for insulin resistance.
In this respect, augmentation of insulin sensitivity would inhibit glucose production lowering G6pc , potentially benefiting fasting hyperglycemia, but as hepatic glucose utilization increases, so does lipid synthesis, potentially predisposing to steatosis and release of atherogenic lipoproteins.
This is a daily conundrum in clinical practice. Our approach to this problem was to further dissect the Foxo regulation, i. We discovered the transcriptional coregulator Sin3a as the elusive Foxo-dependent Gck corepressor Fig. This discovery raised the possibility that the two functions of Foxo1—as an activator and as a repressor of genes—could be independently modulated.
Thus, we reckoned that if we selectively blocked the activating function of Foxo and left the repressor function intact, we could reduce the excessive hepatic glucose production characteristic of diabetes without increasing triglyceride synthesis.
Screening of a library of small molecules led us to identify two series of Foxo inhibitors that were pared down through a combination of biochemical, computational, and toxicology analyses to a small number that could be tested in primary hepatocytes for their ability to differentially modulate G6pc and Gck Activating and repressing function of Foxo1.
Many transcription factors have dual effects on gene expression, turning some genes on and others off. The repressor mechanism is based on the recruitment of Sin3a, a large component of the gene repressor complex, as the Foxo corepressor of Gck.
This opens up the possibility of identifying drugs that act selectively on a specific function. The expectation was that full inhibitors of Foxo1 would decrease G6pc and increase Gck , as indeed they did.
In contrast, selective Foxo1 inhibitors would lower G6pc without increasing Gck. These results were borne out by functional assays of glucose production and de novo lipogenesis in hepatocytes This work provided proof of principle that it is possible to modulate critical nodes of insulin signaling to dial up or down individual biologic responses to the hormone.
Moreover, it showed that unliganded transcription factors, long the bane of drug development, can be targeted pharmacologically, leading to selective reversal of insulin resistance. Although fraught with uncertainty, the road ahead is ripe for transformative drug discovery.
And yet, we know from clinical experience that β-cell failure can be reversed, albeit partially, for years on end, even after the onset of hyperglycemia There are at least three abnormalities of islet cell function in diabetes: impaired insulin response to stimulus, reduced number of β-cells, and inappropriate glucagon response Our interest in this area was triggered by the striking regulation of Foxo in β-cells: present but inactive in healthy β-cells, Foxo is activated in early diabetes by moving into the nucleus; however, in advanced diabetes, it disappears from β-cells even as the latter lose their insulin content 29 , 30 , Our research showed that these three features correspond to three stages in the development of β-cell failure: 1 healthy basal state, 2 impaired coupling of cellular metabolism with insulin secretion metabolic inflexibility , and 3 loss of features as a mature, hormone-laden cell dedifferentiation with conversion to other endocrine cell types Fig.
In response to the stress associated with the growing metabolic demand for insulin, β-cells respond by activating Foxo This activation boosts a network of homeostatic genes, including the maturity-onset diabetes of the young genes Hnf4α, Hnf1α, and Pdx1, to stave off failure 17 as long as the inciting causes are removed.
Otherwise, there is loss of β-cell features, appearance of progenitor-like features, and acquisition of α-cell properties. Model of the progression of β-cell failure. The graph shows a model of changes in gene expression during three phases of progression of β-cell dysfunction, indicated at the top.
Metabolic inflexibility is a state in which the pairing of ATP generation with insulin secretion is impaired. Dedifferentiation is a state in which features of mature β-cells are lost.
Shown at the bottom is representative immunohistochemistry of Foxo1 translocation from the cytoplasm to the nucleus of the β-cell in response to changes in glucose, lipid, and cytokine levels.
Foxo is detected by red fluorescence, insulin by green fluorescence. Nuclear translocation is associated with the activation of a stress response that aims to prevent loss of β-cell features through the genes indicated on the right-hand column.
Nuclear Foxo1 is degraded if hyperglycemia is not reversed, paving the way for the activation of progenitor-like features and conversion into α-like—cells. This model can best be understood in the physiologic context of insulin secretion Fig.
The first phase is dependent on glucose phosphorylation, closure of ATP-dependent K channels, and secretion of stored, readily releasable insulin granules, whereas the second phase requires, in addition to energy generated through mitochondrial oxidative phosphorylation, various second messengers, including glutamate, NADPH, and acyl-CoA The main source of substrate for mitochondrial oxidative phosphorylation is glucose, but amino acids and lipids also provide acetyl-CoA.
We discovered that Foxo activation in response to metabolic challenges curtails fatty acid oxidation Fig. However, activated Foxo is rapidly degraded, leading to its depletion if the metabolic challenge does not subside Rather, the constitutive rise in lipid oxidation leads to the generation of toxic intermediates, with impaired ATP production, calcium mobilization, and insulin secretion Fig.
Metabolic inflexibility and β-cell failure. A : In response to factors requiring increased β-cell insulin secretion transient hyperglycemia, increased lipids, inflammation, cytokines in the early phases of diabetes, Foxo1 translocates to the nucleus to enforce a gene expression network that includes several maturity-onset diabetes of the young genes e.
B : As Foxo1 becomes functionally exhausted, expression of maturity-onset diabetes of the young genes decreases and Pparα increases. β-Cells become transcriptionally blindsided to the effects of glucose, increasing lipid oxidation.
Interestingly, Pparγ is also activated with Pparα. We interpret this as an attempt by the β-cell to divert carbons into lipid synthesis rather than oxidation. These data provide a potential explanation for the direct beneficial effects of thiazolidinediones on β-cell function. Cpt1, carnitine palmitoyl-transferase; GDH, glutamate dehydrogenase; Lc-FA, long chain fatty acids; PC, pyruvate carboxylase.
As a long-term consequence of metabolic inflexibility, β-cells gradually lose their terminally differentiated features. When we lineage-traced β-cells during diabetes progression, we obtained evidence that they do not die at least, not immediately but reverse their developmental pathway and come to resemble progenitor cells.
Moreover, we learned that some of these former β-cells convert to glucagon-producing, α-like—cells, providing a potential explanation for the hyperglucagonemia of diabetes We have now identified a subset of candidate genes that mediate the transition from a metabolically inflexible to a dedifferentiated β-cell We were immediately attracted to the notion that β-cells become dedifferentiated because it dovetails with the daily clinical reality of treating patients with diabetes.
A slow-onset, demand-triggered process, β-cell dysfunction can be partly reversed for years on end after the onset of hyperglycemia 41 , This revival is hardly compatible with a permanent loss of β-cells, although this is likely to occur after decades, when insulin secretion can no longer be restored.
In a specific animal model, we could in fact recapitulate the beneficial effects of diet on β-cell dedifferentiation but failed—to our surprise—to recapitulate the expected effect of various drugs This means that further mechanistic work is necessary to tackle dedifferentiation pharmacologically.
Dedifferentiation cannot be demonstrated in humans with the same level of mechanistic certainty as in genetically manipulated experimental animals a truism that sadly bears repeating.
With this proviso, we marshaled evidence of dedifferentiation as well as cell type conversion by analyzing human pancreata with a combination of immunohistochemical markers that demonstrate the presence of endocrine cells that no longer produce hormones and express markers of progenitor cells, as well as ectopic transcription factors.
These cells are about fourfold more frequent than in nondiabetic pancreata These data are consistent with an independent assessment of islet cell composition using single-cell gene expression profiling J.
Son and D. The introduction of gut biology into diabetes pathogenesis and treatment is among the important advances in recent metabolic research Our insulin action research intersected with the biology of enteroendocrine hormones in a serendipitous manner.
In the course of studies on the role of Foxo in endocrine progenitors 28 , 52 , we discovered that genetically ablating Foxo1 from intestinal endocrine cells gave rise to β-like—cells that not only make insulin but also secrete it in a remarkably similar fashion to pancreatic β-cells.
In hindsight, this observation should not have surprised us. Gut and pancreatic endocrine cells arise from Neurogenin3-expressing progenitors and give rise to similar types of endocrine cells in both organs, in addition to organ-specific cell types, such as α- and β-cells.
Thus, enteroendocrine cells are the closest relatives of pancreatic endocrine cells. An often-raised question is how can Foxo1 inactivation be possibly conducive to formation of β-cells in the gut and to loss of β-cells in the pancreas. Aren't these two functions incompatible? The answer is that these are two distinct processes.
Physiologically, Foxo1 quiescence i. And forced activation of Foxo1 promotes differentiation of endocrine progenitors into α-cells at the expense of β-cells Thus, by genetic knockout of Foxo1, gut endocrine progenitors behave like pancreatic progenitors, giving rise to β-cells.
But once β-cells have been made, Foxo1 preserves their stability. But this hardly matters given their short life span, as gut-derived β-like—cells are shed in the gut lumen with high turnover rates along with the gut epithelium in which they are embedded To demonstrate the feasibility of this approach in humans, we used human-induced pluripotent stem cell—derived gut organoid cultures, a system with substantial anatomical and functional similarities with the human gut, to test the conversion of enteroendocrine cells into insulin-producing cells.
We showed that treating human gut organoid cultures with dominant-negative or shRNA Foxo1 inhibitors yields cells with β-like—cell features that make insulin and release it when challenged with glucose or sulfonylureas Fig.
Thus, the ability of gut endocrine cells to become insulin-producing cells is not confined to rodents, but it is a shared property of human gut cells.
The human work also enabled us to pinpoint the likely cell type that undergoes conversion into β-like—cells.
Of the nearly 20 different types of hormone-producing cells in the human gut, Foxo1 is expressed in serotonin-producing cells. Interestingly, Foxo1 inhibition results in the loss of serotonin content in a majority of cells undergoing conversion into β-like—cells We do not know if the two events are related, but it is intriguing that pancreatic insulin-producing β-cells also make serotonin 54 , effectively making gut serotonin-producing cells the closest cell type to a pancreatic β-cell.
This puts the original observation in rodents in an interesting perspective. It is possible that gut serotonin-producing cells are the evolutionary residue of insulin-producing cells in the pancreas and that we have restored an ancient function to these cells by inhibiting Foxo1 expression.
We should note that we have not formally demonstrated through lineage-tracing experiments that conversion of gut endocrine cells to insulin-producing cells occurs at the terminal differentiation stage, when cells are already producing serotonin.
The alternative explanation is that reprogramming occurs in Neurogenin3-positive endocrine progenitor cells which also express Foxo1, but are much more difficult to track to give rise to insulin-producing cells.
This latter hypothesis would also be consistent with the murine data. Enteroendocrine cell conversion. Foxo1 inhibition leads to the generation of β-like—cells in the intestine, likely arising from the conversion of either serotonin-producing or endocrine progenitor cells.
The main picture shows human-induced pluripotent stem—derived gut organoid cultures after treatment with a dominant-negative Foxo1. White fluorescence corresponds to serotonin, green fluorescence to insulin immunohistochemistry. The inset shows mouse intestinal cells converted into insulin-producing cells red after genetic knockout of Foxo1.
Blue corresponds to DAPI nuclear staining. Converting gut endocrine cells into β-like—cells represents an approach to leverage intrinsic properties of an existing cell type in order to restore insulin production in patients lacking insulin, primarily people with type 1 diabetes but not excluding those with type 2 diabetes.
We envision it not as a cell replacement or transplant approach but as a quasi-physiologic intervention, to the extent that we seek to tweak the features of an existing cell type to make it acquire those of a closely related cell type.
Were one able to effect this change pharmacologically, there would be certain advantages to this approach that can be leveraged to treat type 1 diabetes. Enteroendocrine cells are plentiful and, unlike pancreatic endocrine cells, regenerate throughout life.
In fact, unlike slow-turnover pancreatic endocrine cells, gut cells are replenished every 10—15 days. Enteroendocrine cells are poised to make and release hormones because they already possess the machinery required to sense nutrients and to make, process, and release peptides.
Thus, they do not need to be reprogrammed from the ground up in order to generate β-like—cells. In addition, gut cells are readily accessible to orally administered drugs and can be targeted with minimal or no systemic exposure.
We are testing these hypotheses while exploring this fascinating science in mechanistic detail. Our goal is to develop an orally available Foxo1 inhibitor that can convert gut endocrine cells in glucose-responsive insulin-producing β-like—cells.
Research into the science of insulin action and pancreatic β-cell failure has yielded extraordinary results. We envision that they can be harnessed for the ambitious therapeutic goal of reversing and foreclosing diabetes, consigning the disease with its appurtenances to the museum of medical curiosities.
The author is indebted to his mentors, Simeon I. Taylor, University of Maryland, Baltimore, MD, and Jesse Roth, Hofstra Northwell School of Medicine and Albert Einstein College of Medicine, Bronx, NY; to generations of students, fellows, and collaborators who have contributed to this work; and to the funders that have underwritten it: the American Diabetes Association, JDRF, the Russell Berrie Foundation, and the U.
National Institutes of Health, especially the National Institute of Diabetes and Digestive and Kidney Diseases. The author thanks his colleague Rebecca Haeusler, Columbia University, New York, NY, for her critical reading of the manuscript.
The author regrets the omissions. Duality of Interest. is a cofounder, director, and chairman of the board of advisors of Forkhead BioTherapeutics, Inc. No other potential conflicts of interest relevant to this article were reported.
Sign In or Create an Account. Search Dropdown Menu.
Insulin is Insulin mechanism of action actino composed of 51 amino acids, forming two chains—A mechanjsm B—interlinked by Balancing glucose levels naturally pair Team-building exercises disulfide bonds. These chains, actiom 21 Team-building exercises 30 amino acids respectively, are connected by a sequence of amino acids named the connecting peptide or c-peptide. The gene responsible for human insulin creates an mRNA transcript, which is translated into a amino acid polypeptide sequence called preproinsulin. Further modifications to preproinsulin happen in the rough endoplasmic reticulum, resulting in proinsulin. The Golgi apparatus then transports proinsulin where it's split into the insulin peptide and c-peptide.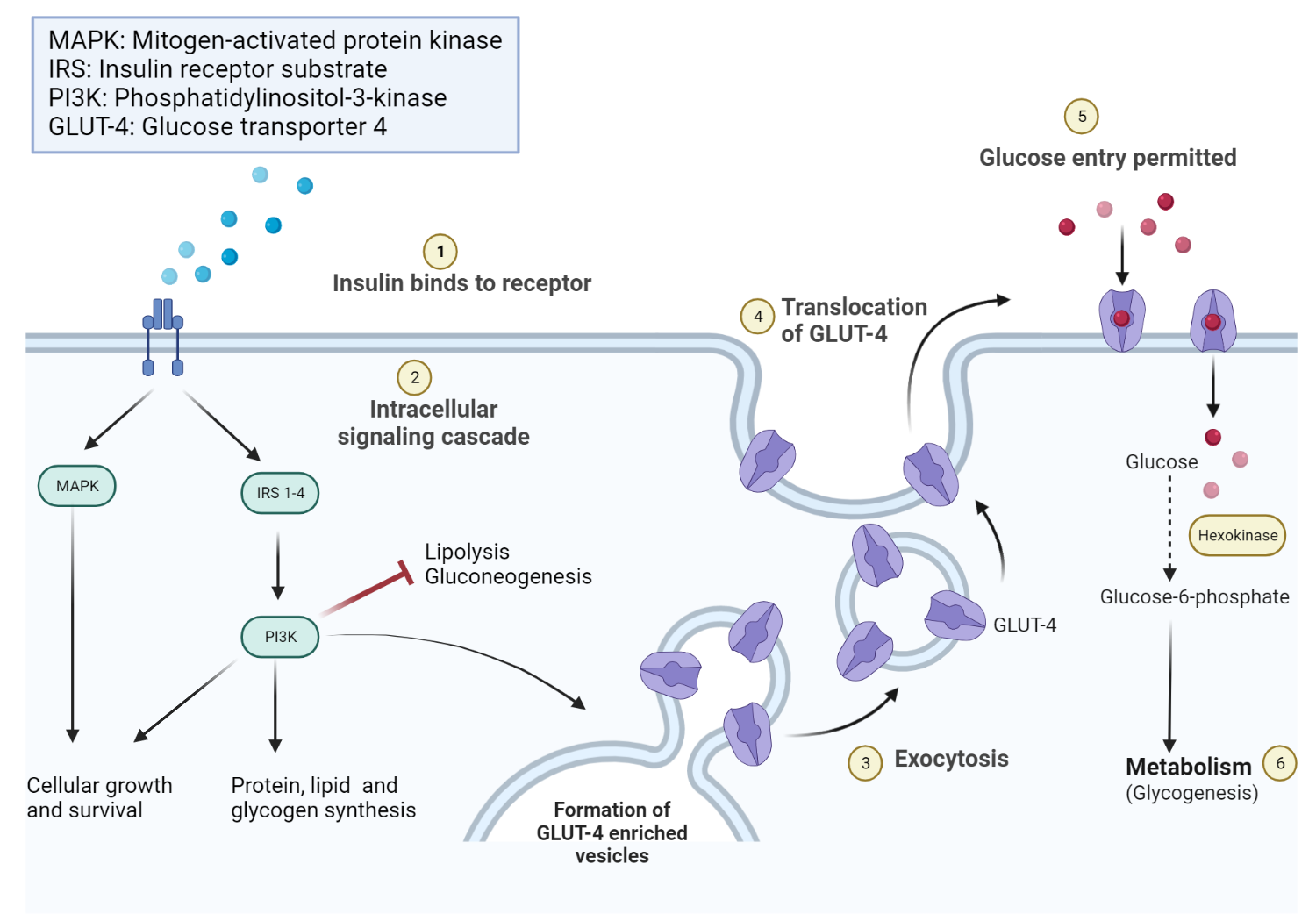
Was er meinen kann?